Abstract
Electropenetrography, formerly known as “electrical penetration graph” and henceforth abbreviated EPG, is used to study the feeding behavior of insects with piercing-sucking mouthparts. The technique uses sensitive electronic equipment to measure resistance and voltage when the insect and host are a part of an electronic circuit. The result is a sequence of waveforms that identify what the insect mouthparts are doing in the host. Although originally developed with aphids, electropenetrography is now applied more broadly. Consequently, more people will encounter results from experiments using EPG and how those results inform on overall pest biology.
Herein, we used the Asian citrus psyllid as an example because there is extensive literature on this insect, which spreads the causal agent (pathogen) of the citrus disease called citrus greening (a.k.a. huanglongbing). It is difficult to measure how an insect with piercing-sucking mouthparts feeds on a host plant because the insect’s mouthparts are small and hidden beneath opaque plant tissues. Electropenetrography is the only technique that can detect insect feeding behaviors in real-time while keeping both the insect vector and the plant host alive. Electropenetrography allows for a better understanding of host-insect-pathogen relationships, pesticide action, and the role of non-host plants. It can address fundamental ecological questions such as “How does an insect adapt to a host?” and more applied questions like “Does a pesticide kill an insect before the insect transmits a disease agent?” Electropenetrography can allow us to better understand challenges and, thus, improve our management decisions. The target audience for this publication includes scientists, Extension agents, and growers.
Introduction
Numerous insects feed on plant sap, leading to plant damage. The damage can reduce yield or yield quality and may result in economic losses. Which part of the plant an insect uses for food and water and the mechanisms by which the insect acquires these essentials defines the biological interactions between plants, insects, and pathogens. These interactions determine the outcomes of different crop protection strategies. This publication explains how some insects feed and how differing insect feeding behaviors can influence pest management outcomes. The details will focus on the Asian citrus psyllid, citrus greening (a.k.a. huanglongbing), and citrus pest management.
Many insects prefer to eat specific parts of the plant and use diverse behaviors to do so. These behaviors determine food availability and limit which pathogens insects can transmit. For example, a xylem-feeding insect would be a poor vector of a phloem-limited pathogen. Management strategies are also affected by insect feeding behavior. The management goal is to maximize plant health, usually through minimizing pest populations. This process starts by selecting a cultivar suited to the agricultural environment. Electropenetrography helps to identify host plant resistance in developing appropriate cultivars. Electropenetrography also helps to identify insect biotypes that break host plant resistance. We begin with an introduction to plant anatomy and physiology, then shift to a brief look at the structure of psyllid mouthparts. We finish with how EPG has advanced our understanding of the Asian citrus psyllid as a citrus pest. Again, though it was originally used to study primarily aphids, EPG is now applied more broadly, and more experiments use EPG, which makes a general understanding of the process useful to stakeholders.
