Plant nutrients are one of the environmental factors essential for crop growth and development. Nutrient management is crucial for optimal productivity in commercial crop production. Those nutrients in concentrations of = 100 parts per million (ppm) in plant tissues are described as micronutrients and include iron (Fe), zinc (Zn), manganese (Mn), copper (Cu), boron (B), chlorine (Cl), molybdenum (Mo), and nickel (Ni). Micronutrients such as Fe, Mn, Zn, and Cu are easily oxidized or precipitated in soil, and their utilization is, therefore, not efficient. Chelated fertilizers have been developed to increase micronutrient utilization efficiency. This publication provides an overview of chelated fertilizers and considerations for their use to county Extension faculty, certified crop advisers (CCAs), crop consultants, growers, and students who are interested in commercial crop production.
What is chelated fertilizer?
The word chelate is derived from the Greek word chelé, which refers to a lobster's claw. Hence, chelate refers to the pincer-like way in which a metal nutrient ion is encircled by the larger organic molecule (the claw), usually called a ligand or chelator. Table 1 lists common natural or chemical synthetic ligands (Havlin et al. 2005; Sekhon 2003). Each of the listed ligands, when combined with a micronutrient, can form a chelated fertilizer. Chelated micronutrients are protected from oxidation, precipitation, and immobilization in certain conditions because the organic molecule (the ligand) can combine and form a ring encircling the micronutrient. The pincer-like way the micronutrient is bonded to the ligand changes the micronutrient's surface property and favors the uptake efficiency of foliarly applied micronutrients.
Why is chelated fertilizer needed?
Because soil is heterogeneous and complex, traditional micronutrients are readily oxidized or precipitated. Chelation keeps a micronutrient from undesirable reactions in solution and soil. The chelated fertilizer improves the bioavailability of micronutrients such as Fe, Cu, Mn, and Zn, and in turn contributes to the productivity and profitability of commercial crop production. Chelated fertilizers have a greater potential to increase commercial yield than regular micronutrients if the crop is grown in low-micronutrient stress or soils with a pH greater than 6.5. To grow a good crop, crop nutrient requirements (CNRs), including micronutrients, must be satisfied first from the soil. If the soil cannot meet the CNR, chelated sources need to be used. This approach benefits the plant without increasing the risk of eutrophication.
Several factors reduce the bioavailability of Fe, including high soil pH, high bicarbonate content, plant species (grass species are usually more efficient than other species because they can excrete effective ligands), and abiotic stresses. Plants typically utilize iron as ferrous iron (Fe2+). Ferrous iron can be readily oxidized to the plant-unavailable ferric form (Fe3+) when soil pH is greater than 5.3 (Morgan and Lahav 2007). Iron deficiency often occurs if soil pH is greater than 7.4. Chelated iron can prevent this conversion from Fe2+ to Fe3+.
Applying nutrients such as Fe, Mn, Zn, and Cu directly to the soil is inefficient because in soil solution they are present as positively charged metal ions and will readily react with oxygen and/or negatively charged hydroxide ions (OH-). If they react with oxygen or hydroxide ions, they form new compounds that are not bioavailable to plants. Both oxygen and hydroxide ions are abundant in soil and soilless growth media. The ligand can protect the micronutrient from oxidization or precipitation. Figure 1 shows examples of the typical iron deficiency symptoms of lychee grown in Homestead, Florida, in which the lychee trees have yellow leaves and small, abnormal fruits. Applying chelated fertilizers is an easy and practical correction method to avoid this nutrient disorder. For example, the oxidized form of iron is ferric (Fe3+), which is not bioavailable to plants and usually forms brown ferric hydroxide precipitation (Fe(OH)3). Ferrous sulfate, which is not a chelated fertilizer, is often used as the iron source. Its solution should be green. If the solution turns brown, the bioavailable form of iron has been oxidized and Fe is therefore unavailable to plants.
In the soil, plant roots can release exudates that contain natural chelates. The nonprotein amino acid, mugineic acid, is one such natural chelate called phytosiderophore (phyto: plant; siderophore: iron carrier) produced by graminaceous (grassy) plants grown in low-iron stress conditions. The exuded chelate works as a vehicle, helping plants absorb nutrients in the root-solution-soil system (Lindsay 1974). A plant-excreted chelate forms a metal complex (i.e., a coordination compound) with a micronutrient ion in soil solution and approaches a root hair. In turn, the chelated micronutrient near the root hair releases the nutrient to the root hair. The chelate is then free and becomes ready to complex with another micronutrient ion in the adjacent soil solution, restarting the cycle.
