Recent technology has been developed to precisely engineer genes for traits of interest. This approach is known as "CRISPR" gene editing. Gene editing is distinct from other forms of genetic engineering, such as transgenic technology, often colloquially referred to as "GMO". With gene editing, the final product can match that obtained by conventional plant breeding, but in a much shorter timeframe. CRISPR has been applied in many agronomic crops and is poised to make contributions in strawberry. We anticipate that, over the next decade, CRISPR and other gene editing techniques will be used to rapidly develop elite strawberry varieties with improved disease resistance, fruit quality, and other valuable attributes.
What is CRISPR gene editing?
One of the great disappointments in the pursuit of improved varieties is the discovery of a new advanced selection that would be valued by the industry except for one critical flaw. Gene editing technology can be used to almost surgically adjust the gene or genes behind that deleterious trait. CRISPR (Clustered Regularly Interspaced Short Palindromic Repeats) is one form of gene editing that can be used to precisely modify a gene of interest without otherwise compromising the favorable traits of an elite variety (Rani et al. 2016; Bortesi and Fischer 2015). For example, instead of breeding for many years to move a disease resistance gene from a wild strawberry into a modern strawberry, gene editing allows a direct introduction of the genetic information. Think of it as a "cut and paste" mechanism. This is particularly useful for cultivated strawberries because they are genetically complex, making conventional breeding difficult. The UF/IFAS strawberry breeding program has identified several important gene regions controlling disease resistance traits that are directly relevant to Florida growers. By using CRISPR technology, these genes or gene variants can be moved into desirable genetic backgrounds that can be further moved via conventional crossing in later generations. We can utilize established DNA marker-assisted breeding tools to track the edited genes in subsequent generations, adding to the speed of new variety development. Based on policy discussions, it is possible that the first-generation plants containing the edited genes will not require extensive regulation, and these tools will be extremely valuable in the long-term efforts of every strawberry breeding program.
Does CRISPR gene editing = transgenic technology?
"Transgenic technology" refers to the transfer of a genetic material from one species to another. CRISPR, on the other hand, can be used to precisely change DNA sequence, switching it from one naturally occurring variant to another naturally occurring variant. Using this new technique, we can cut a strawberry's genome at a desired location so that existing genes can be removed or added. Early indications suggest that gene editing should be regulated like conventionally bred crops, as the final product can simply match what may be done by conventional breeding. Recently, the USDA announced that it would not regulate a new mushroom developed using CRISPR (Waltz 2016; Hoffman 2021). Countries like Sweden and Argentina have made similar proclamations, indicating that the finished CRISPR-edited varieties do not fall under certain regulations because they do not contain "foreign" DNA. We published another article about CRISPR gene editing in crop improvement (https://edis.ifas.ufl.edu/publication/HS1334).
Development of a CRISPR System in Strawberry
While the finished varieties do not contain DNA from other organisms, the process introduces genetic information that orchestrates the desired genetic change. The first step is to develop and optimize a tissue culture and transformation system (protocols to introduce foreign DNA to new plants) for UF strawberry lines, so that new plants can be regenerated from cells containing introduced DNA. However, just as each cultivar has different traits and qualities, they also behave differently with respect to introduction of new genes.
The major strawberry cultivars grown in Florida such as ‘Florida Radiance’, Sweet Sensation® 'Florida127' and 'Florida Brilliance' were used for tissue culture optimization. As shown in Figure 1, callus induction was tested with different strawberry tissues, and embryogenic callus growth was most vigorous on stolons (runners) and petioles. To identify the optimal conditions for shoot and root regeneration for ‘Florida Radiance’, Sweet Sensation® 'Florida127' and 'Florida Brilliance', explants were grown on a range of media with varying compositions of plant growth regulators.
