This series of EDIS publications provides information about different agricultural management options available to improve resource-use efficiency and adapt to climate variability and change. To see the complete series of publications, visit https://edis.ifas.ufl.edu/entity/topic/series_agricultural_management_options.
Introduction
Adapting to climate variability and change can be achieved through a broad range of management alternatives and technological advances. While decision making in agriculture involves many aspects beyond climate, including economics, social factors, and policy considerations, climate-related risks are a primary source of yield and income variability. Existing strategies can help producers minimize the risks associated with climate variability and change as well as improve resource use efficiency. This series of EDIS publications gives information on these existing technologies, and this publication focuses on the use of microirrigation to improve production systems.
What is microirrigation?
Microirrigation is the slow, frequent application of water directly to relatively small areas adjacent to individual plants through emitters placed along a water delivery line. Water is generally conveyed in low-pressure, flexible plastic tubing. Generally, water must be of high quality to avoid clogging the small emitters; this is often managed with filtration and occasional chemical treatments. A leading advantage of microirrigation is that non-beneficial evaporation—meaning evaporation of water from soil surfaces and plant canopies that does not contribute to plant growth—is greatly reduced when compared to sprinkler irrigation. Microirrigation is a broad term and includes several application methods.
- Drip irrigation: Water is applied through small emitters to the soil surface, usually at or near the plant to be irrigated (Figures 1 and 2). Emitter low rates are usually less than 3 gallons per hour, and application rates (inches/day) depend on emitter and lateral spacing.
- Subsurface drip irrigation (SDI): Water is applied below the soil surface through drip line laterals that are installed at a depth of 12–18 inches (Figure 3). Tillage, planting, and other field operations are not impeded by laterals because they are established at a sufficient depth to allow for field operations and long-term use. Emitter flow rates for SDI are generally less than 3 gallons per hour. SDI can have a useable lifetime of up to 20 years, making it the most economically competitive with center pivot irrigation of low-value commodity row crops (Lamm et al. 2010).
- Microspray irrigation: Water is applied to the soil surface in a small spray or mist by an emitter 6–12 inches above the soil surface. Application rates are usually less than 40 gallons per hour. Microspray can provide the additional service of freeze damage protection for some horticultural crops.
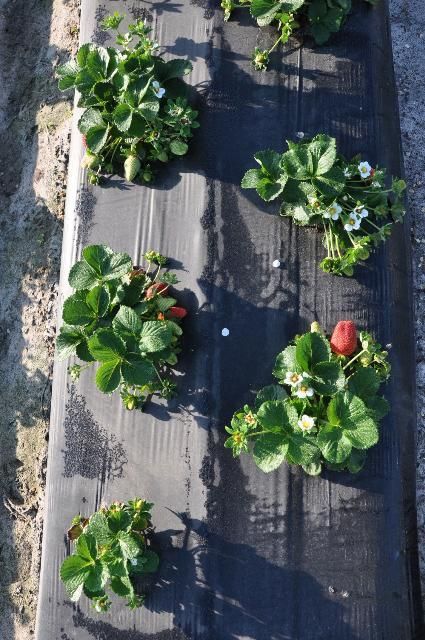
Credit: Lincoln Zotarelli, UF/IFAS
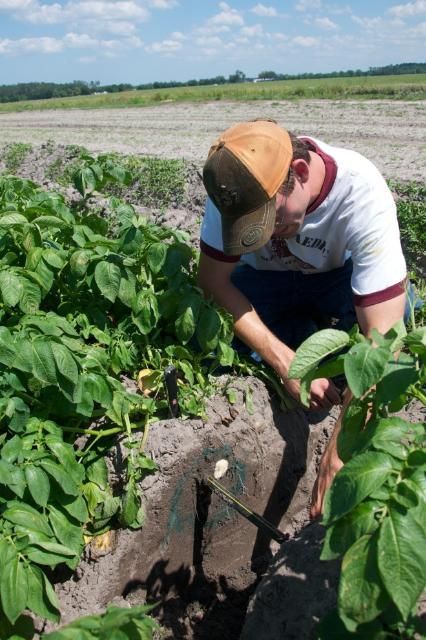
Credit: Lincoln Zotarelli, UF/IFAS
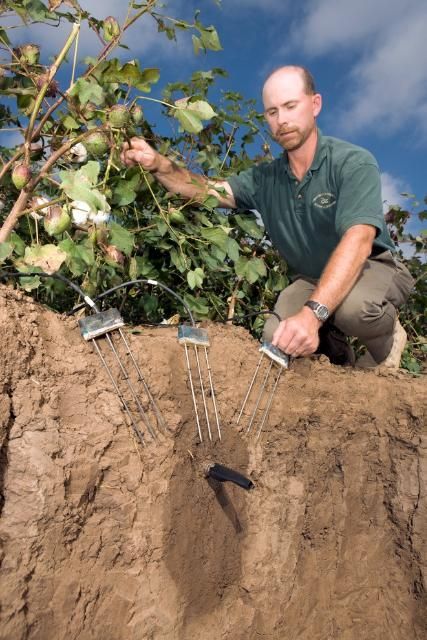
Credit: Peggy Greb
How does microirrigation reduce climate-related risks?
