Nematode Management in Organic Agriculture
The objectives of this publication are to provide information on plant-parasitic nematodes causing damage in organic agriculture and to introduce methods for their management. The intended audience is agricultural professionals and anyone producing agricultural products organically.
Nematodes are usually microscopic. They are classified as unsegmented worms, belonging to the Phylum Nematoda (Figure 1). Plant-parasitic nematodes are a concern for growers of agricultural or garden crops. These plant-parasitic nematodes will mainly feed on the roots of plants. A few kinds will feed on foliage, but this is not common. Many other kinds of nematodes are present in the soil as well. These include decomposers, predators, insect parasites, and animal parasites. Some nematodes are aquatic and do not affect terrestrial plants. Other nematodes act as decomposers, predators, and insect parasites. In farming systems, nematode predators and parasites of insects are beneficial, while nematode parasites of animals and plants are considered pests in agriculture. Beneficial nematodes that feed on either bacteria or fungi help in nutrient cycling by accelerating the decomposition of organic matter (Figure 2). Predatory nematodes may keep harmful nematodes at lower levels by feeding on them. Entomopathogenic nematodes (nematodes harmful to insects) may help to reduce numbers of some insect pests by infecting them with bacteria.
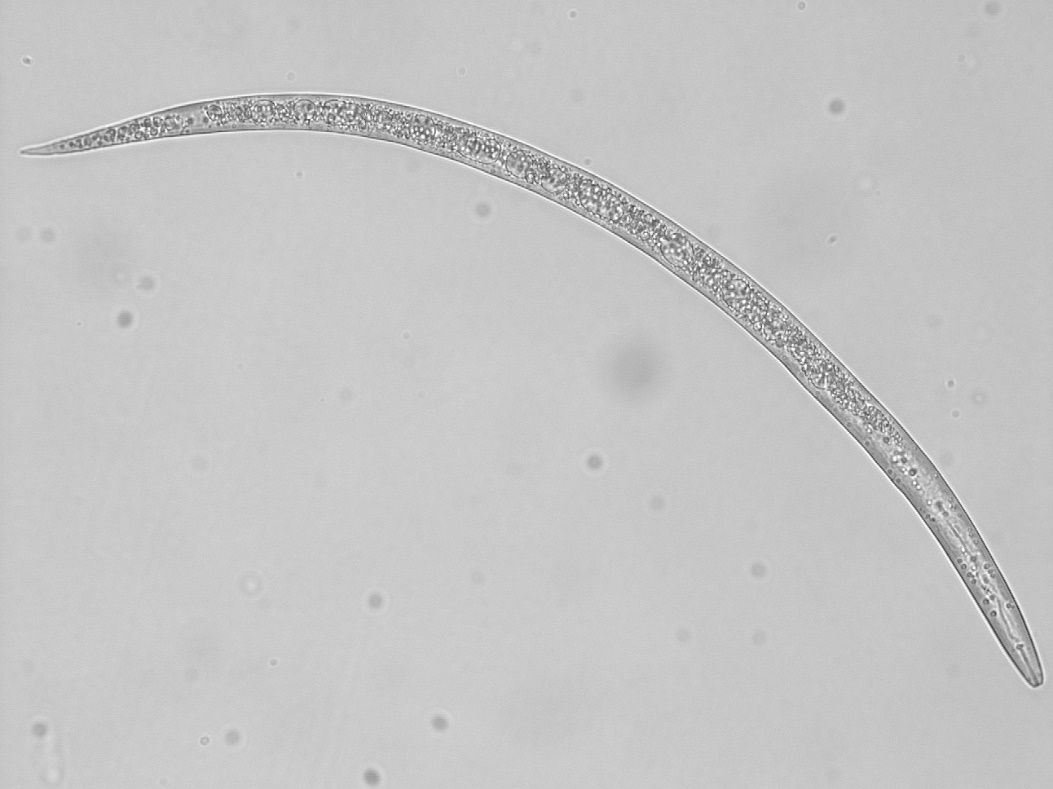
Credit: Zane Grabau UF/IFAS
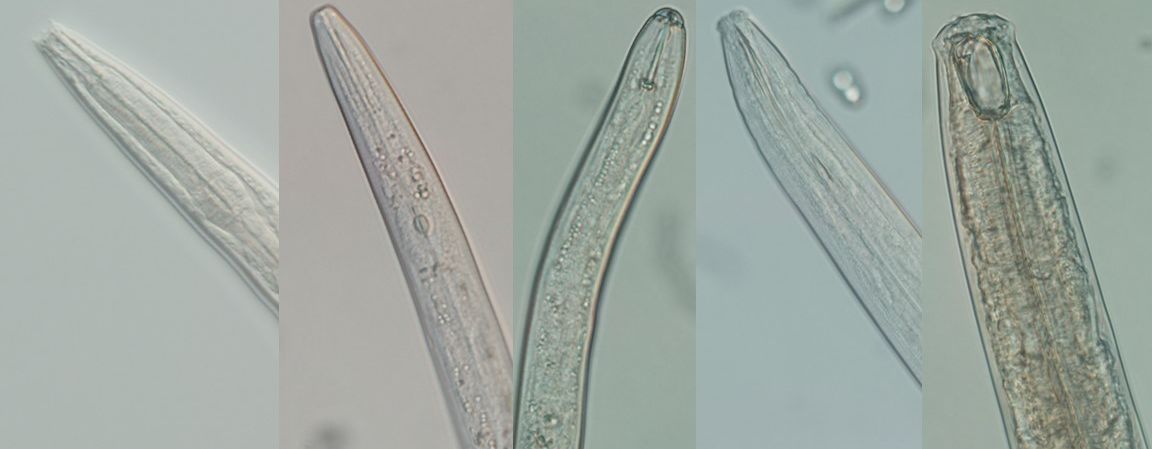
Credit: Zane Grabau UF/IFAS
Nematode Damage and Detection
Since most plant-parasitic nematodes feed on plant roots, symptoms are comparable to nutrient or water deficiency. These can be yield loss, stunting (Figure 3), yellowing, wilting, symptoms of nutrient deficiency, and malformations of the root system caused by direct feeding damage. In addition, invasion by plant-parasitic nematodes often provides an infection route for other organisms such as bacteria or fungi since nematode activity creates an