Plant Anatomy: A Psyllid’s Viewpoint
When people eat a lettuce leaf, they experience the whole leaf. We might separate the midrib from the leafy part, but that is our level of detail. Small insects experience the leaf at a cellular level, more like a microscopist’s view.
The Asian citrus psyllid (Diaphorina citri) lands on a host plant and moves to the lower leaf surface. Next, the psyllid salivates at a suitable site and inserts its piercing-sucking mouthparts into the plant. Saliva seals the wound. The goal of the psyllid is to reach the phloem (a source of nutrition) or xylem (a source of water). Figure 1 is a skeletonized citrus leaf showing the xylem veins. The xylem transports water and minerals from the plant’s roots to its leaves and other plant parts. Phloem cells surround xylem cells in a living, healthy leaf. Sugars and other metabolites produced in leaves move through phloem cells to where they are needed elsewhere in the plant. Insects like aphids mostly ingest phloem sap. The psyllid is more flexible. It chooses phloem sap when feeding on young leaves, but xylem sap when it is on older leaves (Ebert et al. 2018). The difference in behavior is because female psyllids lay eggs exclusively on young leaves. Laying eggs consumes considerable energy. In the case of psyllids, the metabolic cost of reproduction is fueled by elevated nutrient levels in the phloem. Psyllid females do not lay eggs on mature leaves, so they feed on mature leaves only enough to survive.
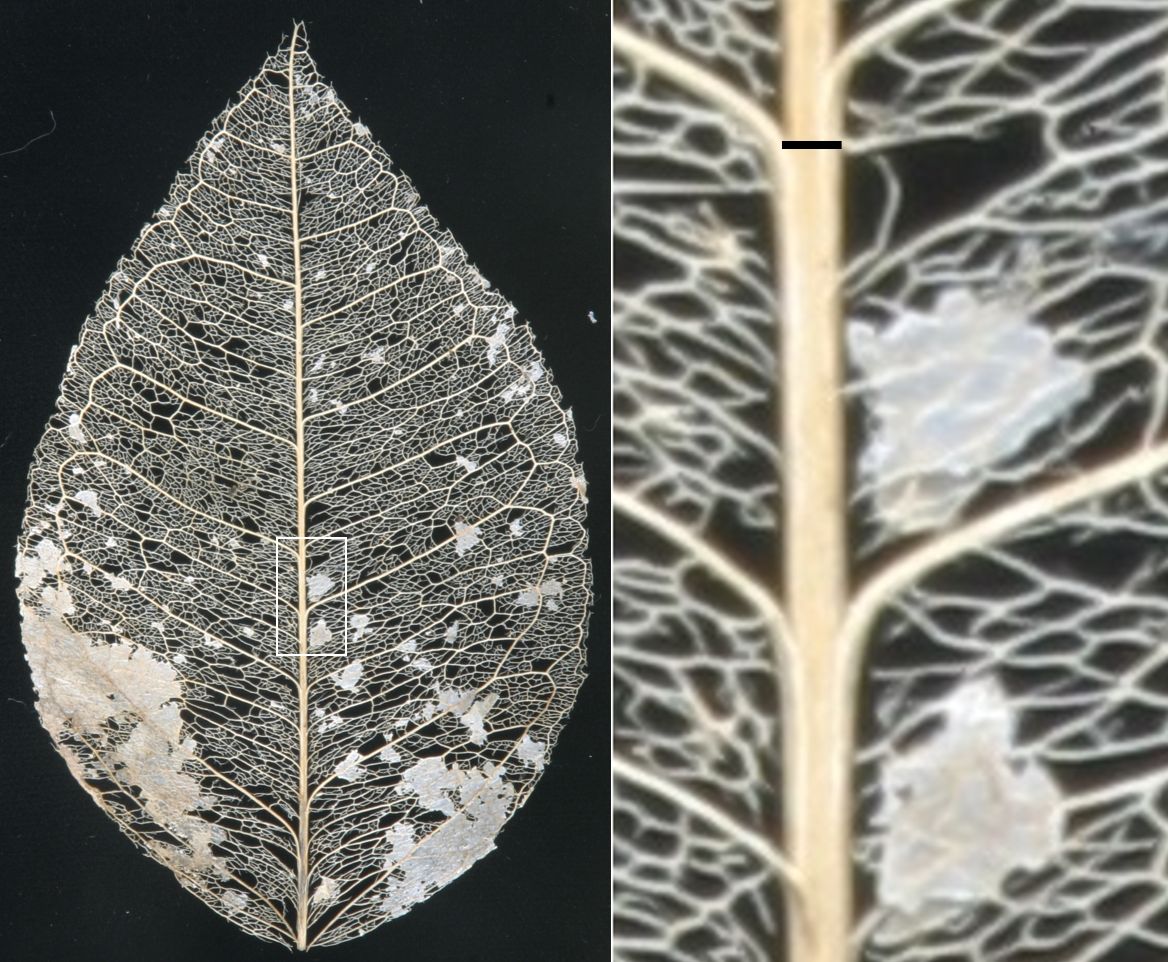
Credit: Timothy Ebert, UF/IFAS CREC
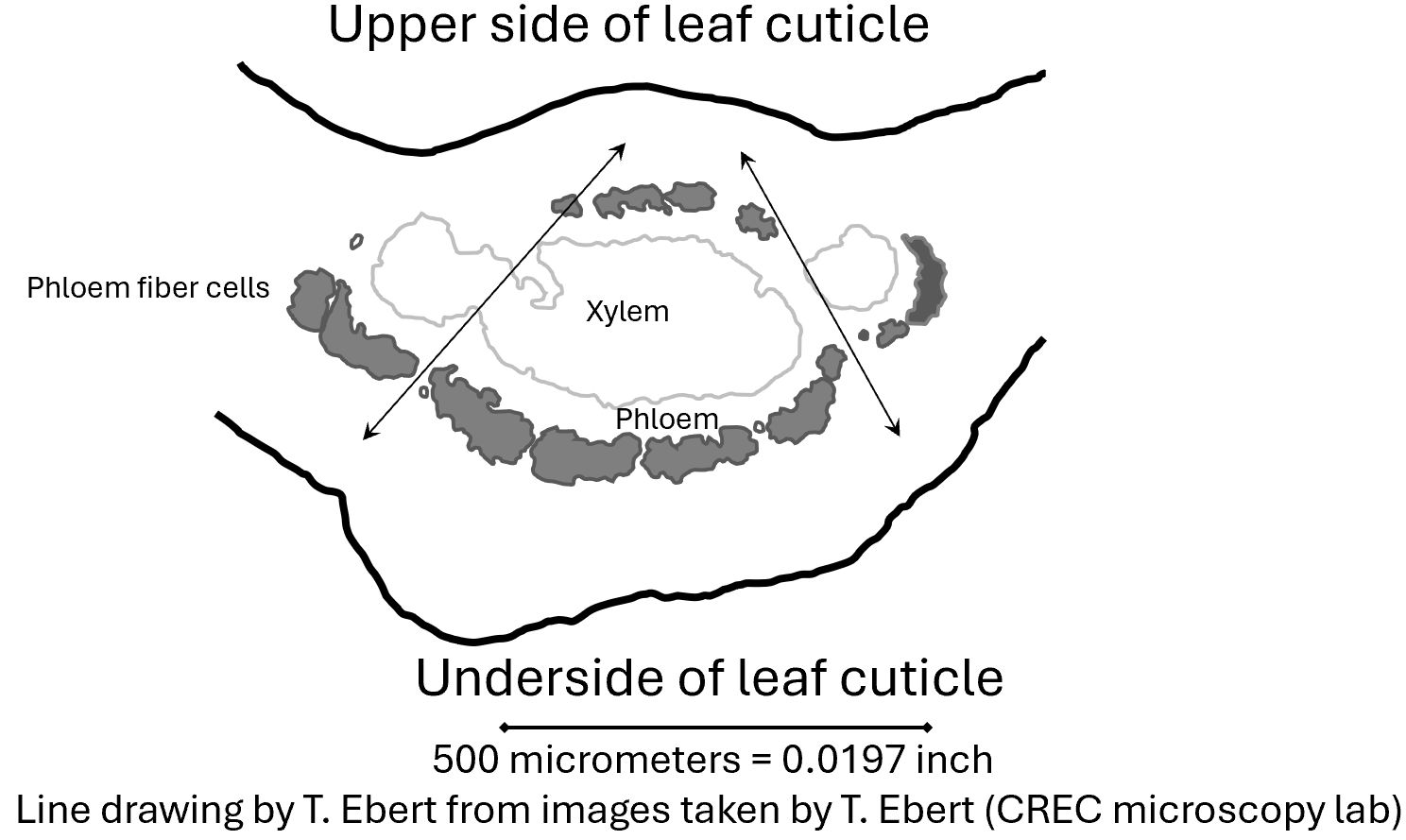
Credit: Timothy Ebert, UF/IFAS CREC
Figure 2 shows the phloem and xylem from two minor veins merging with the phloem and xylem of the central vein. The phloem fiber cells are a mechanical barrier forcing the psyllid to take time to find gaps or go around. Insect mouthparts find these gaps rather than go through the phloem fiber cells. The convergence of veins results in a wider gap between phloem fiber cells. This encourages the insects to feed where the veins come together.
The cells in a plant develop and change with age. Young tissue does not have the same structure as older tissue. There are fewer xylem cells, no mature phloem fiber cells, and phloem tissue is a relatively thick layer in the young leaf (Chernova et al. 2018; Ebert et al. 2018).
Plant Physiology: A Psyllid’s Viewpoint
Adult psyllids identify their host plants by sight and smell. Each plant has an aroma (Fancelli et al. 2018) that identifies the species and indicates the growth stage and health of the plant (Santos Silva et al. 2023). Female psyllids lay eggs at the tips of new growth (flush) (Cifuentes-Arenas et al. 2018). First-instar psyllids only survive on the youngest growth. Flush stops growing after a while, the meristem (growing point) dies, and the remaining leaves finish development and harden off (Cifuentes-Arenas et al. 2018). This maturation kills the younger immatures if they have not developed enough to survive on older tissues. It therefore benefits the psyllid adult to identify flushes that will last long enough for the offspring to complete development. Insects, like people, require many different nutrients to live. Psyllids must get everything they need, including water, minerals, vitamins, carbohydrates, fatty acids, and other essential nutrients, from their host plant (Hijaz and Killiny 2014).
