This is the second EDIS publication in the cellular agriculture series that provides information to seafood industry producers and consumers on production strategies for developing novel cell-based seafood products.
Introduction
Cellular agriculture, which is using the advances in muscle tissue engineering for food production, has been proposed as a complementary method to conventional seafood production systems (i.e., aquaculture and wild-capture fisheries) to ensure sustainable seafood production for feeding the growing global population. In the first of this series, publication #FSHN21-2, “Cellular Agriculture for Production of Cell-Based Seafood," we discussed background information on cell-based seafood manufacturing techniques, the challenges of the industry, and why cell-based seafood production has several manufacturing advantages over the production of other cell-based animal proteins, such as beef and poultry. This EDIS publication provides information on why some fish species are better candidates for cell-based seafood production. In addition, we discuss why zebrafish could be a suitable animal model for the research and development of cell-based seafood.
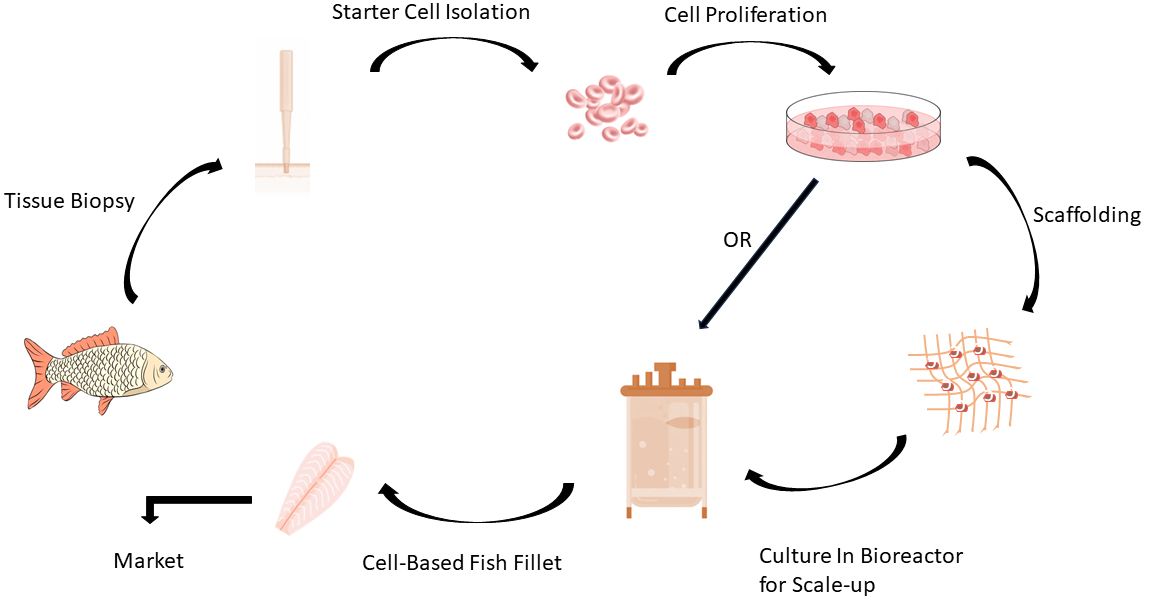
Credit: Rose Omidvar and Razieh Farzad, UF/IFAS
Why should lean fish be the primary focus for cell-based seafood production?
The key element of a fillet's complexity relates to the presence of fat cells within its primary muscle tissue matrix, as there are barriers related to different growth patterns of muscle and fat on the same substrate (Sun et al. 2017). Lipid content in the fish muscle varies somewhere between 0.5% to 20%, depending on the type of fish. According to their muscle fat content, fish can be categorized as ‘‘fatty’’ or ‘‘lean’’ (Alam 2007). Lean fish, such as cod, haddock, hake, and zebrafish, have a flesh lipid content of 0.1% to 1%. In such cases, fat is stored mostly in the liver. Fatty fish, such as tuna, mackerel, herring, salmon, anchovies, and capelin, store the lipid in the flesh (i.e., fillets); have muscle-fat content within the range of 10% to 20%; and have a relatively small liver (Kling et al. 2012). Since the fat amount is high in fatty fish, fat cells accumulate appearing as globular (Caballero, Trugo, and Finglas 2003). These fat cells are intimately associated with muscle protein, thus adding complexity to the process of cell-based meat production. On the other hand, the fat content in the lean fish is spread in thin layers, which minimizes manufacturing complexity; hence it is considered a better candidate for this development. The minimal fat content in the fillets of lean fish and a high level of fat in the liver indicate that intermuscular fat probably does not play a key biological role in lean fish muscle growth (Potter et al. 2020). Using this assumption, a process can be designed that does not require the co-culturing of the fat and muscle cells. Therefore, focusing on lean fish for cell-based seafood development has been suggested to be a potentially more tenable first manufacturing solution for the cell-based seafood industry.