Any type of irrigation in a commercial agriculture system can greatly reduce the climate-related yield risks resulting from water stress (Harwood et al. 1999). Because of improved efficiencies and reduced pest pressure, microirrigation can provide some added measure of reduced risk compared to overhead irrigation systems. This can be summarized as follows:
- Because of its high efficiency (less non-beneficial soil-water evaporation, wind drift, evaporation of canopy-intercepted water), microirrigation reduces the irrigation water volume required to grow crops, which can lower the risk of water supply shortages for irrigation.
- Microirrigation allows for flexibility in the timing and amounts of applied water according to the evapotranspiration/plant demand.
- Because less water is applied, nutrient leaching is reduced.
- Nutrient applications can also be better timed to meet plant needs. Application of fertilizers in irrigation water means the nutrients can be delivered directly to the root zone.
- Microirrigation allows for the use of polyethylene mulch, which helps soil-water conservation and reduces fertilizer leaching from rainfall.
- Microirrigation can be used to protect small horticultural crops from freezes.
What are the agronomic benefits?
The reduced wetting of soil surfaces and plant canopies may result in lower weed and disease pressure. Yield improvements in some low-value row crops have been demonstrated for SDI when compared to center pivot. See the example for cotton in Figure 4. Also, lower water footprints (the ratio of crop water use to yield) have been observed for SDI even when compared to highly efficient application technologies under center pivots (Figure 5). The following list is a summary of the leading agronomic benefits of microirrigation:
- Reduced water use: Because drip irrigation brings the water to the plant root zone and does not wet the entire field, drip irrigation typically requires 25%–50% of the volume of water needed by comparable overhead irrigation systems (Lamm and Trooien 2003).
- Reduced pest problems: Weed and disease problems may be reduced because drip irrigation does not wet the row middles or the foliage of the crops like overhead irrigation.
- Reduced surface crusting: Microirrigation can reduce the crusting of soil surfaces that may result from repeated sprinkler applications.
- Joint management of irrigation and fertilization: Drip irrigation can improve the efficiency of both water and fertilizer applications. Precise nutrient application is possible using drip irrigation, potentially reducing fertilizer costs and soluble nutrient losses.
- Production advantages: In horticultural systems, when combined with raised beds, polyethylene mulch, and transplants, drip irrigation enhances earliness and crop uniformity. Using polyethylene mulch also increases the cleanliness of harvested products and reduces the risk of contamination with soilborne pathogens. Reflective mulches further help reduce the incidence of viral diseases by affecting insect vectors, such as thrips, whiteflies, or aphids.