Host plant quality changes with plants’ age and health and with the season. The irrigation and fertilization choices of growers also influence host plant quality by changing plant health. The psyllid must adapt to these changes or move to a new host. Moving to a new host is risky because it exposes the psyllid to predators and because the psyllid could dehydrate. Alternatively, the psyllid can compensate for reduced host quality by spending more time feeding on sap. However, plants have a variety of protective defenses against insects that include chemical defenses (Kessler and Kalske 2018) and resource reallocation (Schultz et al. 2013; Jiang et al. 2019). The psyllid must overcome more of these defenses when it ingests more sap.
Psyllid Mouthparts
The insect mouth is made up of several segments that are modified in different insect groups depending on how they feed (Nel et al. 2018). Each of the segments has a specialized name (AES 2024). In the Asian citrus psyllid, the maxilla and mandible form a straw-like tube for swallowing a liquid diet (Figure 3). The diameter of this straw is less than half the diameter of the finest human hair. The mouthparts are so small that they can pass between cells (intercellularly) but are strong enough to pass through cells (intracellularly) in search of phloem or xylem cells. In a psyllid, the mandible and maxilla combine to make the salivary canal, food canal, and two nerve canals.
Earlier we said that newly hatched immature psyllids only survive on new flush. One explanation is that the mouthparts of newly hatched immatures are neither hard enough nor long enough to reach the phloem on mature leaves. Mouthpart length in newly hatched immatures averages 266 µm, compared to 611 µm in adults (Ammar et al. 2013). Immature psyllid mouth parts are longer than the measured distance to phloem in mature leaves (Ammar et al. 2013), but they are nevertheless insufficiently long because psyllid mouthparts go around cells rather than taking the shortest path. In addition to this mechanical issue, the nutritional quality and chemical defenses near the growing point differ from elsewhere in the plant. Young immatures may take advantage of this difference. In any case, newly hatched insects only survive near the growing tip of the new flush. This is important in disease acquisition by the vector as discussed later.
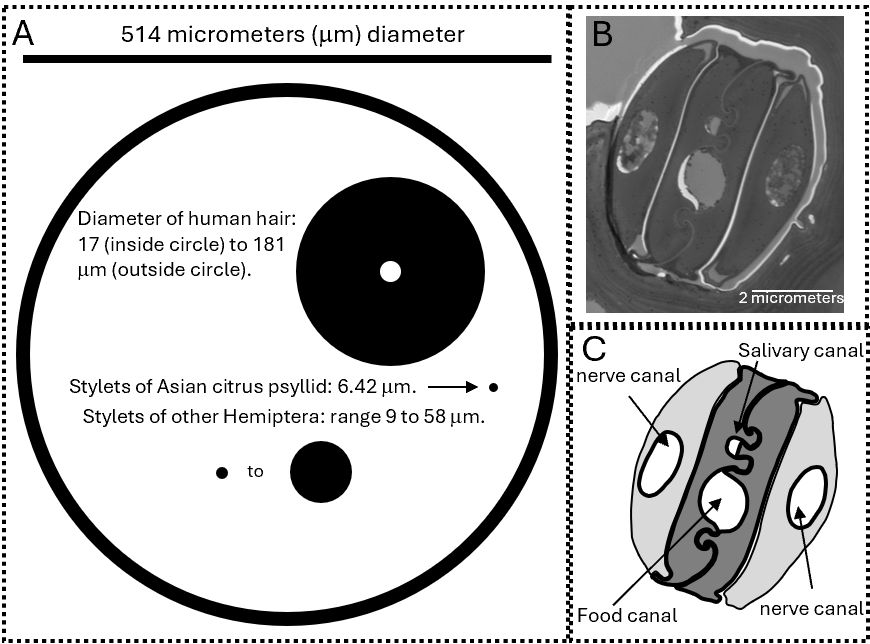
Credit: 3A and 3C: Drawings by Timothy Ebert, UF/IFAS CREC. 3B TEM image by Dianne Achor, UF/IFAS CREC microscopy lab
Electropenetrography
Before the development of EPG, the study of psyllid feeding behavior relied on observations of the aftereffects of that feeding. As they feed, psyllids create a salivary sheath. The salivary sheath remains in the plant for several weeks. These salivary tracks can be seen with a microscope, which makes it possible to identify where the insect’s mouthparts have been. However, preparation of such images kills the leaf and can only document what happened in the past. Electropenetrography, by contrast, identifies what the insect is doing in real-time, and it leaves both the plant and the insect unchanged.
Electropenetrography is a technique that makes the insect part of an electronic circuit. It subjects the insect to a gentle electrical current and records voltage changes across the insect. While a flashlight battery is 1.5 volts, EPG studies use a voltage between 0.025 and 0.15 volts. A computer records the voltage change across the insect and produces a graph (Figure 4). Initially, the insect is touching the plant only with its feet. A probe starts when the insect inserts its mouthparts into the plant. The insect forces the mouthparts through the plant (pathway phase, Figure 5). The pathway phase might end when mouthparts contact a xylem or phloem cell (Figures 6 and 7), or the insect might withdraw its mouthparts and move to a new location. Every behavior produces a unique pattern of changing voltages called a waveform. The waveform is diagnostic, in that each insect exhibiting that pattern is engaged in the same activity, at least within a species. A probe ends when the mouthparts leave the plant. Recordings get converted from voltages to the time spent in each type of behavior. Variables like “average duration of phloem salivation” or “time to first salivation into phloem” are calculated from these data (Ebert et al. 2015).