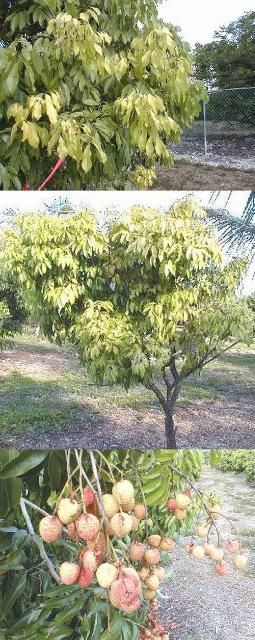
Credit: Yuncong Li, UF/IFAS
Chemical reactions between micronutrient chelates and soil can be avoided by using a foliar application. Chelated nutrients also facilitate nutrient uptake efficiency for foliar application because crop leaves are naturally coated with wax that repels water and charged substances, such as ferrous ions. The organic ligand around the chelated micronutrient can penetrate the wax layer, thus increasing iron uptake (Figure 2). Compared to traditional iron fertilization, chelated iron fertilization is significantly more effective and efficient (Figure 3) than non-chelated fertilizer sources.
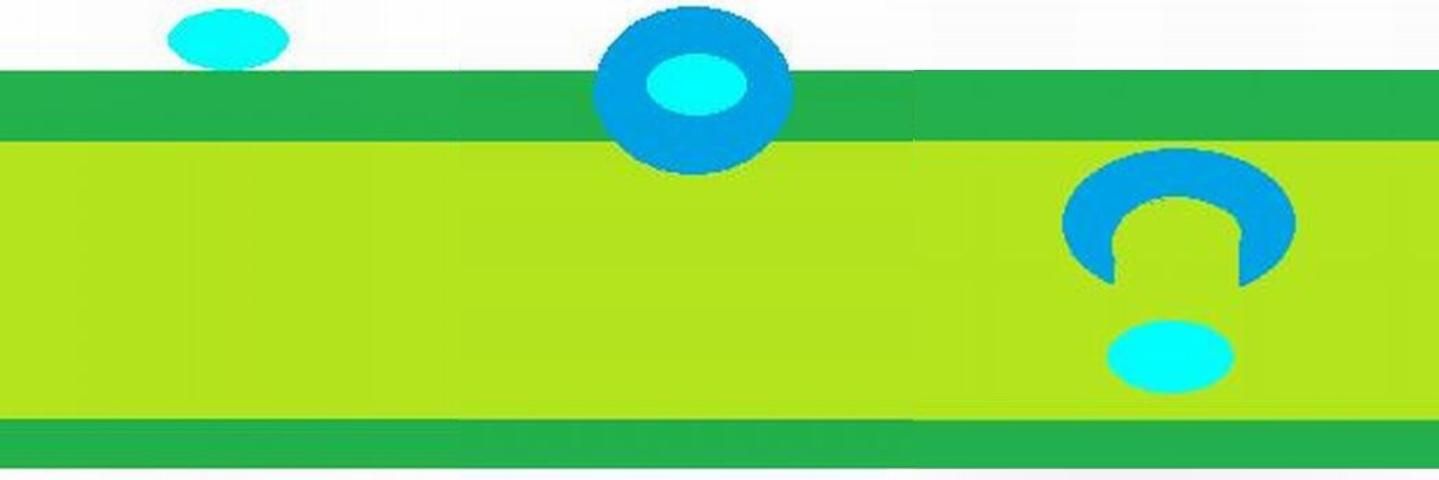
Credit: Fullerton (2004)
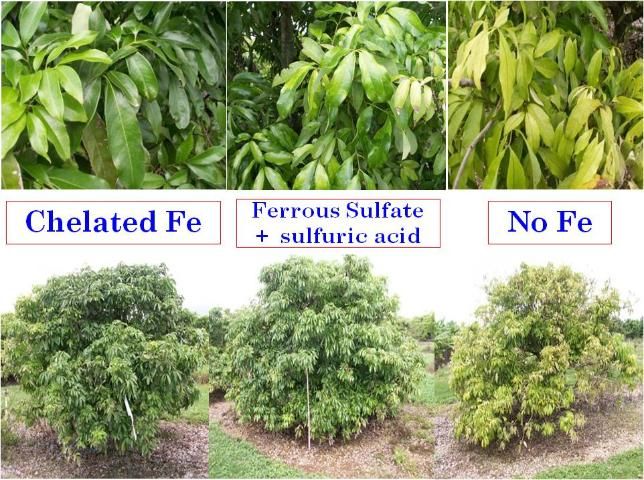
Credit: Yuncong Li, UF/IFAS
Therefore, chelated fertilization can improve micronutrient use efficiency and make micronutrient fertilization more cost effective. The images in Figure 3 show the difference in three treatments with lychee: chelated Fe(II) is greener than FeSO4 plus sulfuric acid, and FeSO4 plus sulfuric acid is greener than no iron fertilization (Schaffer et al. 2011).