Fat content and the fatty acid profile of fish fillets affect consumers. The flavor profile of the cell-based seafood product can be simplified using lean fish tissue as the starting material. Due to the small amount of fat in lean fish muscle tissue, there is less variation in flavor across the species compared to fatty fishes like salmon, which has a more complex flavor profile. Due to the similar sensory qualities of lean fish across species, there is a possibility for many species to be used interchangeably in the mass market, recreating a fully recognized sensory experience as any other lean fish (Prell and Sawyer 1988). Additionally, lipid oxidation results in the production of undesirable flavors and oxidized products; it induces protein oxidation, which contributes to deterioration in the texture and sensory characteristics of the fish fillet. By producing a lean fish, the lipid oxidation will be delayed, leading to longer shelf life (Kunyaboon et al. 2021). Given that, the manufacturing steps of a single successfully developed cell-based lean fish species can be used to develop other cell-based lean fish species. This is also critical for the sustainable production of cell-based seafood since focusing on single species development would be less resource intensive, reducing the time and cost of production.
Can zebrafish be used as a research and production model?
Most of the research in cell culture in the past decades has been focused on mammalian cell cultures; in vitro models have been extremely useful for expanding knowledge about molecular mechanisms of muscle growth in mammalians (Goswami et al. 2022). However, there is still an extensive knowledge gap on growing fish tissue in vitro. One main challenge is the lack of a proper cell source. Despite many different available seafood species in the ocean, only a few seafood cell lines are available (Zhang et al. 2020). Even though there are multiple private startups currently working on cell line development of various kinds of seafood such as salmon, bluefin tuna, yellowtail, and shrimp products (Rubio et al. 2019), we have to base our work on what is known in the public domain, and zebrafish by far has been the most studied fish on a cellular level in the literature. Thereby we contend zebrafish is a great candidate for research related to cell-based seafood production due to the following attributes.
Zebrafish (Danio rerio) is a small-bodied, freshwater fish species native to South Asia. For several decades, zebrafish has been used as a major model organism for biomedical research and comparative and evolutionary biology due to the many technical advantages it offers (Arunachalam et al. 2013). Compared to other vertebrate models, zebrafish are easy and inexpensive to maintain, possess excellent reproductive capabilities, have a short life cycle, and are readily available (Tavares and Lopes 2013). The Zebrafish Information Network (ZFIN) has established an online database where the integrated genetic and genomic data for the zebrafish are collected (ZFIN n.d.).
Researchers investigating cell-based meat procedures can use these available protocols, related to zebrafish on a cellular level, as a benchmark and expand it to other lean fish categories. For example, there are experimental protocols available for isolating muscle stem cells or embryonic stem cells of zebrafish (Xiao et al. 2016). Another advantage of using zebrafish compared to wild-catch or aquaculture-raised species is the possibility of raising them in the lab since supplies of embryos are readily available (Kinth, Mahesh, and Panwar 2013). As mentioned earlier, zebrafish have high fecundity and create offspring within a short generation time. Aside from that, there are specific pathogen-free (SPF) zebrafish research facilities where colonies of zebrafish starter cells can be obtained (Kent et al. 2011). This would reduce preparation steps and shorten research time while ensuring the safety and wellness of the cells. All these findings together make zebrafish a feasible choice for research and development of cell-based lean fish meat production.
Conclusion
Comparing the lean and fatty fish muscle properties suggests that cell-based lean fish fillet production can be less complex and resource-intensive due to reducing the process steps and decreasing the production timeline. There are decades of research using zebrafish as a model for human disease, that have generated a wealth of information on fish muscle development and growth. Therefore, we suggest using all of this information on zebrafish, a lean fish, for developing the protocols for cell-based seafood. The information can be used to understand how fish muscle develops and grows, to define optimal culture conditions, and to develop and maintain cell lines from other lean fish species.
References
Alam, A. N. 2007. Structure of Fish Muscles and Composition of Fish.
Arunachalam, M., M. Raja, C. Vijayakumar, P. Malaiammal, and R. L. Mayden. 2013. “Natural History of Zebrafish (Danio rerio) in India.” Zebrafish 10 (1): 1–14. https://doi.org/10.1089/zeb.2012.0803
Berger, J., and P. D. Currie. 2012. “Zebrafish models flex their muscles to shed light on muscular dystrophies.” Disease Models & Mechanisms 5 (6): 726–732. https://doi.org/10.1242/dmm.010082
Caballero, B., L.C. Trugo, and P.M. Finglas. 2003. Encyclopedia of Food Sciences and Nutrition. Academic.
