Introduction
This publication outlines how the bacterium Wolbachia pipientis is used as a tool to control mosquito populations and reduce the spread of mosquito-borne disease. Its intended audience is the general public, mosquito control professionals, and others interested in mosquitoes and mosquito control. Biting mosquitoes transmit many pathogens that cause deadly diseases in people, our pets, and our livestock. This includes diseases like malaria, which is caused by Plasmodium parasites (a genus of single-celled parasitic organisms), viral diseases like dengue and West Nile fever, and diseases like filariasis, which is caused by parasitic worms.
Mosquito-borne diseases result in millions of infections and hundreds of thousands of deaths every year. Effective vaccines for most of these diseases have not been developed or are not currently available to the general public. Accordingly, disease management is focused on controlling mosquito populations, most commonly by treatment with chemical insecticides. However, overreliance on these chemicals over the past fifty years has reduced their effectiveness, and mosquito populations around the world have developed resistance. This has driven scientists to explore new options for controlling mosquitoes, including using the bacterium Wolbachia pipientis.
What are Wolbachia?
Wolbachia are gram-negative bacteria (a group of bacteria with a thin cellular wall) from class Alphaproteobacteria. They are commonly found in insects in nature as they need to live inside the cells of an invertebrate animal host in order to survive (Figure 1). It is estimated that 40–60% of all terrestrial insects naturally carry Wolbachia, including many fruit flies, beetles, and moths. Wolbachia is also common in non-insect invertebrates like spiders, scorpions, and nematode worms. Wolbachia are not capable of infecting vertebrates, including people, mammals, birds, fish, amphibians, or reptiles.
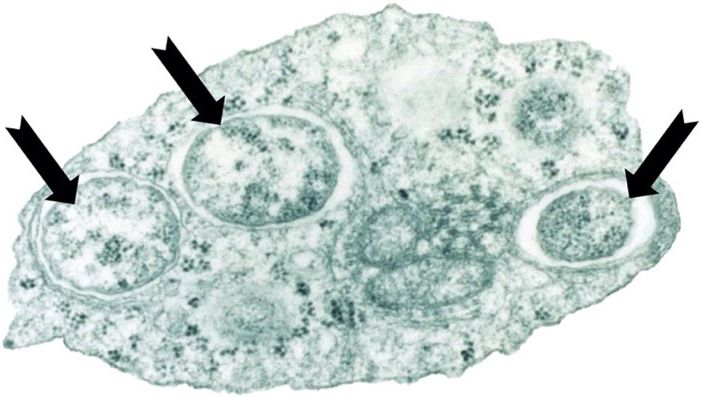
Credit: Dr. Scott L. O’Neill and published in the journal PLoS Biology. It is used here under a creative commons attribution 2.5 generic license. The three black arrows were added by the authors.
Wolbachia spread from mother to offspring, and they often modify their host’s biology to promote maternal transmission. For example, Wolbachia cause a reproductive manipulation known as cytoplasmic incompatibility, which is common in Wolbachia-carrying mosquitoes and other insects (Figure 2). Cytoplasmic incompatibility occurs when Wolbachia-carrying males mate with Wolbachia-free females and the result of these pairings is that the eggs produced do not hatch. In contrast, Wolbachia-carrying female insects are capable of producing live larvae after mating with either Wolbachia-carrying or Wolbachia-free males. This provides a comparative advantage to Wolbachia-carrying insects, as their offspring make up a larger part of the next generation. Cytoplasmic incompatibility helps to promote the spread of Wolbachia within insect populations over time and is a major reason why Wolbachia is so commonplace in nature.