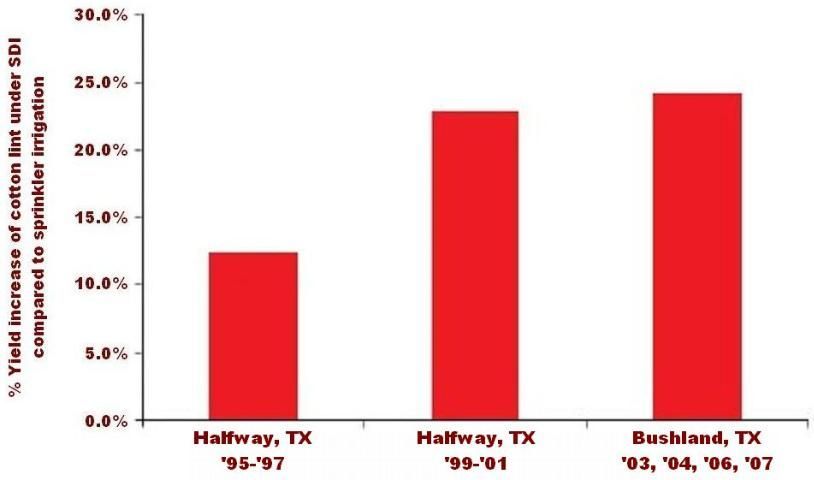
Credit: Daniel Dourte
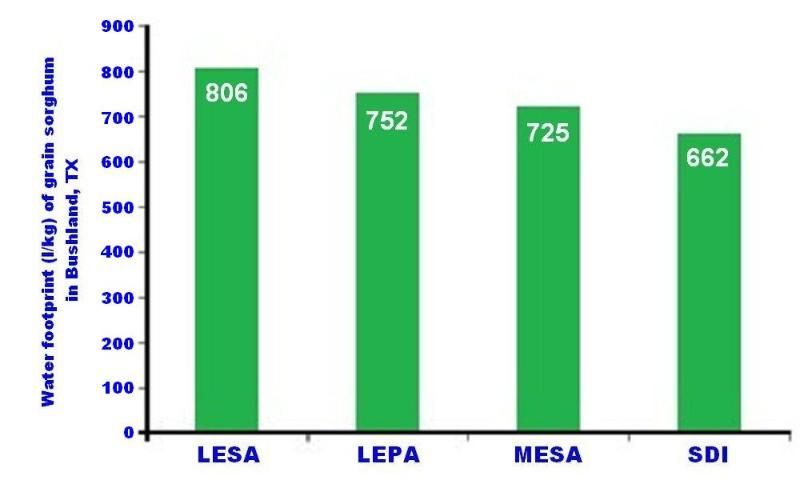
Credit: Daniel Dourte
What are the impacts on production costs?
- Low pumping needs: Drip systems require low operating pressure (20–25 psi at field entrance, 10–12 psi at the drip tape) compared to overhead systems (50–80 psi). Many existing small pumps and wells may be used to adequately irrigate small acreage using drip systems.
- Automation: Drip irrigation application may be simply managed and programmed using irrigation controllers, thereby reducing labor cost.
- Flexibility: Drip systems are adaptable to oddly shaped fields or those with uneven topography or soil texture, thereby eliminating the underutilized or non-cropped corners and maximizing the use of available land.
What is the investment cost?
Drip irrigation systems typically cost around $500–$1,500 per acre. Part of the cost is a capital investment useful for several years, and another part is because of the annual cost of disposable parts. Growers new to drip irrigation should start with a relatively simple system on a small acreage before moving to a larger system. SDI for commodity row crops has been found to be less expensive than center pivot irrigation systems on a 160-acre corn field for an SDI lifetime greater than 15 years (Lamm et al. 2012).
What are the impacts on greenhouse gas emissions?
SDI has been shown to result in substantial decreases in N2O emissions when compared with emissions under furrow irrigation (Sanchez-Martin et al. 2008). The fuel costs of SDI compared to center pivot irrigation can be $5–$15 per acre lower for corn irrigation (O'Brien et al. 1997; Lamm et al. 2012), suggesting reductions in energy-related CO2 emissions can be observed with SDI.
What are the barriers and incentives for implementation?
Barriers
- Economic investment
- Lack of information about the system, correct management, and maintenance
- High-quality water
Incentives
- Cost-Share Programs for Water Conservation – qualified growers are expected to contribute a portion of total project cost
- Water Conservation Programs administered by USDA (e.g., Environmental Quality Incentives Program [EQIP])
- State and Regional Water Conservation Programs (e.g., BMP Cost-Share Program [FDASC]; FARMS Program [SWFWMD]; Water Protection and Sustainability Cost-Share Program [SJRWMD])
Acknowledgments
This information was developed in contribution to the project, "Climate Variability to Climate Change: Extension Challenges and Opportunities in the Southeast USA," and was supported by Agriculture and Food Research Initiative competitive grant no. 2011-67003-30347 from the USDA National Institute of Food and Agriculture.