Cook, Z. T., N. L. Brockway, Z. J. Tobias, J. Pajarla, I. S. Boardman, H. Ippolito, S. Nkombo Nkoula, and T. A. Weissman. 2019. “Combining Near-Infrared Fluorescence with Brainbow to Visualize Expression of Specific Genes within a Multicolor Context.” Molecular Biology of the Cell 30 (4): 427–524. https://doi.org/10.1091/mbc.E18-06-0340
Goswami, M., Y. Belathur Shambhugowda, A. Sathiyanarayanan, N. Pinto, A. Duscher, R. Ovissipour, W. Singh Lakra, and R. Chandragiri Nagarajarao. 2022. “Cellular Aquaculture: Prospects and Challenges.” Micromachines 13 (6): 828. https://doi.org/10.3390/mi13060828
Erkkilä, A., V. D. de Mello, U. Risérus, and D. E. Laaksonen. 2008. “Dietary Fatty Acids and Cardiovascular Disease: An Epidemiological Approach.” Progress in Lipid Research 47 (3): 172–187. https://doi.org/10.1016/j.plipres.2008.01.004
Kent, M. L., C. Buchner, V. G. Watral, J. L. Sanders, J. LaDu, T. S. Peterson, and R. L. Tanguay. 2011. “Development and Maintenance of a Specific Pathogen-free (SPF) Zebrafish Research Facility for Pseudoloma neurophilia.” Diseases of Aquatic Organisms 95 (1): 73–79. https://doi.org/10.3354/dao02333
Khan, K. M., A. D. Collier, D. A. Meshalkina, E. V. Kysil, S. L. Khatsko, T. Kolesnikova, Y. Y. Morzherin, J. E. Warnick, A.V. Kalueff, and D. J. Echevarria. 2017. “Zebrafish Models in Neuropsychopharmacology and CNS Drug Discovery.” British Journal of Pharmacology 174 (13): 1925–1944. https://doi.org/10.1111/bph.13754
Kinth, P., G. Mahesh, and Y. Panwar. 2013. “Mapping of Zebrafish Research: A Global Outlook.” Zebrafish 10 (4): 510–517. https://doi.org/10.1089/zeb.2012.0854
Kling, P., E. Jönsson, T. O. Nilsen, I. E. Einarsdottir, I. Rønnestad, S. O. Stefansson, and B. T. Björnsson. 2012. “The Role of Growth Hormone in Growth, Lipid Homeostasis, Energy Utilization, and Partitioning in Rainbox Trout: Interactions with Leptin, Ghrelin and Insulin-like Growth Factor I.” General and Comparative Endocrinology 175 (1): 153–162. https://doi.org/10.1016/j.ygcen.2011.10.014
Kunyaboon, S., K. Thumanu, J. W. Park, C. Khongla, and J. Yongsawatdigul. 2021. “Evaluation of Lipid Oxidation, Volatile Compounds and Vibrational Spectroscopy of Silver Carp (Hypophthalmichthys molitrix) during Ice Storage as Related to the Quality of Its Washed Mince.” Foods 10 (3): 495. https://doi.org/10.3390/foods10030495
Ouellet, V., J. Marois, S. J. Weisnagel, and H. Jacques. 2007. “Dietary cod protein improves insulin sensitivity in insulin-resistant men and women: A randomized controlled trial.” Diabetes Care 30 (11): 2816–2821. https://doi.org/10.2337/dc07-0273
Potter, G., A. S. T. Smith, N. T. K. Vo, J. Muster, W. Weston, A. Bertero, L. Maves, D. L. Mack, and A. Rostain. 2020. “A more open approach is needed to develop cell-based fish technology: It starts with Zebrafish.” One Earth 3 (1): 54–64. https://doi.org/10.1016/j.oneear.2020.06.005
Prell, P. A., and F. M. Sawyer. 1988. “Flavor Profiles of 17 Species of North Atlantic Fish.” Journal of Food Science 53 (4): 1036–1042. https://doi.org/10.1111/j.1365-2621.1988.tb13524.x
Rubio, N., I. Datar, D. Stachura, D. Kaplan, and K. Krueger. 2019. “Cell-Based Fish: A Novel Approach to Seafood Production and an Opportunity for Cellular Agriculture.” Frontiers in Sustainable Food Systems 3: 43. https://doi.org/10.3389/fsufs.2019.00043
Sun, W., T. He, C. Qin, K. Qiu, X. Zhang, Y. Luo, D. Li., and J. Yin. 2017. “A Potential Regulatory Network Underlying Distinct Fate Commitment of Myogenic and Adipogenic Cells in Skeletal Muscle.” Science Reports 7: 44133. https://doi.org/10.1038/srep44133
Tavares, B., and S. S. Lopes. 2013. “The Importance of Zebrafish in Biomedical Research. Acta Médica Portuguesa 26 (5): 583–592. https://doi.org/10.20344/amp.4628
Xiao, Y., M. Gao, L. Gao, Y. Zhao, Q. Hong, Z. Li, J. Yao, H. Cheng, and R. Zhou. 2016. “Directed Differentiations of Zebrafish Pluripotent Embryonic Cells to Functional Cardiomyocytes.” Stem Cell Reports 7 (3): 370–382. https://doi.org/10.1016/j.stemcr.2016.07.020
Zebrafish Information Network (ZFIN). n.d. Search page. Retrieved on September 5, 2022. https://zfin.org/.
Zhang, G., X. Zhao, X. Li, G. Du, J. Zhou, and J. Chen. 2020. “Challenges and Possibilities for Bio-manufacturing Cultured Meat.” Trends in Food Science & Technology 97: 443–450. https://doi.org/10.1016/j.tifs.2020.01.026