entryway into the root that would otherwise not be available. Nematode reproduction is very fast. On average, a typical life cycle may be only 30 days at summer temperatures. This means that even if nematode numbers are low at the beginning of the growing season, nematode populations can rapidly increase and can become harmful to the crop in a relatively short period of time.
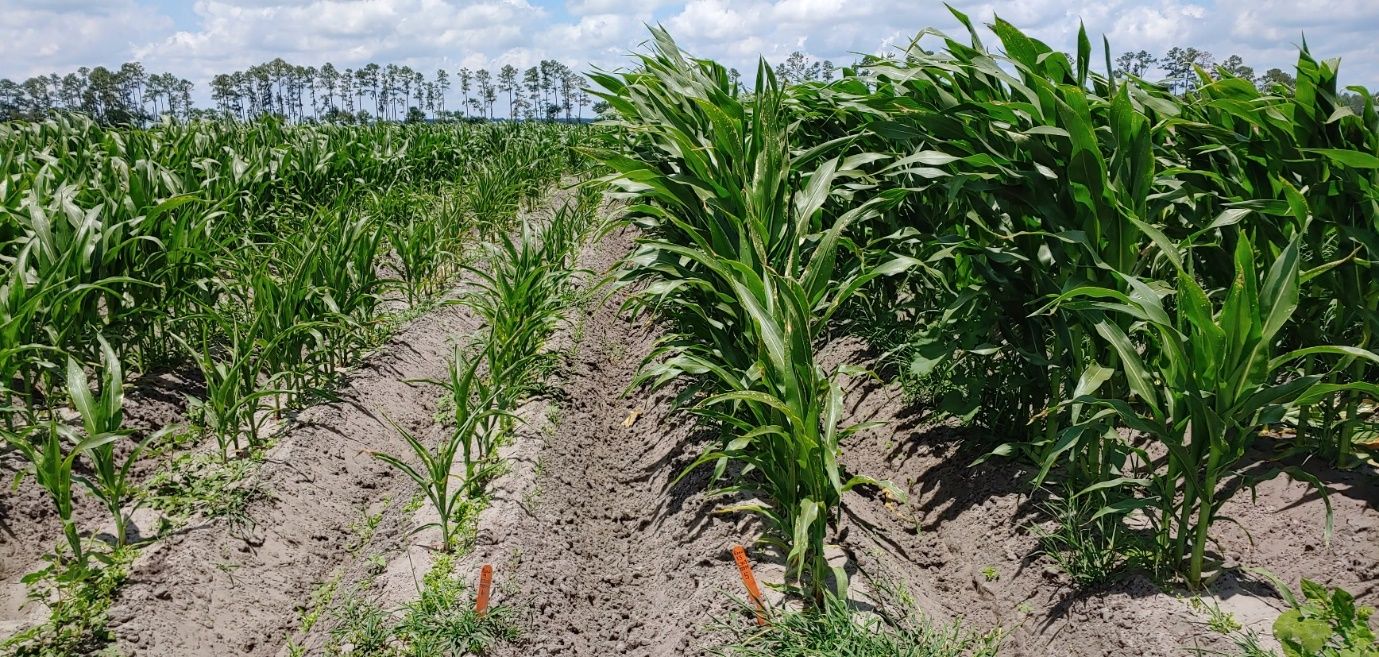
Credit: Zane Grabau, UF/IFAS
Often, the most damaging nematodes in the southeastern United States and the tropics are root-knot nematodes (Meloidogyne spp.). These nematodes are pests of nearly all major crops and are therefore widespread. Damage can be directly observed by examining the roots because root-knot nematodes produce galls or knot-like swellings along the plant roots of most crop species (Figure 4). These galls cannot not be easily removed because they are part of the plant root tissue. In contrast, nodules caused by beneficial nitrogen-fixing bacteria—such as those often found on roots of leguminous crops like