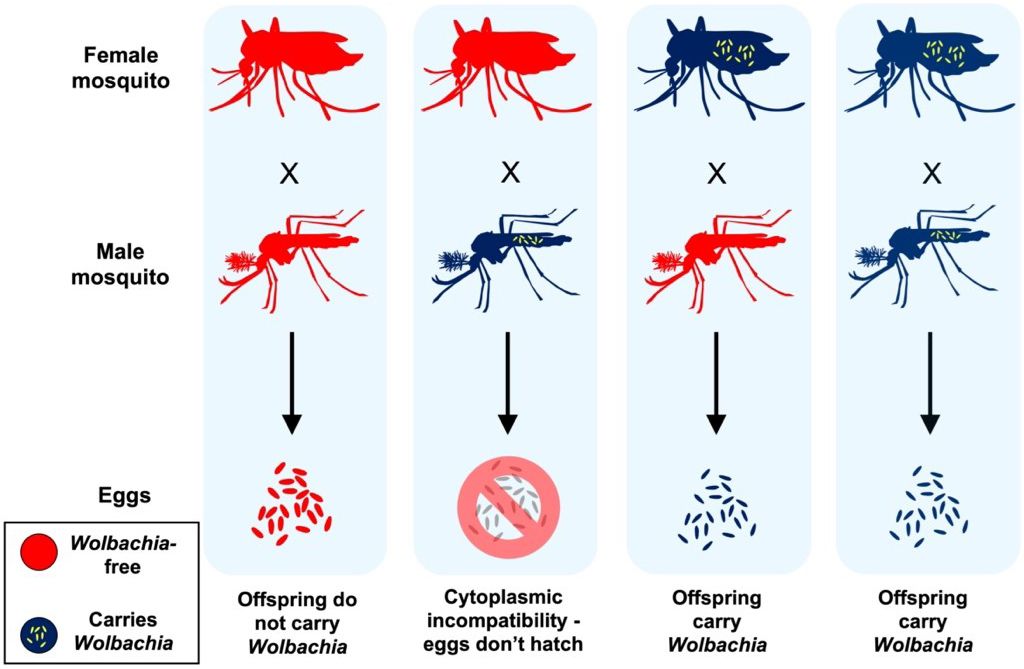
Credit: E. P. Caragata, UF/IFAS
Why are Wolbachia-carrying mosquitoes important to control of mosquitoes and mosquito-borne disease?
Wolbachia are naturally found in some mosquito species, including Culex pipiens (the common house mosquito) and Aedes albopictus (the tiger mosquito). However, Wolbachia is not naturally found in other important mosquito species such as Aedes aegypti (the yellow fever mosquito), which transmits dengue, chikungunya, yellow fever and Zika viruses, and Anopheles mosquito species, which spread malaria-causing Plasmodium parasites. Researchers have observed that natural Wolbachia infections protect Drosophila fruit flies from virus-induced mortality. To see if virus blocking effects occurred in mosquito species that transmit human pathogens, researchers generated Wolbachia infections in Aedes aegypti, through a process known as transinfection. They isolated Wolbachia from one host insect and injected it into newly laid mosquito eggs to produce Ae. aegypti mosquito colonies that persistently carry Wolbachia.
When Wolbachia-carrying Ae. aegypti mosquitoes were exposed to viruses in the laboratory, researchers observed that Wolbachia made them less likely to become infected. Their cells and tissues were less hospitable for pathogen infection, and they were less likely have pathogens detected in their saliva or to transmit the pathogen when they bit a human. In Wolbachia-transinfected Ae. aegypti, blocking has been observed to be effective against many pathogens, including dengue and Zika viruses. In transinfected Anopheles stephensi, Wolbachia blocks the human malarial parasite Plasmodium falciparum. The way that Wolbachia blocks these pathogen infections is not fully understood, but researchers believe it is linked to changes that Wolbachia makes to mosquito immunity, metabolism, and host cellular components used to produce RNA and proteins, which are commonly exploited by pathogens when they infect mosquitoes. The fact that Wolbachia infections in mosquitoes cause pathogen blocking and/or cytoplasmic incompatibility has been exploited to control mosquito populations through two different techniques known as population suppression and population replacement.
How can Wolbachia be used to suppress mosquito populations?
Population suppression aims to reduce the size of a target mosquito population, which will result in people experiencing fewer mosquito bites and, thus, fewer infections. This approach relies on cytoplasmic incompatibility to “crash,” or suppress a target mosquito population, and involves the release of large numbers of Wolbachia-carrying male mosquitoes into nature (Figure 3). Because male mosquitoes do not bite, the public may view this approach favorably. Before they are released, male pupae or adults must be separated from females. Researchers must take care not to include female mosquitoes in their releases. Only the males are required to induce cytoplasmic incompatibility. Accidentally releasing a large number of Wolbachia-infected females could lead to Wolbachia becoming established at the release site, which would reduce the level of suppression possible in future releases.