Which crops often need chelated fertilizers?
Vegetable and fruit crop susceptibility to micronutrients differs significantly (Table 2). For those in the highly or moderately susceptible categories, chelated fertilizers are often needed. For those with low susceptibility, no chelated fertilizers are needed unless the soil is low in micronutrient bioavailability, as demonstrated by a soil test. Soil pH is a major factor influencing micronutrient bioavailability; therefore, if soil pH is greater than 6.5, then the soil may have limited micronutrient bioavailability, and chelated fertilizers may be needed.
Which chelated fertilizer should be used?
Each of the ligands (Table 1) can form a chelated fertilizer with one or more micronutrients. The effectiveness and efficiency of a particular chelated fertilizer depends on the pH of the plant growth medium.
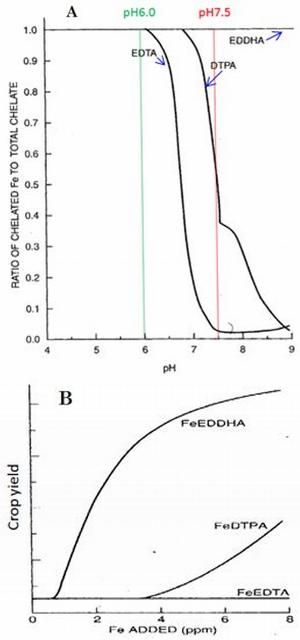
Credit: (A) Havlin et al. (2005), Norvell (1972); (B) Havlin et al. (2005), Lindsay (1974)
The ligands EDTA, DTPA, and EDDHA are often used in chelated fertilizers (Table 4). Their effectiveness differs significantly. EDDHA chelated Fe is most stable at soil pH greater than 7 (Figure 4, A and B). Chelated fertilizer stability is desired because it means the chelated micronutrient will remain in a bioavailable form for a much longer time period, thus increasing micronutrient use efficiency in vegetable and fruit production. The stability of three typical chelated Fe fertilizers varies at different pH conditions (Figure 4, A). The Y-axis represents the ratio of chelated Fe to total chelate and ranges from 0 to 1.0. A value of 1.0 means the chelate is stable. The X-axis represents soil pH. At 6.0, the ratios for all three chelated Fe fertilizers are 1.0 (stable), but at pH 7.5, only the ratio of EDDTA chelated Fe is 1.0. That of DTPA chelated Fe is only 0.5, and that of EDTA chelated Fe is only 0.025. So, in practice, EDDTA chelated Fe fertilizer is most effective when pH is greater than 7 but most costly. Accordingly, crop yields of these three chelated fertilizers are in this order: FeEDDHA > FeDTPA > FeEDTA (Figure 4, B). See Micronutrient Deficiencies in Citrus: Iron, Zinc, and Manganese (https://edis.ifas.ufl.edu/publication/ss423) for effective pH ranges of iron chelates. Table 3 shows the relationship between soil pH and chelated fertilizer requirement.
Correction of Fe deficiency depends on individual crop response and many other factors. For instance, for vegetables, the rate is usually 0.4–1 lb. chelated Fe in 100 gal. of water per acre. Deciduous fruits need 0.1–0.2 lb. chelated Fe in 25 gal. of water per acre (Table 5). Foliar application is more effective than soil application. For foliar application, either inorganic or chelated Fe is effective, but for fertigation, chelated Fe should be used. In high pH soil, crops are also vulnerable to Cu deficiency stresses. Chelated Cu is significantly more effective than inorganic Cu. A commonly used copper chelate is Na2CuEDTA, which contains 13% Cu. Natural organic materials have approximately 0.5% Cu (Table 5).