C:\LyraEDISServlet?command=getScreenImage&oid=15623809
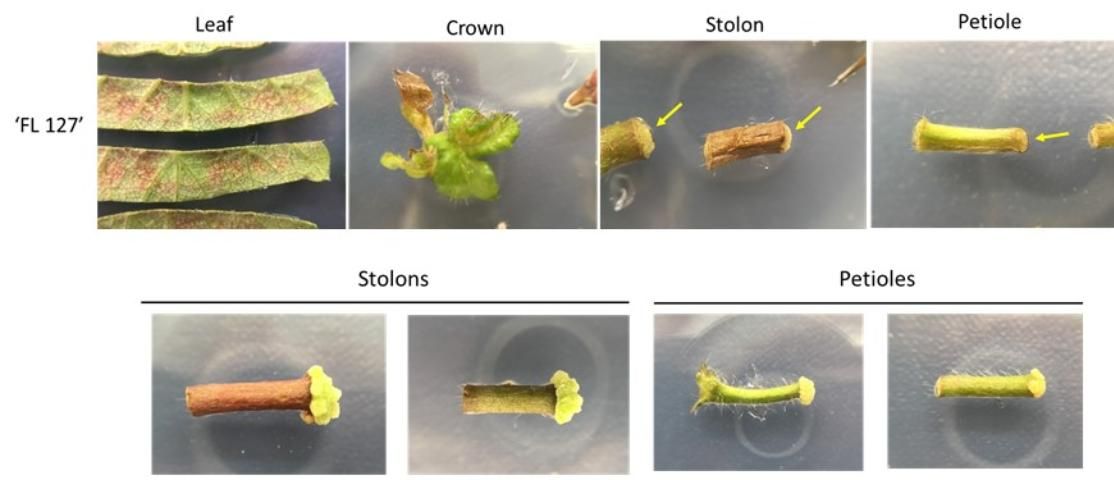
Credit: Cheolmin Yoo
About one inch of petiole or stolon from the leaf-end or shoot-end, respectively, were collected from greenhouse grown plants and used for the tissue culture process. Optimal conditions for tissue culture medium, nutrient, and hormone were tested for the UF accessions. The runner (stolon) produced calli more vigorously than petiole segments. It takes about 14 weeks for ‘Florida Radiance’, Sweet Sensation® 'Florida127' and 'Florida Brilliance' to develop from embryogenic callus to young plantlet in rooting media (Figure 2). 'Florida Brilliance' produced more regenerated plants than Sweet Sensation® 'Florida127'.
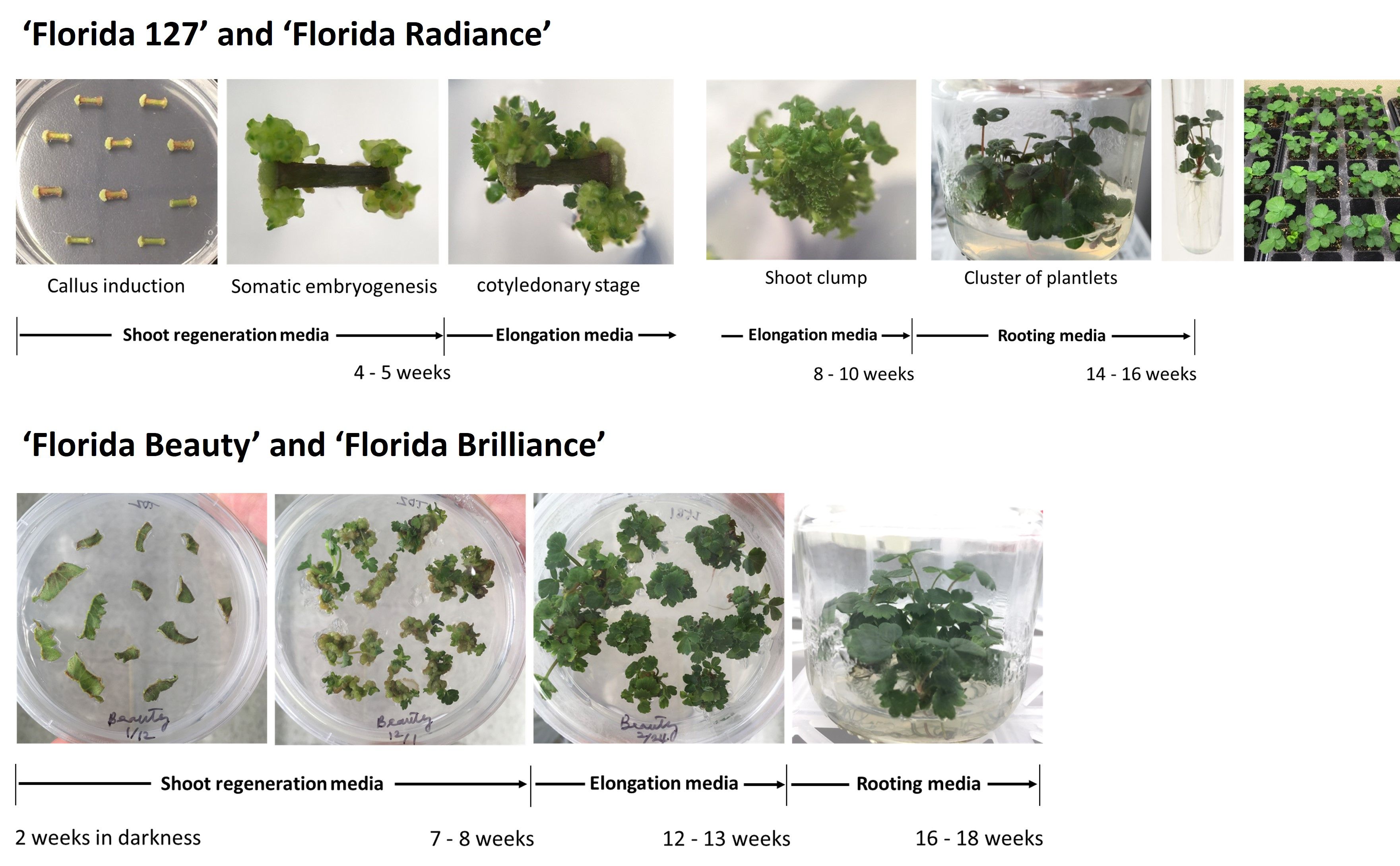
No "Foreign" DNA Sequences
Once the genetically engineered gene product is ready for CRISPR gene editing, transformation, where the new genetic material is delivered to a single strawberry cell, is the first step in the genetic engineering process. For DNA delivery, Agrobacterium tumefaciens‐mediated transformation is widely used for CRISPR gene editing. Agrobacterium tumefaciens is a widespread, naturally occurring soil bacterium that causes crown gall in many plant species and has the ability to introduce new genetic material into plant cells (Gelvin 2003). This bacterium works as a natural genetic engineer and is used in labs for plant transformation. Gene-edited plants using Agrobacterium-mediated transformation will contain foreign bacterial DNA sequences. It is not an easy process to remove the bacterium-derived DNA sequences through breeding.
In contrast, we are currently using a biolistic particle bombardment method in which DNA-coated metal particles are delivered to the plant cells using a "gene gun" (Figure 3). This method can be applied to a wide range of cell and tissue types, and there is no need for bacterial DNA insertion. Later, the gene edited tissues can be regenerated to mature plants using the tissue culture protocol outlined above. The GFP was delivered to cells using a hand-held “gene gun”. When GFP is successfully delivered into cells, green fluorescence can be observed under a UV-microscopy (Figure 3B). Since we already understand the sequence of a gene involved in chlorophyll synthesis called the “Phytoene Desaturase (PDS)” gene, we have used CRISPR to interfere with the function of this gene as a way to test whether our strawberry CRISPR system works. Figure 2 shows that CRISPR precisely knocked out the chlorophyll gene in Sweet Sensation® (Figure 3C).