peanuts and beans—can be easily removed and have a more uniform shape (Figure 5). Another method to distinguish nematode galls from nodules is to slice them in half and expose them to air, which will cause nitrogen-fixing nodules to appear pink or red if the bacterial colony is active, or green or brown if the colony is inactive.
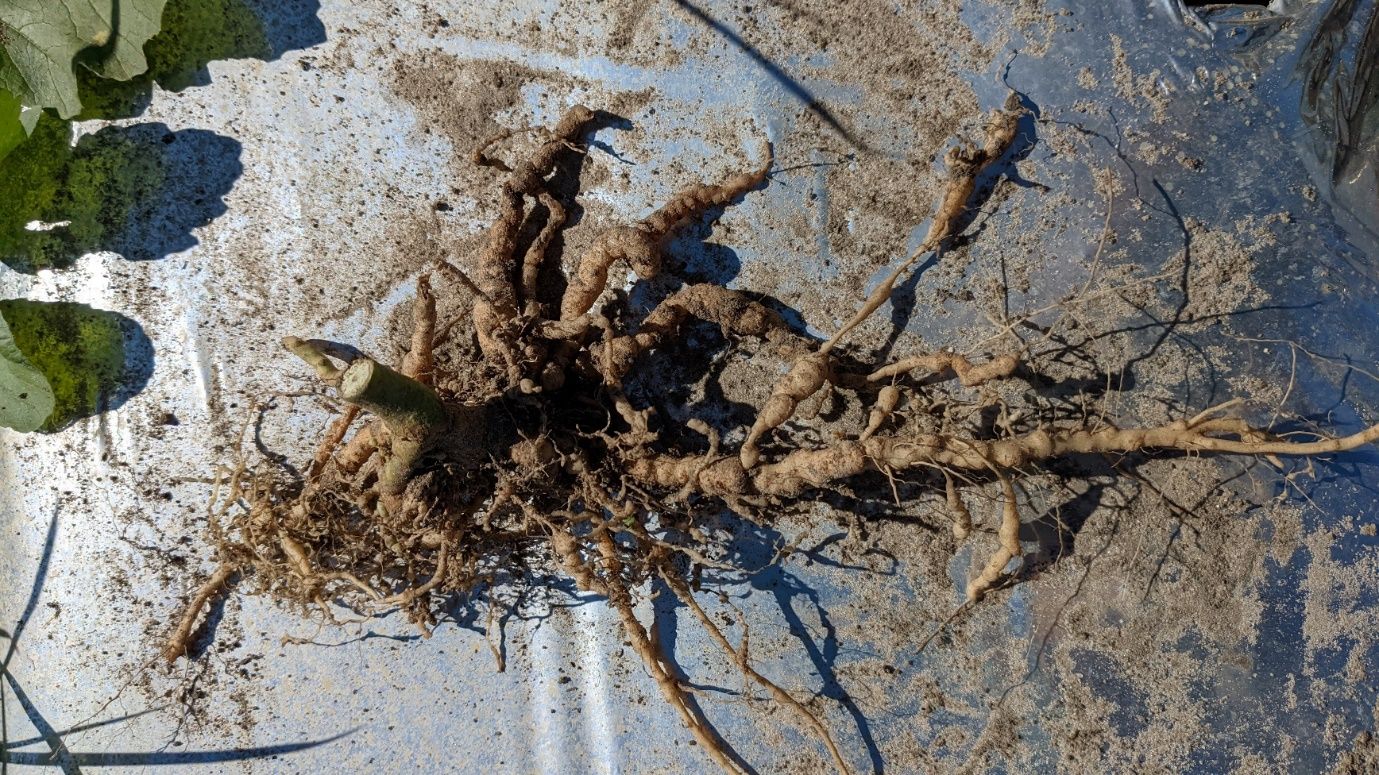
Credit: Zane Grabau, UF/IFAS
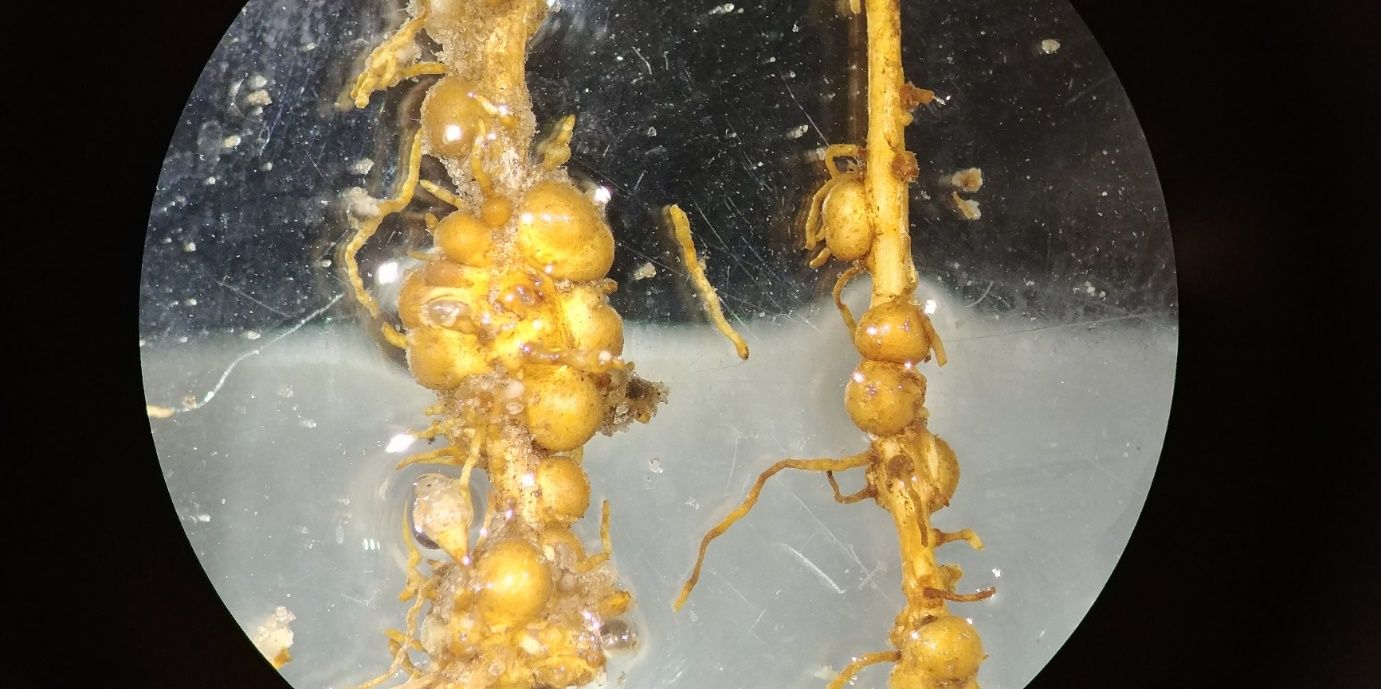
Credit: Zane Grabau, UF/IFAS
Evidence of direct feeding, apparent in the form of galls and other root malformations, leads to the damage symptoms described above. The invasive stage of the root-knot nematode life cycle is the juvenile root-knot nematode, which can freely move through the soil and will enter the root of a suitable host plant. Only the female is capable of establishing a feeding site. The female will become immobile, causing the plant to form giant cells for feeding, which essentially appear as a gall on the root. These characteristic galls reveal that root-knot nematodes are present.