References
Colaizzi, P. D., A. D. Schneider, S. R. Evett, and T. A. Howell. 2004. "Comparison of SDI, LEPA, and Spray Irrigation Performance for Grain Sorghum." Trans. ASAE 47 (5): 1477–1492.
Harwood, J., R. G. Heifner, K. Coble, P. Janet, and A. Somwaru. 1999. Managing Risk in Farming: Concepts, Research and Analysis. Washington, DC: Economic Research Service, USDA.
Lamm, F. R., P. D. Colaizzi, J. P. Bordovsky, T. P. Trooien, J. Enciso-Medina, D. O. Porter, D. H. Rogers, and D. M. O'Brien. 2010. "Can Subsurface Drip Irrigation (SDI) Be a Competitive Irrigation System in the Great Plains Region for Commodity Crops?" 2010. Proceedings of the 5th National Decennial Irrigation meeting of the ASABE, Phoenix Convention Center, 5–8 December 2010, Phoenix, AZ USA.
Lamm, F. R., D. M. O'Brien, D. H. Rogers, and T. J. Dumler. 2012. "Using the K-State Center Pivot Sprinkler and SDI Economic Comparison Spreadsheet." Proceedings of the 24th Annual Central Plains Irrigation Conference, Colby, Kansas, February 21–22. https://www.ksre.k-state.edu/sdi/reports/2012/LammUsingCPSDI12.pdf. Accessed January 26, 2023.
Lamm, F. R., and T. P. Trooien. 2003. "Subsurface Drip Irrigation for Corn Productivity: A Review of 10 Years of Research in Kansas." Irrig. Sci. 22 (3–4): 195–200.
O'Brien, D. M., D. H. Rogers, F. R. Lamm, and G. Clark. 1997. "Economic Comparison of SDI and Center Pivots for Various Field Sizes." Kansas State University. https://www.ksre.k-state.edu/historicpublications/pubs/MF2242.pdf. Accessed January 26, 2023.
Sanchez-Martin, L., A. Arce, A. Benito, L. Garcia-Torres, and A. Vallejo. 2008. "Influence of Drip and Furrow Irrigation Systems on Nitrogen Oxide Emissions from a Horticultural Crop." Soil Biology & Biochemistry 40 (7): 1698–1706.
Additional information
Clark, G. A., and D. Z. Haman. 2011. Microirrigation in Mulched Bed Production Systems: Irrigation Depths. AE72. Gainesville: University of Florida Institute of Food and Agricultural Sciences. https://edis.ifas.ufl.edu/publication/ae049
Florida Agricultural Water Conservation Best Management Practices. [2012.] https://www.fdacs.gov/Agriculture-Industry/Water/Agricultural-Best-Management-Practices
Haman, D. Z. 2011. Scheduling Tips for Drip Irrigation of Vegetables. AE259. Gainesville: University of Florida Institute of Food and Agricultural Sciences. https://edis.ifas.ufl.edu/publication/ae092
Haman, D. Z., and F. T. Izuno. 2003. Principles of Microirrigation. AE70. Gainesville: University of Florida Institute of Food and Agricultural Sciences. https://edis.ifas.ufl.edu/publication/wi007
Bayabil, H.K., K. W. Migliaccio, M.D. Dukes, and L. Vasquez. 2023. Basic Tips for Designing Efficient Irrigation Systems. AE539. Gainesville: University of Florida Institute of Food and Agricultural Sciences. https://edis.ifas.ufl.edu/publication/AE539
Simonne, E., R. Hochmuth, J. Breman, W. Lamont, D. Treadwell, and A. Gazula. 2012. Drip-Irrigation Systems for Small Conventional Vegetable Farms and Organic Vegetable Farms. HS1144. Gainesville: University of Florida Institute of Food and Agricultural Sciences. https://edis.ifas.ufl.edu/publication/hs388