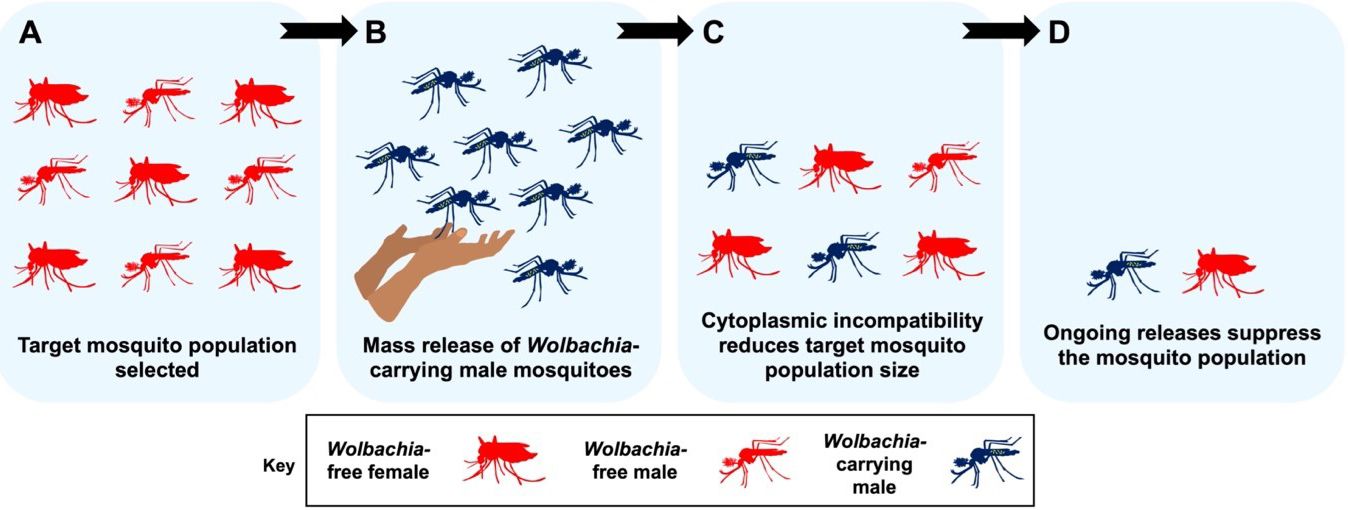
Credit: E. P. Caragata, UF/IFAS
Released males mate with wild, Wolbachia-free female mosquitoes, and because of cytoplasmic incompatibility, the eggs laid by those female mosquitoes do not hatch. Because female mosquitoes typically mate with only one male mosquito in their lives, these females will never produce living offspring. Releasing very large numbers of male, Wolbachia-carrying mosquitoes can help them outcompete wild males. If releases continue for a sufficient period of time, they can lead to drastic decreases in the number of mosquitoes in an area, because the eggs of any Wolbachia-free female mosquito that mates with a Wolbachia-carrying male will not hatch. However, if suppression is not complete, or if mosquitoes enter from surrounding areas, new releases may be needed to keep mosquito numbers low. Ongoing financial costs associated with monitoring mosquito numbers and performing additional releases may be necessary to achieve persistent control of mosquito populations.
Population suppression with Wolbachia has already been tested against populations of Ae. aegypti and Ae. albopictus mosquitoes in several countries, including China, Singapore, and the United States. A large-scale project in Singapore, called Project Wolbachia, released millions of male Ae. aegypti mosquitoes into an area of more than 300,000 households. They observed in excess of 90% suppression of mosquito populations and a 65–80% decrease in dengue cases. Another large-scale project in China targeting Ae. albopictus released tens of millions of males, which caused a 94% decrease in local mosquito numbers. As of 2024, releases in the United States have been relatively small in scale and designed not to suppress populations over large areas of the country but rather as proof of concept, to show that this control strategy is safe and can be successful. Example releases in Fresno, California, and in Miami, Florida, have been promising, resulting in mosquito population suppression of 95% and 78%, respectively.
Population suppression using Wolbachia can quickly reduce the size of local mosquito populations and can be an effective way of preventing cases of human disease. It can be used together with other types of mosquito control, reducing the need to use chemical insecticides. And the approach is unlikely to harm beneficial insects, including pollinators. These benefits, combined with the fact that released male mosquitoes are incapable of producing progeny with wild female mosquitoes, make this approach comparatively ecologically friendly. Given the many strengths of this method, we are likely to see widespread adoption of the use of Wolbachia to suppress mosquito populations in the coming decade.