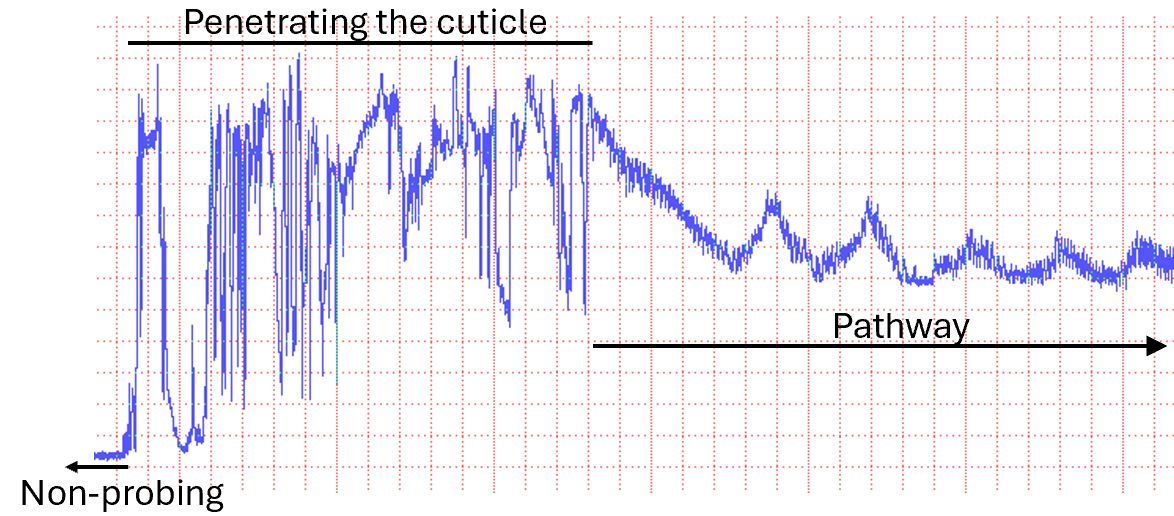
Credit: Timothy Ebert, UF/IFAS CREC
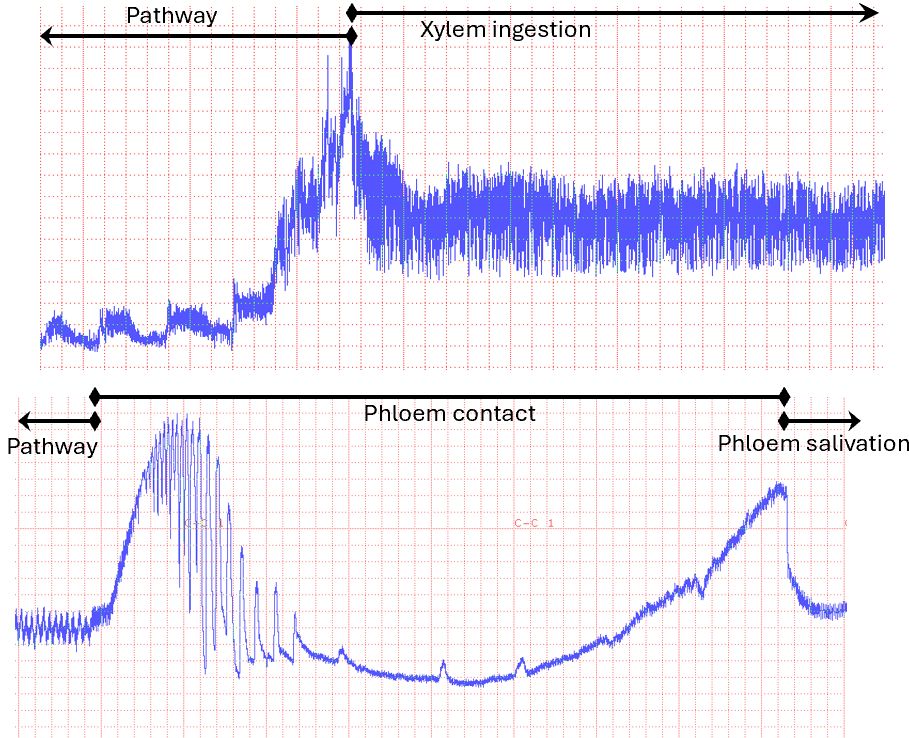
Credit: Timothy Ebert, UF/IFAS CREC
Initial experimental set-up can influence some EPG results. It takes time to attach a wire to an insect for EPG experiments. During this time, the insect is starving and dehydrating. Migration to a new host is a natural condition that produces similar circumstances. Dispersal rates of three days to move 100 yards (91 meters), four days to move 400 yards, and 12 days to travel 2200 yards (2 kilometers) have been recorded in field trials (Lewis-Rosenblum et al. 2015). A psyllid can fly continuously for about 3 hours and cover about 2600 yards (2.4 kilometers) on a laboratory flight mill (aerial treadmill) (Martini et al. 2014). Even longer distances are possible if insects are assisted by the wind or human movements. Extended starvation periods reduce the time the insect takes to start probing, but such probes are more likely to result in xylem feeding by the psyllid. Electropenetrography has recently shown that survival can be extended by utilization of non-host plants for emergency rations when a host plant is not available (George et al. 2020; George et al. 2022), though other approaches also confirm that utilization of non-hosts improves survival (Zhang et al. 2019; Pec et al. 2023).
There is a concern that handling the insect, attaching a wire, and passing a current through the insect alters the insect’s behavior. The key is to realize that not all arthropods are the same, and no single EPG method will work for all arthropods. Smaller insects require smaller wires for attachment, as shown for whiteflies (Lei et al. 2009) and further refined by Chesnais and Mauck (2018). Some insects can feel the electrical current and respond to it. Eliminating this response is done by reducing the voltage of the applied signal or by using alternating current (AC) rather than direct current (DC) (Cervantes and Backus 2015, 2018). A variety of methods and tools for handling insects, ranging from suction devices for holding the insect to chill plates or carbon dioxide for incapacitating the insect, make wiring insects easier. Laboratory conditions like temperature and lighting can also influence outcomes (Son et al. 2012). The point is that there are conditions where EPG can cause behavioral changes in insects, and the solution to that problem is to adjust the methods to eliminate the unwanted behavioral effect (Ebert and Rogers 2016).
Insect Herbivore and Vector Biology
Different groups of plant-feeding Hemiptera have different approaches to getting a meal. Some, like aphids, prefer phloem sap and largely ignore xylem, and others, like sharpshooters, mainly consume xylem sap. Insects like the Asian citrus psyllid change behavior depending on where they are: the time spent phloem-feeding is increased on young tissue, and the time spent xylem-feeding is increased when they are on mature leaves (Ebert et al. 2018). For vectors, the preferred cell type must match the pathogen’s lifestyle. The agent that causes citrus greening disease lives exclusively in the phloem and requires a vector that feeds on the phloem. Therefore, the disease cycle is less likely to be completed when psyllids are on mature leaves. The Asian citrus psyllid requires young tissues to complete its life cycle (Cifuentes-Arenas et al. 2018), and the psyllid is mostly a phloem feeder on immature plant tissues (Ebert et al. 2018). Thus, it is more likely that the disease cycle is completed when psyllids are on young leaves.
Citrus Greening Disease
Candidatus Liberibacter asiaticus (CLas) is the currently accepted causal agent for citrus greening. Candidatus Liberibacter asiaticus is a bacterium restricted to living in the phloem. In Florida, the Asian citrus psyllid is the sole vector of CLas. The insect acquires CLas while ingesting phloem and transmits CLas during salivation. Once swallowed, CLas eventually passes through the gut wall, moves through the blood, and enters the salivary glands. When the insect salivates, bacterial cells pass down the salivary canal and into the plant.