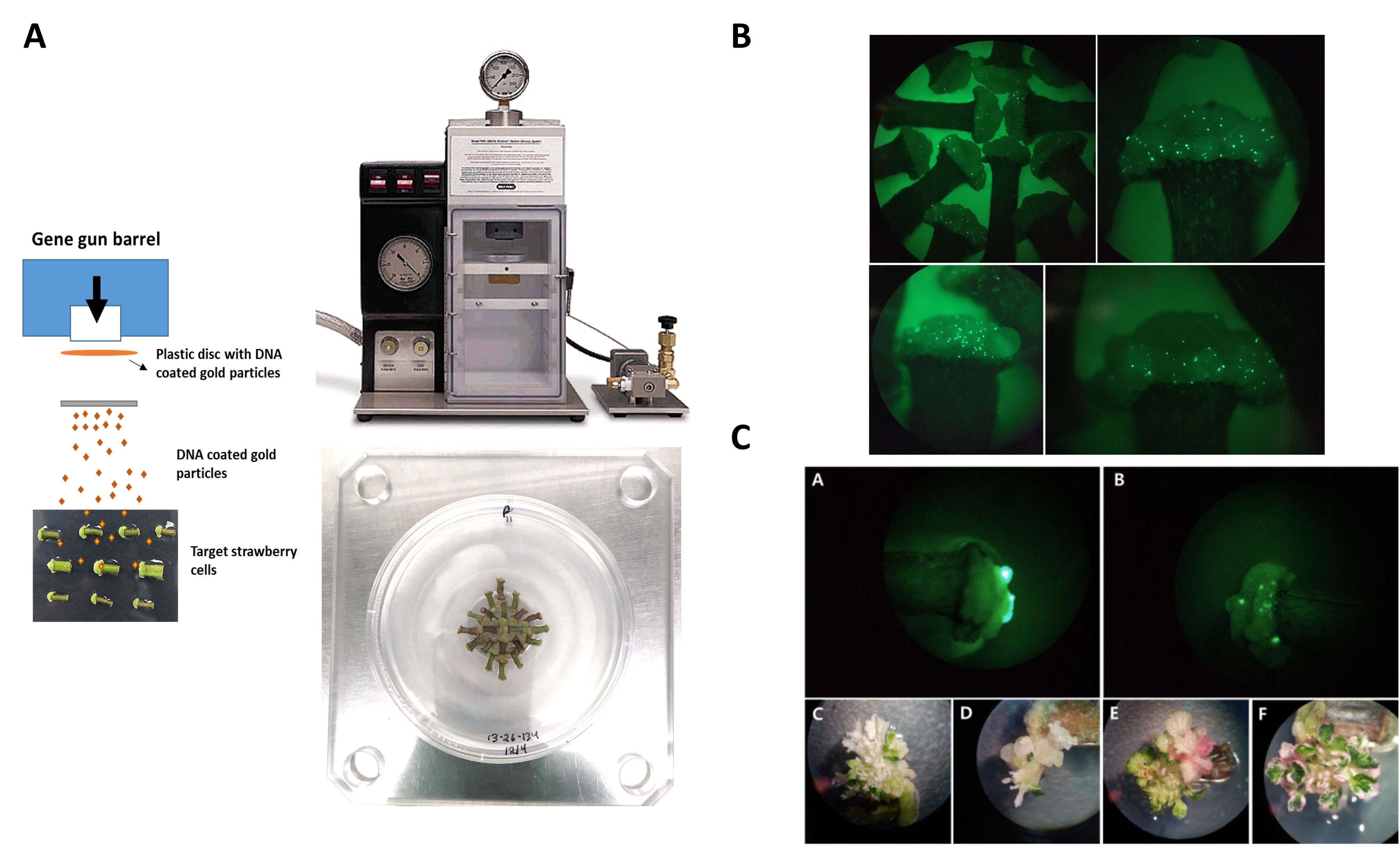
Credit: UF/IFAS and Jin-Hee Kim
Recently, the development of gene editing using protoplasts and regeneration of plants from protoplasts has been demonstrated in other plant species (Woo et al. 2015; Kanchiswamy 2016; Dutt et al. 2015). This method is known as a completely "DNA-free" gene editing system. Briefly, the protein/genetic material complex for gene editing will be assembled in vitro and the complex mixed with strawberry protoplast isolated from embryogenic calli and polyethylene glycol, which allows direct transfer by endocytosis into protoplasts. The gene edited with protoplasts is cultured (cell suspension culture) into calli, and mature plants can be regenerated using the tissue culture protocol outlined above. We are currently developing the protocol of CRISPR gene editing with strawberry protoplast (Figure 4).