How can Wolbachia be used to replace mosquito populations?
The second form of mosquito control involving Wolbachia seeks to replace wild, disease-spreading mosquito populations with those that carry Wolbachia. The ultimate goal is to use Wolbachia to make a target mosquito population less likely to spread key pathogens such as dengue, chikungunya, and Zika (Figure 4). Accordingly, this approach relies on selecting Wolbachia that are good at stopping pathogens from infecting mosquitoes. It also depends on using cytoplasmic incompatibility to spread Wolbachia into the target mosquito population.
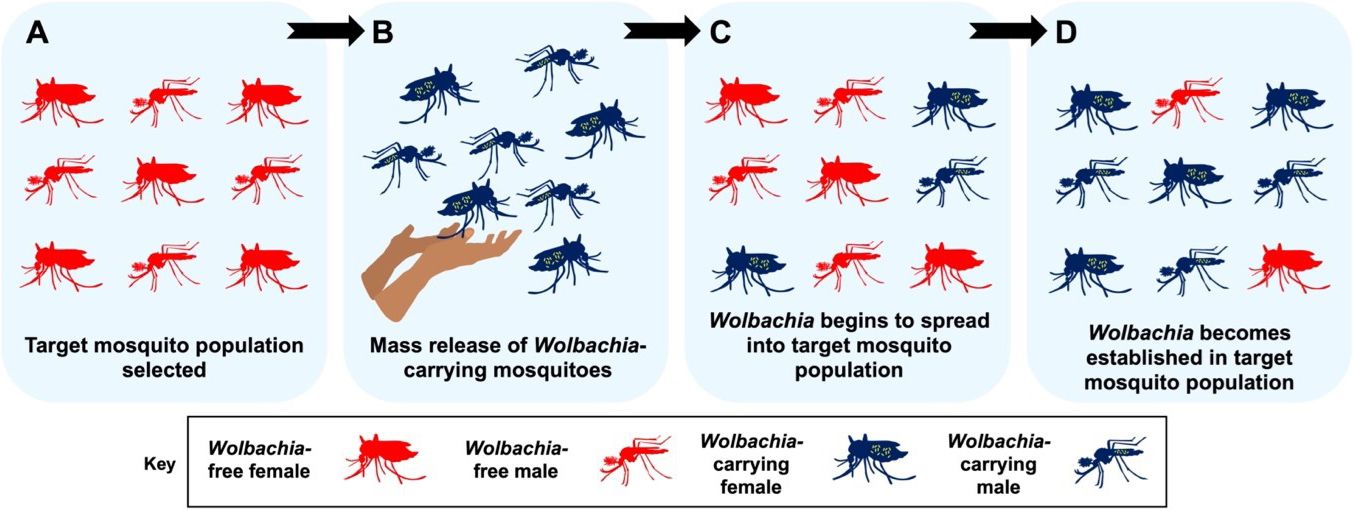
Credit: E. P. Caragata, UF/IFAS
Unlike the population suppression approach, which only involves releases of male mosquitoes, population replacement involves releases of both male and female mosquitoes. Released males cause cytoplasmic incompatibility, which helps to reduce the size of the local mosquito population. Released females lay many Wolbachia-carrying eggs, which helps to establish Wolbachia in the area. As time passes and releases continue, the percentage of the population that harbors Wolbachia will grow large enough for the bacteria to remain stable. At this point releases can be stopped, and if Wolbachia remains well established in the area, it may never be necessary to release mosquitoes again. For instance, Wolbachia infections of Aedes aegypti populations in North Queensland, Australia, have been stable for more than a decade.
Population replacement programs involving Wolbachia have been used for many years in countries such as Australia, Brazil, Indonesia, and Vietnam by the World Mosquito Program, and in Malaysia by the Wolbachia Malaysia Project. In these projects, the target mosquito species has been Aedes aegypti. Their results have been highly consistent and show that releasing mosquitoes for only a few weeks or months will result in Wolbachia becoming established in the target mosquito populations. Many of these projects have also seen decreases in arboviral infections in human communities in release areas.