As with many plant and animal diseases (Van Damme et al. 2021), the number of bacterial cells that move from the psyllid into the citrus plant influences the success rate of disease transmission. One of our knowledge gaps is the nature of this relationship in citrus. What is the probability that the citrus tree will become infected if one, two, three, or more bacterial cells move from the vector to the host? How many bacterial cells per minute pass into the plant during salivation? Likewise, the number of ingested bacterial cells influences acquisition by the psyllid. The main point is that the movement of the pathogen in this system is a set of probabilities. Increasing the time spent salivating or ingesting phloem increases the chance of a successful infection. Increasing the number of vectors will also have this effect.
Asian Citrus Psyllid
The Asian citrus psyllid (Diaphorina citri) can reproduce on several hosts in the plant family Rutaceae (e.g., Citrus spp., Bergera koenigii, Murraya paniculata). Many other hosts are listed (CDFA 2024), and not all are in the Rutaceae family (Stelinski 2019). Some of these hosts will support a higher population growth rate than others. Furthermore, CLas will not survive on some hosts (e.g., Murraya spp.). An infected psyllid may lose the ability to vector CLas while feeding on hosts where CLas cannot survive. Moving to an infected host would allow the psyllid to reacquire CLas (Ramsey et al. 2022). However, adult psyllid feeding behavior changes depending on host plant species (Shugart 2019), tissue age (Ebert et al. 2018), and other factors (Tao et al. 2023). Changes in feeding patterns influence how efficiently the vector acquires and transmits the pathogen.
Adult Asian citrus psyllids spend more time feeding on phloem when they are on immature leaves (32.0%) versus mature leaves (18.5%) (values from 'Midsweet' orange scion on 'Kuharske citrange' rootstock). From the beginning of a recording, adult psyllids took 10.7 hours to start salivating into the phloem on new flush, whereas they took up to 15.5 hours on mature leaves. The average duration of a salivation event was 1.7 minutes on flush and 0.4 minutes on mature leaves. Thus, adults should be more effective vectors of CLas on new growth versus when they are on mature leaves. Adults should be better able to acquire CLas when on new growth. The adult spent an average of 2.2 hours on immature leaves versus 1.3 hours on mature leaves per phloem ingestion event (Ebert et al. 2018). Because there is an expected difference between inoculation and acquisition on flush and inoculation and acquisition on mature leaves, there should be a seasonal component to infection. The disease should spread more rapidly through a grove when flush is abundant. Relative to adults, immatures acquire CLas more easily (Pelz-Stelinski et al. 2010). One reason is that immatures spend about 79% of their time feeding on phloem sap compared to 24% in adults (George et al. 2018). Therefore, the risk of spreading CLas increases during periods of citrus growth. However, CLas promotes off-season flushing, and management practices may also have this effect. The result is that scouting is always necessary to detect psyllid outbreaks.
In a grove with thousands of psyllids, the average time to salivate into the phloem is not particularly relevant if the goal is to prevent disease. The minimum time is a more relevant measure. From a sample of 720 insects on young flush, it took 13.2 minutes for the first insect to begin salivating into the phloem, and 2.9% of the insects reached the phloem within the first hour (data from Ebert and Rogers 2016). The duration of this earliest event was 1.02 minutes. The shortest time to begin ingestion was 13.5 minutes. While the average psyllid takes a long time to initiate behaviors relevant to disease inoculation and acquisition, a few individuals accomplish these tasks quickly. The probability of successful infection with a 1.02-minute salivation event is unknown. However, if the goal is disease prevention, even one success is too many.
Insecticides
Insecticides are used in vector management to limit the rate of disease spread. In some cases, the goal is to stop the disease from spreading. For prevention, the insecticide must kill or repel the vector before any vector can engage in the inoculation behavior. For the psyllid-CLas system, that behavior is salivating into the phloem. Analytical methods do not yet exist to measure pesticide concentrations at a cellular level. However, phloem salivation always occurs before phloem feeding. We used electropenetrography to estimate the time to the first feeding event with pesticide-spiked artificial diets. With an imidacloprid spiked diet, 64% of the psyllids ingested diet at 55 ppm. At 550 ppm, 4% of psyllids ingested diet (Langdon et al. 2019). Admire Pro (imidacloprid) applied at label rates only reached 44.8 to 194.4 ppm. Therefore, imidacloprid at label rates will not stop the spread of CLas. However, the insecticide will reduce the rate of disease spread by reducing vector abundance. Other insecticides were not tested.
Typically, insecticides are developed to kill targeted pests. However, a few may act in other ways. For example, kaolin gets stuck on the tarsal claws (essentially the psyllid’s feet). This reduces the ability of the insect to hold onto the plant. An EPG study with kaolin as a treatment showed fewer probes from insects on treated plants. Only one out of 15 insects salivated into the phloem, but that one did not ingest phloem (Kim 2013).
An alternative to insecticides for vector management is mechanical exclusion. Exclusion takes two forms in commercial citrus production. Growers can build screened enclosures covering several acres (CUPS). Alternatively, growers can cover individual trees with a screen bag (IPC). With IPCs, the tree grows to fill the bag. As the bag fills, the leaves become tightly appressed to the screen. Electropenetrography methods could determine whether psyllids are able to feed on the plant through the screen.
Non-Hosts
A host is a plant on which an insect can complete its life cycle. A non-host would not support reproduction but could provide water or have some nutritional value (Zhang et al. 2019; Guz et al. 2020). Recent advances allow the detection of non-host plant DNA in the gut contents of insects. Surprisingly, some insects use a variety of non-host plants. It is suggested that these are emergency rations in times of stress (Cooper et al. 2019). Electropenetrography is one approach for evaluating the quality of different plants for an insect’s feeding. Electropenetrography results could rank non-hosts based on the level of physiological stress the insect will endure before resorting to feeding on a non-host plant. One challenge is identifying all of a given insect’s potential emergency ration hosts. Another as-yet-unanswered question for many non-host species and insect vector species interactions is how long a given insect can survive on such hosts (Zhang et al. 2019).
Non-host plant use by vectors can have several effects relevant to pest management. Non-hosts can supply a different balance of nutrients to result in a more nutritionally complete diet. Better diets would increase survival and reproduction on host plants. Non-hosts may boost survival and reproduction by providing manageable (sublethal) stress (Hoffmann 2009; Chen et al. 2020; Cutler et al. 2022). Both the metabolism of toxins and increased reproduction require metabolic energy. This requirement is measurable using EPG (Wang et al. 2023). In addition to a reproductive effect, the ability to use non-hosts influences insect dispersal. Non-hosts may provide emergency rations for surviving a migration between geographically distant hosts (Stelinski 2019). Finally, non-host utilization is a pathway to broaden the host range of a plant pathogen. As Ristaino et al. point out, “plant pathogens may shift hosts and gain the ability to infect new hosts when introduced into new regions” (2021). The cassava mosaic virus in Africa is one example.