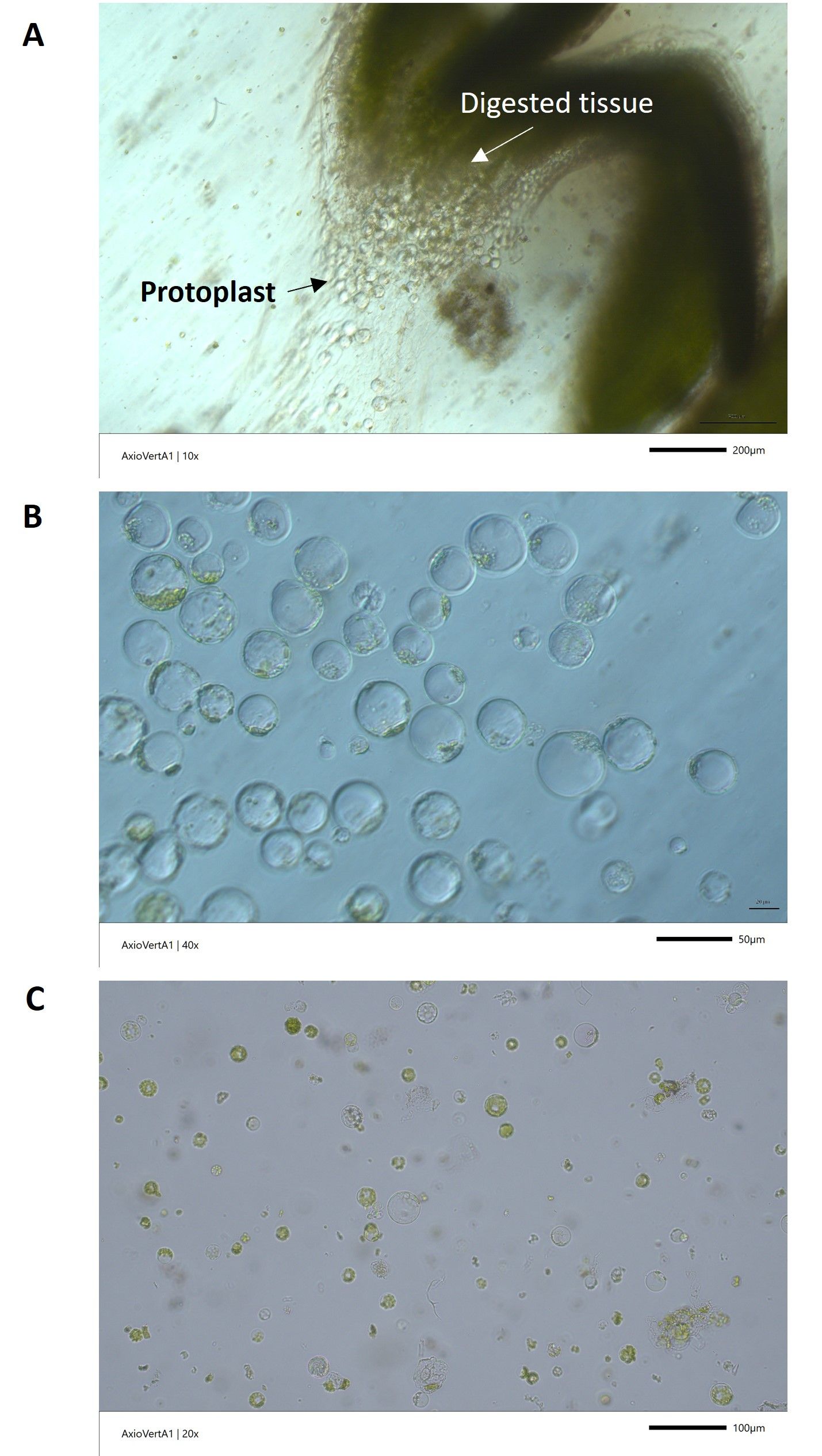
Credit: undefined
Gene Editing for Disease Resistance
The UF strawberry breeding program provides a direct commercialization path for disease-resistant varieties to reach Florida growers. New varieties with better resistance will bring increased profitability to the Florida strawberry industry. The Florida Strawberry Growers Association estimates that diseases cost the Florida industry at least $15 million each year. In the last five years, the UF strawberry breeding program has identified regions of strawberry chromosomes that control fruit flavor and multiple disease resistance (Barbey et al. 2021; Chandra et al. 2021; Nelson et al. 2021; Noh et al. 2018; Oh et al. 2021; Oh et al. 2020; Oh et al. 2019; Salinas et al. 2020). Efforts are ongoing to narrow these chromosomal regions down and identify the exact gene sequences that provide these disease resistances, with a recent emerging strawberry disease, Neopestalotiopsis, resistance as the first priority. Our goal is to add Neopestalotiopsis resistance to 'Florida Brilliance', Sweet Sensation® 'Florida127,' and new cultivars such as ‘Florida Medallion’, which are currently highly susceptible to this disease. Evaluations of the gene-edited lines will be performed in concert with crosses to integrate the changes into other major varieties and advanced selections with conventional hybridization.