For example, in Rio de Janeiro, Brazil, releases through 2021 involved 91 million mosquitoes in an area inhabited by 1.25 million people and resulted in a 60% drop in dengue and chikungunya infections in the area. In Yogyakarta, Indonesia, 1.75 million Wolbachia-carrying mosquito eggs were released. This resulted in very high levels of Wolbachia in mosquitoes across the release area and a 73% reduction in dengue cases. In Kuala Lumpur, Malaysia, releasing 71 thousand mosquitoes across nearly ten thousand households caused Wolbachia to become established in 98% of mosquitoes and reduced dengue cases by more than 40%.
Because the population replacement approach involves the establishment of Wolbachia in nature over the long term, it is important for researchers to evaluate any potential long-term impacts to people and the environment that result from Wolbachia-carrying mosquitoes. Although Wolbachia is very common in nature, it is not normally found in Ae. aegypti mosquitoes. As time passes, the way that Wolbachia affects its new mosquito host, or the viruses that come to infect that host might change in unexpected ways. Because of this, scientists must regularly demonstrate that the released Wolbachia-carrying mosquitoes are still capable of blocking the target viral infections, or new mosquito-borne pathogens that might emerge in the area. Fortunately, studies have shown that the amount of Wolbachia in mosquitoes, and their ability to block viruses were unchanged, even after years spent in nature, but this sustained success does not eliminate the need to monitor mosquito populations after releases stop.
Before the first population replacement experiments, scientists conducted studies to determine the risk of Wolbachia to the food chain. They observed no evidence that predators that normally eat mosquitoes, like spiders and small fish, can become infected with Wolbachia after they eat a Wolbachia-carrying mosquito. It is also important to build and maintain effective methods of communication with local stakeholders in order to demonstrate the necessity and benefits of new types of mosquito control programs. For pathogen replacement programs, this community engagement is particularly important, because these programs involve releases of female mosquitoes, which will bite people in the release area, even if carrying Wolbachia means there is no clear risk of them spreading pathogens.
Future Prospects of Wolbachia-Based Mosquito Control
Both population suppression and replacement approaches have been very successful in achieving their goals: reducing mosquito population sizes for suppression and spreading Wolbachia into mosquito populations for replacement. Both approaches have also been linked to decreased disease incidence, a sign of the direct benefits of these strategies to public health. Accordingly, they are both becoming more commonly used around the world and the expectation is that this will likely continue in the future with new projects beginning in more countries. Neither approach is inherently better than the other. Each does what it does well, but there will be circumstances where one approach might be more suitable than the other, and that is a decision that should be left to local stakeholders. In a world where cases of mosquito-borne disease are increasing, it is important to have a variety of different mosquito control options available to decision makers as this will allow them to pick the most appropriate approach for their situation.
References
Bian, G., D. Joshi, Y. Dong, P. Lu, G. Zhou, X. Pan, Y. Xu, G. Dimpoulos, and Z. Xi. 2013. “Wolbachia Invades Anopheles stephensi Populations and Induces Refractoriness to Plasmodium Infection.” Science 340 (6133): 748–751. https://doi.org/10.1126/science.1236192