An insect vector’s ability to use non-host plants carries other risks to agricultural systems. Non-host use could put different pathogens in the same host and enable hybridization between two pathogens. Phytophthora andina is a hybridized pathogen, though it is not transmitted by a vector (Goss et al. 2011). The beetle-vectored Dutch elm disease bacterium is another example of hybridization (Brasier 2001). Another agricultural risk is that the pathogen could move to a new vector. The Pierce’s disease bacterium, for example, acquired a new vector when it was introduced into Taiwan (Backus and Shih 2020). These examples show that plant pathogens can change hosts and vectors to invade new areas. The ability of a vector to sample non-hosts provides opportunities for these transitions to take place.
Within host and non-host plants, there is a continuous range of quality for supporting insect survival. A few plant species may be optimal hosts and supply all the insect’s requirements. Other plants may only avert an insect’s death from dehydration by a few hours or not at all. Experiments measuring reproductive output are essential in understanding the first part of this range of quality. However, EPG experiments cover the entire range. Understanding non-host utilization is useful in understanding migration where host plants are widely dispersed. Electropenetrography is ideally suited to this task because it can identify changes in behavior related to differences in host quality. For example, behavioral differences between apparently suitable hosts (Shugart et al. 2019). New EPG technologies are expanding the breadth of applications for EPG. This growing field assists the development of novel means of pest management and provides a better understanding of current approaches.
Summary
Feeding behaviors of insects with piercing-sucking mouthparts are not directly observable during feeding because insect mouthparts are hidden by the opaque tissues of the plant or animal host. Electropenetrography (EPG) solves this problem by making the insect a part of an electronic circuit. Repetitive patterns in voltage changes (waveforms) are characteristic of specific behaviors, recorded in real time. Because EPG is not destructive, after EPG, the insect and host can be used in further experiments to understand long-term effects of treatments on survival and reproduction. We used the Asian citrus psyllid on citrus as an example of how improved knowledge about vector biology via EPG could improve management outcomes. For example, insecticides reduce vector populations, but they can be less effective than expected. Through EPG research, we now understand that imidacloprid does not act quickly enough at label rates to be effective at disease prevention. Infected psyllids have enough time to infect new trees before they die. Imidacloprid reduces the rate of disease spread in the environment but cannot stop the spread. Electropenetrography identified this problem, and it can also help find a solution to this problem by explaining psyllid-plant interactions. Electropenetrography provided details about feeding of immature versus adult psyllids in relation to locations within the plant (phloem versus xylem) to identify vulnerabilities in biology that can be exploited to breed resistant varieties of citrus. Electropenetrography has also aided important research on psyllid ecology. Vector dispersal is essential in the spread of a disease like citrus greening, yet the Asian citrus psyllid can only live a few days without water. Electropenetrography and gut content analyses have shown that some insects can get water and nutrients from non-host plants, thereby increasing the dispersal range of the psyllid and the bacterium it carries. Non-host use provides opportunities for the bacterium to interact with other pathogens and potential new vectors, stimulating evolution of new bacterial variants and new vectors. Understanding this process is aiding in the development of management strategies.
Selected References
(all links accessed 08-08-2024)
AES. 2024. "Insect Mouthparts." https://www.amentsoc.org/insects/fact-files/mouthparts.html
Ammar, E.-D., D. G. Hall, and R. G. Shatters, Jr. 2013. “Stylet Morphometrics and Citrus Leaf Vein Structure in Relation to Feeding Behavior of the Asian Citrus Psyllid Diaphorina citri, Vector of Citrus Huanglongbing Bacterium.” Plos One. https://doi.org/10.1371/journal.pone.0059914
Backus, E. A., and H. T. Shih. 2020. “Review of the EPG Waveforms of Sharpshooters and Spittlebugs Including Their Biological Meanings in Relation to Transmission of Xylella fastidiosa (Xanthomonadales: Xanthomonadaceae).” Journal of Insect Science 20(4). https://doi.org/10.1093/jisesa/ieaa055
Brasier, C. M. 2001. “Rapid Evolution of Introduced Plant Pathogens via Interspecific Hybridization.” Bioscience 51:123–133. https://doi.org/10.1641/0006-3568(2001)051[0123:REOIPP]2.0.CO;2
CDC. 2020. Vaccine Administration: Needle Gauge and Length. https://www.cdc.gov/vaccines/hcp/admin/downloads/vaccine-administration-needle-length.pdf
CDFA. 2024. Asian Citrus Psyllid Host List. https://www.cdfa.ca.gov/plant/pdep/target_pest_disease_profiles/hostlists/AsianCitrusPsyllid-HostList.pdf
Cervantes, F. A., and E. A. Backus. 2015. “Amount of applied signal voltage affects behaviors in EPG recordings of Lygus lineolaris adults on pinhead cotton squares.” Annual Meeting of the Entomological Society of America. Entomological Society of America, Minneapolis, MN. Nov 2015.