References
Barbey CR et al. 2021. “Genetic analysis of methyl anthranilate, mesifurane, linalool, and other flavor compounds in cultivated strawberry (Fragaria × ananassa)” Frontiers in Plant Science. 12: 615749.
Bortesi, L. and R. Fischer. 2015. "The CRISPR/Cas9 system for plant genome editing and beyond." Biotechnol Adv. 33(1): 41‒52.
Chandra S et al. 2021. “Comparative transcriptome analysis to identify candidate genes for FaRCg1 conferring resistance against Colletotrichum gloeosporioides in cultivated strawberry (Fragaria × ananassa)” Frontiers in Genetics. 12: 730444.
Dutt, M. et al. 2015. "Transgenic Citrus Expressing an Arabidopsis NPR1 Gene Exhibit Enhanced Resistance against Huanglongbing (HLB; Citrus Greening)." PLoS One 10(9):e0137134.
Gelvin, S.B. 2003. "Agrobacterium-mediated plant trans-formation: the biology behind the "gene-jockeying" tool." Microbiol Mol Biol Rev. 67(1):16‒37.
Hoffman, N. E. “Revisions to USDA biotechnology regulations: The SECURE rule.” Proceedings of the National Academy of Sciences of the United States of America.
Kanchiswamy, C.N. 2016. "DNA-free genome editing meth-ods for targeted crop improvement." Plant Cell Rep. 35(7): 1469‒1474.
Mangandi, J. et al. 2017. "Pedigree-Based Analysis in a Mul-tiparental Population of Octoploid Strawberry Reveals QTL Alleles Conferring Resistance to Phytophthora cactorum." G3 7(6): 1707‒1719.
Nelson JR et al. 2021. “Discovery of three loci increasing resistance to charcoal rot caused by Macrophomina phaseolina in octoploid strawberry.” G3 11:jkab037.
Noh Y-H et al. 2018. “High-throughput marker assays for FaRPc2-mediated resistance to Phytophthora crown rot in octoploid strawberry.” Molecular Breeding 38:104.
Oh Y et al. 2021. “Genomic characterization of the fruity aroma gene, FaFAD1, reveals a gene dosage effect on γ-decalactone production in strawberry (Fragaria × ananassa).” Frontiers in Plant Science 12: 639345.
Oh Y, et al. 2020. “Development of subgenome-specific markers for FaRXf1 conferring resistance to bacterial angular leaf spot in allo-octoploid strawberry.” Int J Fruit Sci. 1-13.
Oh Y, et al. 2019. “The strawberry DNA testing handbook.” HortScience 54: 2267-2270.
Rani, R., et al. 2016. "CRISPR/Cas9: a promising way to exploit genetic variation in plants." Biotechnol Lett.
Roach, J.A., et al. 2016. "FaRXf1: a locus conferring resistance to angular leaf spot caused by Xanthomonas fragariae in octoploid strawberry." Theor Appl Genet. 129(6): 1191‒1201.
Salinas N, Fan Z, Peres N, Lee S, Whitaker VM (2020) FaRCa1 confers moderate resistance to the root necrosis form of strawberry anthracnose caused by Colletotrichum acutatum HortScience 55:693-698
Waltz, E. 2016. "Gene-edited CRISPR mushroom escapes US regulation." Nature 532(7599): 293.
Woo, J.W., et al. 2015. "DNA-free genome editing in plants with preassembled CRISPR-Cas9 ribonucleoproteins." Nat Biotechnol. 33(11): 1162‒1164.