Caragata, E. P., H. L. C. Dutra, P. H. F. Sucupira, A. G. A. Ferreira, and L. A. Moreira. 2021. “Wolbachia as Translational Science: Controlling Mosquito-Borne Pathogens.” Trends in Parasitology 37 (12): 1050–1067. https://doi.org/10.1016/j.pt.2021.06.007
Caragata, E. P., S. Dong, Y. Dong, M. L. https://doi.org/10.1146/annurev-micro-011320-025557
. 2020. “Prospects and Pitfalls: Next-Generation Tools to Control Mosquito-Transmitted Disease.” Annual Review Microbiology 74:455–475.Crawford, J. E., D. W. Clarke, V. Criswell, M. Desnoyer, D. Cornel, B. Deegan, K. Gong, et al. 2020. “Efficient Production of Male Wolbachia-Infected Aedes aegypti Mosquitoes Enables Large-Scale Suppression of Wild Populations.” Nature Biotechnology 38 (4): 482–492. https://doi.org/10.1038/s41587-020-0471-x
Dutra, H. L., M. N. Rocha, F. B. Dias, S. G. Mansur, E. P. Caragata, and L. A. Moreira. 2016. “Wolbachia Blocks Currently Circulating Zika Virus Isolates in Brazilian Aedes aegypti Mosquitoes.” Cell Host and Microbe 19 (6): 771–774. https://doi.org/10.1016/j.chom.2016.04.021
Garcia, G. A., G. Sylvestre, R. Aguiar, G. Borges da Costa, A. J. Martins, J. Bento Pereira Lima, M. T. Petersen, et al. 2019. “Matching the Genetics of Released and Local Aedes aegypti Populations Is Critical to Assure Wolbachia Invasion.” PLoS Neglected Tropical Diseases 13 (1): e0007023. https://doi.org/10.1371/journal.pntd.0007023
Hedges, L. M., J. C. Brownlie, S. L. O'Neill, and K. N. Johnson. 2008. “Wolbachia and Virus Protection in Insects.” Science 322 (5902): 702. https://doi.org/10.1126/science.1162418
Hurst, T. P., G. Pittman, S. L. O'Neill, P. A. Ryan, H. L. Nguyen, and B. H. Kay. 2012. “Impacts of Wolbachia Infection on Predator Prey Relationships: Evaluating Survival and Horizontal Transfer between wMelPop Infected Aedes aegypti and Its Predators.” Journal of Medical Entomology 49 (3): 624–630. https://doi.org/10.1603/me11277
Indriani, C., W. Tantowijoyo, E. Rances,https://doi.org/10.12688/gatesopenres.13122.1
et al. 2020. “Reduced Dengue Incidence following Deployments of Wolbachia-Infected Aedes aegypti in Yogyakarta, Indonesia: A Quasi-Experimental Trial Using Controlled Interrupted Time Series Analysis.” Gates Open Research 4:50.Klasson, L., T. Walker, M. Sebaihia, M. J. Sanders, M. A. Quail, A. Lord, S. Sanders, et al. 2008. “Genome Evolution of Wolbachia Strain wPip from the Culex pipiens Group.” Molecular Biology and Evolution 25 (9): 1877–1887. https://doi.org/10.1093/molbev/msn133
Liu, N. 2015. “Insecticide Resistance in Mosquitoes: Impact, Mechanisms, and Research Directions.” Annual Review of Entomology 60:537–559. https://doi.org/10.1146/annurev-ento-010814-020828
Mains, J. W., C. L. Brelsfoard, P. R. Crain, Y. Huang, and S. Dobson. 2013. “Population Impacts of Wolbachia on Aedes albopictus.” Ecological Applications 23 (2): 493–501. https://doi.org/10.1890/12-1097.1
Mains, J. W., P. H. Kelly, K. L. Dobson, W. D. Petrie, and S. L. Dobson. 2019. “Localized Control of Aedes aegypti (Diptera: Culicidae) in Miami, FL, via Inundative Releases of Wolbachia-Infected Male Mosquitoes.” Journal of Medical Entomology 56 (5): 1296–1303. https://doi.org/10.1093/jme/tjz051
McMeniman, C. J., R. V. Lane, B. N. Cass,https://doi.org/10.1126/science.1165326
. 2009. Stable Introduction of a Life-Shortening Wolbachia Infection into the Mosquito Aedes aegypti.” Science 323 (5910): 141–144.Moreira, L. A., I. Iturbe-Ormaetxe, J. A. Jeffery, G. Lu, A. T. Pyke, L. M. Hedges, B. C. Rocha, et al. 2009. “A Wolbachia Symbiont in Aedes aegypti Limits Infection with Dengue, Chikungunya, and Plasmodium.” Cell 139 (7): 1268–1278. https://doi.org/10.1016/j.cell.2009.11.042