Cervantes, F. A., and E. A. Backus. 2018. “EPG Waveform Library for Graphocephala atropunctata (Hemiptera: Cicadellidae): Effect of Adhesive, Input Resistor, And Voltage Levels on Waveform Appearance and Stylet Probing Behaviors.” Journal of Insect Physiology 109:21–40. https://doi.org/10.1016/j.jinsphys.2018.05.008
Chen, X. D., M. Seo, T. A. Ebert, M. Ashfaq, W. Qin, and L. L. Stelinski. 2020. “Hormesis in the Brown Citrus Aphid, Toxoptera citricida (Kirkaldy) (Hemiptera: Aphididiae) Exposed to Sublethal Doses of Imidacloprid.” Florida Entomologist 103: 337–343. https://doi.org/10.1653/024.103.0305
Chernova, T. E., P. V. Mikshina, V. V. Salnikov, N. N. Ibragimova, O. V. Sautkina, and T. A. Gorshkova. 2018. “Development of Distinct Cell Wall Layers Both in Primary and Secondary Phloem Fibers of Hemp (Cannabis sativa L.).” Industrial Crops and Products 117: 97–109. https://doi.org/10.1016/j.indcrop.2018.02.082
Chesnais, Q., and K. E. Mauck. 2018. “Choice of Tethering Material Influences the Magnitude and Significance of Treatment Effects in Whitefly Electrical Penetration Graph Recordings.” Journal of Insect Behavior 31:656–671. https://doi.org/10.1007/s10905-018-9705-x
Cifuentes-Arenas, J. C., A. de Goes, M. P. de Miranda, G. A. C. Beattie, and S. A. Lopes. 2018. “Citrus Flush Shoot Ontogeny Modulates Biotic Potential of Diaphorina citri.” Plos One 13:1–17. https://doi.org/10.1371/journal.pone.0190563
Cooper, W. R., D. R. Horton, M. R. Wildung, A. S. Jensen, J. Thinakaran, D. Rendon, L. B. Nottingham, et al. 2019. “Host and non-host 'whistle stops' for psyllids: molecular gut content analysis by high-throughput sequencing reveals landscape-level movements of Psylloidea (Hemiptera).” Environmental Entomology 48:554–566. https://doi.org/10.1093/ee/nvz038
Cutler, G. C., M. Amichot, G. Benelli, R. N. C. Guedes, Y. Qu, R. R. Rix, F. Ullah, and N. Desneux. 2022. “Hormesis and Insects: Effects and Interactions in Agroecosystems.” Science of the Total Environment 825:153899. https://doi.org/10.1016/j.scitotenv.2022.153899
Ebert, T. A., and M. E. Rogers. 2016. “Effect of Substrate Voltage on EPG Recordings of Ingestion and Probing Behavior in Diaphorina citri (Hemiptera: Liviidae).” Florida Entomologist 99:528–534. https://doi.org/10.1653/024.099.0328
Ebert, T. A., E. A. Backus, H. J. Shugart, and M. E. Rogers. 2018. “Behavioral plasticity in probing by Diaphorina citri (Hemiptera, Liviidae): ingestion from phloem versus xylem is influenced by leaf age and surface.” Journal of Insect Behavior 31:119–137. https://doi.org/10.1007/s10905-018- 9666-0
Ebert, T. A., E. Backus, M. Cid, A. Fereres, and M. Rogers. 2015. “A New SAS Program for Behavioral Analysis of Electrical Penetration Graph Data.” Computers and Electronics in Agriculture 116:80–87. https://doi.org/10.1016/j.compag.2015.06.011
Fancelli, M., M. Borges, R. A. Laumann, J. A. Pickett, M. A. Birkett, and M. C. Blassioli-Moraes. 2018. “Attractiveness of host plant volatile extracts to the Asian citrus psyllid, Diaphorina citri, is reduced by terpenoids from the non-host cashew.” Journal of Chemical Ecology 44:397–405. https://doi.org/10.1007/s10886-018-0937-1
George, J., E. D. Ammar, D. G. Hall, R. G. Shatters, Jr., and S. L. Lapointe. 2018. “Prolonged phloem ingestion by Diaphorina citri nymphs compared to adults is correlated with increased acquisition of citrus greening pathogen.” Scientific Reports 8:10352. https://doi.org/10.1038/s41598-018-28442-6
George, J., R. Kanissery, M. Bashyal, B. Tamayo, and L. L. Stelinski. 2022. “Survival and Feeding Behavior of Diaphorina citri (Hemiptera: Liviidae) Adults on Common Cover Crops in Citrus.” Agriculture 12. https://doi.org/10.3390/agriculture12122175
George, J., R. Kanissery, E.-D. Ammar, I. Cabral, L. T. Markle, J. M. Patt, and L. L. Stelinski. 2020. “Feeding Behavior of Asian Citrus Psyllid [Diaphorina citri (Hemiptera: Liviidae)] Nymphs and Adults on Common Weeds Occurring in Cultivated Citrus Described Using Electrical Penetration Graph Recordings.” Insects 11:48. https://doi.org/10.3390/insects11010048
Goss, E. M., M. E. Cardenas, K. Myers, G. A. Forbes, W. E. Fry, S. Restrepo, and N. J. Grunwald. 2011. “The Plant Pathogen Phytophthora andina Emerged via Hybridization of an Unknown Phytophthora Species and the Irish Potato Famine Pathogen, P. infestans.” Plos One 6:e24543. https://doi.org/10.1371/journal.pone.0024543
Guz, N., M. Arshad, N. S. Cagatay, A. Dageri, and M. I. Ullah. 2020. “Detection of Wolbachia (Rickettsiales: Anaplasmataceae) and Candidatus Liberibacter asiaticus (Rhizobiales: Rhizobiaceae) Associated with Diaphorina citri (Hemiptera: Liviidae) Collected from Citrus reticulata (Sapindales: Rutaceae) and Alternate Host, Cordia myxa (Boraginales: Boraginaceae).” Journal of Economic Entomology 113:1486–1492. https://doi.org/10.1093/jee/toaa043
Hijaz, F., and N. Killiny. 2014. “Collection and Chemical Composition of Phloem Sap from Citrus sinensis L. Osbeck (Sweet Orange).” Plos One 9:1–11. https://doi.org/10.1371/journal.pone.0101830
Hoffmann, G. R. 2009. “A Perspective on the Scientific, Philosophical, and Policy Dimensions of Hormesis.” Dose Response 7:1–51. https://doi.org/10.2203/dose-response.08-023.Hoffmann
Jiang, Y., C. X. Zhang, R. Chen, and S. Y. He. 2019. “Challenging Battles of Plants with Phloem-Feeding Insects and Prokaryotic Pathogens.” Proceedings of the National Academy of Science U S A 116: 23390–23397. https://doi.org/10.1073/pnas.1915396116
Kessler, A., and A. Kalske. 2018. “Plant Secondary Metabolite Diversity and Species Interactions.” Annual Review of Ecology, Evolution, and Systematics 49:115–138. https://doi.org/10.1146/annurev-ecolsys-110617-062406
Kim, K. D. 2013. “Integrated Management of Asian Citrus Psyllid, Diaphorina citri Kuwayama, for Protecting Young Citrus Trees from Huanglongbing.” PhD diss., University of Florida. https://ufdcimages.uflib.ufl.edu/UF/E0/04/62/49/00001/KIM_K.pdf