In addition to soil pH, Mn is also influenced by aeration, moisture, and organic matter content. Chelated Mn can improve Mn bioavailability. Mn deficiency occurs more often in high pH and dry soil. Similar to other micronutrients, foliar spray is much more effective than soil application. For commercial vegetable production, 0.2–0.5 lb. MnEDTA in 200 gal. of water per acre can effectively correct Mn deficiency (Table 5). Zinc is another micronutrient whose bioavailability is closely associated with soil pH. Crops may be susceptible to Zn deficiency in soil with pH > 7.3. Spraying 0.10–0.14 lb. chelated Zn in 100 gal. of water per acre is effective (Poh et al. 2009). Animal waste and municipal waste also contain Cu, Mn, and Zn micronutrients (Table 5). For more information about micronutrient deficiency in crops, see Plant Tissue Analysis and Interpretation for Vegetable Crops in Florida (https://edis.ifas.ufl.edu/publication/ep081), Micronutrient Deficiencies in Citrus: Iron, Zinc, and Manganese (https://edis.ifas.ufl.edu/publication/ss423), and Iron (Fe) Nutrition of Plants (https://edis.ifas.ufl.edu/publication/ss555).
Practical Take-Home Message
- High pH soil (pH > 6.5) often has low bioavailability in micronutrients such as Fe, Mn, Zn, and Cu, and micronutrient fertilizers are needed for commercial crop production.
- Crop susceptibility to the above micronutrient deficiencies depends on the plant species and cultivar. Commercial crops can be categorized into three susceptibility groups: high, medium, and low. The first two groups often need chelated fertilizers.
- Inorganic water-soluble micronutrient application to the soil is often ineffective for correcting micronutrient disorders.
- Chelated fertilizers are less reactive to soil conditions and can significantly enhance nutrient uptake and utilization efficiencies.
- Chelate fertilization rates range from 0.2 to 1 lb. micronutrient per acre for vegetable production and 0.1–0.5 lb. micronutrient per acre for fruit production.
- Foliar application of chelated fertilizers is often more effective than soil application.
References
Alloway, B. J. 2008. Micronutrient Deficiencies in Global Crop Production. Heidelberg, Germany: Springer Science + Business Media, B. V. Berlin.
Fullerton, T. 2004. "Chelated Micronutrients." Agro Services International Inc. http://www.agroservicesinternational.com/Articles/Chelates.pdf.
Havlin, J. L., J. D. Beaton, S. L. Tisdale, and W. L. Nelson. 2005. Soil Fertility and Fertilizers: An Introduction to Nutrient Management (7th ed.). Upper Saddle River, NJ: Pearson Education.
Lindsay, W. L. 1974. "Role of Chelation in Micronutrient Availability." In The Plant Root and Its Environment, edited by E. E. Carson, 507-524. Charlottesville: University Press of Virginia.
Morgan, B., and O. Lahav. 2007. "The Effect of pH on the Kinetics of Spontaneous Fe (II) Oxidation by O2 in Aqueous Solution – Basic Principles and a Simple Heuristic Description." Chemosphere 68(11): 2080–2084.
Norvell, W. A. 1972. "Equilibria of Metal Chelates in Soil Solution." In Micronutrients in Agriculture, edited by J. J. Mortvedt, P. M. Giordano, and W. L. Lindsay, 115–136. Madison, WI: Soil Science Association of America.
Sekhon, B. S. 2003. "Chelates for Micronutrient Nutrition among Crops." Resonance 8(7): 46–53. https://doi.org/10.1007/BF02834402
Schaffer, B., J. H. Crane, C. Li, Y. C. Li and E. A. Evans. 2011. "Re-Greening of Lychee (Litchi chinensis Sonn.) Leaves with Foliar Applications of Iron Sulfate and Weak Acids." Journal of Plant Nutrition 34(9): 1341–1359.
Selected vegetable and fruit crop species' relative susceptibility* to some micronutrient deficiencies.