National Environment Agency, Singapore. 2022. “Wolbachia-Aedes Mosquito Suppression Strategy.” https://www.nea.gov.sg/corporate-functions/resources/research/wolbachia-aedes-mosquito-suppression-strategy
Nazni, W. A., A. A. Hoffmann, A. NoorAfizah, Y. L. Cheong, M. V. Mancini, N. Golding, G. M. R. Kamarul, et al. 2019. “Establishment of Wolbachia Strain wAlbB in Malaysian Populations of Aedes aegypti for Dengue Control.” Current Biology 29 (24): 4241–4248 e4245. https://doi.org/10.1016/j.cub.2019.11.007
Pinto, S. B., T. I. S. Riback, G. Sylvestre, G. Costa, J. Peixoto, F. B. S. Dias, S. K. Tanamas, et al. 2021. “Effectiveness of Wolbachia-Infected Mosquito Deployments in Reducing the Incidence of Dengue and Other Aedes-Borne Diseases in Niteroi, Brazil: A Quasi-Experimental Study.” PLoS Neglected Tropical Diseases 15 (7): e0009556. https://doi.org/10.1371/journal.pntd.0009556
Popovici, J., L. A. Moreira, A. Poinsignon, I. Iturbe-Ormaetxe, D. McNaughton, and S. L. Oneill. 2010. “Assessing Key Safety Concerns of a Wolbachia-based Strategy to Control Dengue Transmission by Aedes Mosquitoes.” Memórias do Instituto Oswaldo Cruz 105 (8): 957–964. https://doi.org/10.1590/s0074-02762010000800002
Ross, P. A., K. L. Robinson, Q. Yang, A. G. Callahan, T. L. Schmidt, J. K. Axford, M. P. Coquilleau, et al. 2022. “A Decade of Stability for wMel Wolbachia in Natural Aedes aegypti Populations.” PLoS Pathogens 18 (2): e1010256. https://doi.org/10.1371/journal.ppat.1010256
Ryan, P. A., A. P. Turley, G. Wilson, T. P. Hurst, K. Retzki, J. Brown-Kenyon, L. Hodgson, et al. 2019. Establishment of wMel Wolbachia in Aedes aegypti Mosquitoes and Reduction of Local Dengue Transmission in Cairns and Surrounding Locations in Northern Queensland, Australia.” Gates Open Research 3:1547. https://doi.org/10.12688/gatesopenres.13061.2
Teixeira, L., A. Ferreira, and M. Ashburner. 2008. “The Bacterial Symbiont Wolbachia Induces Resistance to RNA Viral Infections in Drosophila melanogaster.” PLoS Biology 6 (12): e2. https://doi.org/10.1371/journal.pbio.1000002
Walker, T., P. H. Johnson, L. A. Moreira, I. Iturbe-Ormaetxe, F. D. Frentiu, C. J. McMeniman, Y. S. Leong, et al. 2011. “The wMel Wolbachia Strain Blocks Dengue and Invades Caged Aedes aegypti Populations.” Nature 476 (7361): 450–453. https://doi.org/10.1038/nature10355
Weill, M., G. Lutfalla, K. Mogensen, F. Chandre, A. Berthomieu, C. Berticat, N. Pasteur, A. Philips, P. Fort, and M. Raymond. 2003. “Comparative Genomics: Insecticide Resistance in Mosquito Vectors.” Nature 423 (6936): 136–137. https://doi.org/10.1038/423136b.
Werren, J. H., L. Baldo, and M. E. Clark. 2008. “Wolbachia: Master Manipulators of Invertebrate Biology.” Nature Reviews Microbiology 6 (10): 741–751. https://doi.org/10.1038/nrmicro1969
World Health Organization. 2020. “Vector-borne diseases.” https://www.who.int/news-room/fact-sheets/detail/vector-borne-diseases
Wu, M., L. V. Sun, J. Vamathevan, M. Riegler, R. Deboy, J. C. Brownlie, E. A. McGraw, et al. 2004. “Phylogenomics of the Reproductive Parasite Wolbachia pipientis wMel: A Streamlined Genome Overrun by Mobile Genetic Elements.” PLoS Biology 2 (3): E69. https://doi.org/10.1371/journal.pbio.0020069
Xi, Z., C. C. Khoo, and S. L. Dobson. 2005. “Wolbachia Establishment and Invasion in an Aedes aegypti Laboratory Population.” Science 310 (5746): 326–328. https://doi.org/10.1126/science.1117607
Zheng, X., D. Zhang, Y. Li, C. Yang, Y. Wu, X. Liang, Y. Liang, et al. 2019. “Incompatible and Sterile Insect Techniques Combined Eliminate Mosquitoes.” Nature 572 (7767): 56–61. https://doi.org/10.1038/s41586-019-1407-9
Zug, R., and P. Hammerstein. 2012. “Still a Host of Hosts for Wolbachia: Analysis of Recent Data Suggests That 40% of Terrestrial Arthropod Species Are Infected.” PLoS One 7 (6): e38544. https://doi.org/10.1371/journal.pone.0038544