Langdon, K. W., T. A. Ebert, and M. E. Rogers. 2019. “Evaluating the Effect of Imidacloprid Administered in Artificial Diet on Feeding Behavior of Diaphorina citri (Hemiptera: Liviidae) Using Electropenetrography.” Journal of Economic Entomology 112:644–652. https://doi.org/10.1093/jee/toy400
Lei, H., W. F. Tjallingii, and J. C. van Lenteren. 2009. “Effect of Tethering during EPG Recorded Probing by Adults of the Greenhouse Whitefly.” Journal of Applied Entomology 121:211–217. https://doi.org/10.1111/j.1439-0418.1997.tb01395.x
Lewis-Rosenblum, H., X. Martini, S. Tiwari, and L. L. Stelinski. 2015. “Seasonal Movement Patterns and Long-Range Dispersal of Asian Citrus Psyllid in Florida Citrus.” Journal of Economic Entomology 108:3–10. https://doi.org/10.1093/jee/tou008
Martini, X., A. Hoyte, and L. L. Stelinski. 2014. “Abdominal color of the Asian citrus psyllid (Hemiptera: Liviidae) is associated with flight capabilities.” Annals of the Entomological Society of America 107:842–847. https://doi.org/10.1603/AN14028
Nel, P., S. Bertrand, and A. Nel. 2018. “Diversification of Insects since the Devonian: A New Approach Based on Morphological Disparity of Mouthparts.” Scientific Reports 8:3516. https://doi.org/10.1038/s41598-018-21938-1
Pec, M., E. A. Ferreira, and M. Penaflor. 2023. “Association of Non-Host Crop Plants with Mandarin in Host Location and Survival of Diaphorina citri Kuwayama (Hemiptera: Psyllidae).” Neotropical Entomology 53:304–313. https://doi.org/10.1007/s13744-023-01107-4
Pelz-Stelinski, K. S., R. H. Brlansky, T. A. Ebert, and M. E. Rogers. 2010. “Transmission Parameters for Candidatus Liberibacter asiaticus by Asian Citrus Psyllid (Hemiptera: Psyllidae).” Journal of Economic Entomology 103:1531–1541. https://doi.org/10.1603/EC10123
Ramsey, J. S., E. D. Ammar, J. E. Mahoney, K. Rivera, R. Johnson, D. O. Igwe, T. W. Thannhauser, M. J. MacCoss, D. G. Hall, and M. Heck. 2022. “Host plant adaptation drives changes in Diaphorina citri proteome regulation, proteoform expression, and transmission of ‘Candidatus Liberibacter asiaticus’, the citrus greening pathogen.” Phytopathology. 112:101–115. https://doi.org/10.1094/PHYTO-06-21-0275-R
Ristaino, J. B., P. K. Anderson, D. P. Bebber, K. A. Brauman, N. J. Cunniffe, N. V. Fedoroff, C. Finegold, et al. 2021. “The Persistent Threat of Emerging Plant Disease Pandemics to Global Food Security.” Proceedings of the National Academy of Science U S A 118 (23): e2022239118. https://doi.org/10.1073/pnas.2022239118
Santos Silva, M., J. M. Patt, C. de Jesus Barbosa, M. Fancelli, P. Roberto Ribeiro Mesquita, F. de Medeiros Rodrigues, and A. Selbach Schnadelbach. 2023. “Asian Citrus Psyllid, Diaphorina citri (Hemiptera: Liviidae) Responses to Plant-Associated Volatile Organic Compounds: A Mini-Review.” Crop Protection 169:106242. https://doi.org/10.1016/j.cropro.2023.106242
Schultz, J. C., H. M. Appel, A. P. Ferrieri, and T. M. Arnold. 2013. “Flexible Resource Allocation during Plant Defense Responses.” Frontiers in Plant Science 4:324. https://doi.org/10.3389/fpls.2013.00324
Shugart, H. 2019. “Probing Behavior and Host Preference in the Asian Citrus Psyllid, Diaphorina citri (Hemiptera: Liviidae).” PhD diss. University of Florida. https://original-ufdc.uflib.ufl.edu/UFE0054047/00001
Shugart, H., T. Ebert, F. Gmitter, and M. Rogers. 2019. “The Power of Electropenetrography in Enhancing Our Understanding of Host Plant-Vector Interactions.” Insects 10:1–22. https://doi.org/10.3390/insects10110407
Son, Y., E. A. Backus, R. L. Groves, and M. W. Johnson. 2012. “Pattern of Stylet Penetration Activity by Homalodisca vitripennis (Hemiptera: Cicadellidae) Adults in Relation to Environmental Temperature and Light Conditions.” Environmental Entomology 41:1215–1230. https://doi.org/10.1603/EN11319
Stelinski, L. L. 2019. “Ecological Aspects of the Vector-Borne Bacterial Disease, Citrus Greening (Huanglongbing): Dispersal and Host Use by Asian Citrus Psyllid, Diaphorina citri Kuwayama.” Insects 10:1–11. https://doi.org/10.3390/insects10070208
Tao, T., Z. Wang, R. Mao, M. Hussain, S. P. Arthurs, F. Ye, X. An, and J. Gao. 2023. “Vermicompost amendments disrupt feeding behavior of Diaphorina citri Kuwayama and boost activities of salicylic acid and jasmonic acid pathway-related enzymes in citrus.” Insects 14 (5): 410. https://doi.org/10.3390/insects14050410
Van Damme, W., R. Dahake, R. van de Pas, G. Vanham, and Y. Assefa. 2021. “COVID-19: Does the infectious inoculum dose-response relationship contribute to understanding geterogeneity in disease severity and transmission dynamics?” Medical Hypotheses 146:110431. https://doi.org/10.1016/j.mehy.2020.110431
Wang, L., J. Zhu, Q. Wang, X. Ji, W. Wang, W. Huang, C. Rui, and L. Cui. 2023. “Hormesis Effects of Sulfoxaflor on Aphis gossypii Feeding, Growth, Reproduction Behaviour and the Related Mechanisms.” Science of the Total Environment 872:162240. https://doi.org/10.1016/j.scitotenv.2023.162240
Zhang, R. M., S. Y. He, W. Wu, Y. J. Huang, C. Y. Zhu, F. Xiao, Z. Y. Liu, and J. W. Zeng. 2019. “Survival and Lifespan of Diaphorina citri on Non-Host Plants at Various Temperatures.” Crop Protection 124:104841. https://doi.org/10.1016/j.cropro.2019.06.001
Review Articles on EPG for More Information
“AC–DC Electropenetrography: Fundamentals, Controversies, and Perspectives for Arthropod Pest Management” https://doi.org/10.1002/ps.6087
“Review of the EPG Waveforms of Sharpshooters and Spittlebugs Including Their Biological Meanings in Relation to Transmission of Xylella fastidiosa (Xanthomonadales: Xanthomonadaceae)” https://doi.org/10.1093/jisesa
“AC–DC Electropenetrography for In-depth Studies of Feeding and Oviposition Behaviors” https://doi.org/10.1093/aesa/saz009
An Online Workshop on Electropenetrography
Methods and Science https://crec.ifas.ufl.edu/extension/epg/