Soil Samples
Plant-parasitic nematodes other than root-knot nematodes do not cause these gall symptoms, and, even when root-knot nematodes are present, galling may not always be distinct. Therefore, soil samples should be taken to determine which nematodes are present in a field. Even when it is clear that root-knot nematodes are to blame for the damage caused, it may be beneficial to find out if other nematodes are present to determine which treatments will work best and should be employed. For further information on how to take soil samples and where to send them, please refer to instructions on the UF/IFAS nematode assay lab website: https://entnemdept.ufl.edu/nematology-assay-lab/ .
Nematode Management
In nematode management, it is important to remember that nematodes can move only very short distances on their own. They spread mainly through unsanitized or poorly sanitized equipment and movement of infected soil and planting material. In order to limit a build-up of nematodes, planting equipment and tools should be properly cleaned. In extreme cases, consider dedicated equipment for each field to avoid spreading infestations. Furthermore only soil and planting material that is known to be free of nematodes should be used because once nematodes are introduced into a field they cannot be eradicated. Using plant material certified by a commodity organization or state agricultural department program, when available, is a reliable way to help ensure plant material is clean. Transplants should only be propagated in nematode-free media (clean or sterilized potting material), and planting material (seeds, cuttings) for establishing transplants should also be nematode-free. After harvest, destroy infected plants to prevent the build-up of nematodes on these crop residues and therefore in the soil. Once they become established in a site, the nematodes will persist there, and management will be required on a regular basis using the strategies described as follows.
Resistant Plants and Rotation Crops
Nematode management is primarily a pre-planting activity. In order to protect the crop, most activities must be started two or three months before the scheduled planting date. Several pre-plant treatments are available for the organic farmer. The choice of a suitable crop cultivar can be a critical decision. Host plant resistance achieved by breeding programs can be a valuable protection against some nematodes.
Two terms that are often used when talking about host plant resistance to nematodes are “tolerance” and “resistance.” Tolerance means that the plant can withstand some damage caused by a particular nematode without experiencing significant yield reduction. In contrast, resistance means that reproduction of a particular nematode is very low or non-existent on the plant. Tolerant or resistant interaction are specific to the crop/cultivar-nematode combination. A crop that is resistant to one nematode may be highly susceptible to a different nematode species. Both resistance and tolerance provide protection for the crop plant, but the next crop following a tolerant plant could be damaged by the nematodes that survived on the tolerant plant. Different plant species or even cultivars of the same plant species can exhibit varying degrees of resistance or tolerance.
Use of resistant cultivars or planting a nematode-resistant cultivar from a crop species that is otherwise susceptible, is one strategy for managing nematodes and minimizing yield loss. Cultivars that are resistant to root-knot nematodes are available for a variety of horticulture and agronomic crops. For information on resistant cultivars available for specific crops, explore UF/IFAS nematode management publications on Ask IFAS.
Crop rotation is another crop selection strategy that can be used to manage nematodes. Crop rotation is the regular alternation of different crops planted in a field. Crop rotation helps with nematode management when a grower’s rotation includes crops that are poor hosts or non-hosts (crops that have high levels of resistance) to the nematodes found in a particular field. These crops may either be cash crops intended for harvest and sales or cover crops that are not typically harvested but that provide benefits to the farming system such as nitrogen enrichment, nematode reduction, and erosion control. In either case, nematode numbers are reduced simply because nematodes are deprived of a suitable host crop. This does not mean that nematode densities are reduced indefinitely, but a successful crop rotation should reduce nematode levels enough so that the following susceptible crop will produce sufficient yields and survive until the end of its regular growing season. In contrast, growing a single crop in monoculture or alternating crops susceptible to the target nematode will increase nematode problems. When multiple nematode species are present in a field, management with rotation becomes more challenging as a crop that is resistant to one nematode species, may increase populations of another. In this case, a combination of management strategies may be needed, and rotation may focus on the most problematic nematode species present.
Summer cover crops that may help manage certain nematodes include cowpea (Figure 6), sorghum, sorghum-sudangrass (Figure 7), marigold, and some tropical legumes such as sunn hemp (Crotalaria juncea, Figure 8) and velvetbean (Mucuna spp.). Winter crops useful for managing certain nematodes include different grasses such as oat and rye or alternative, bahiagrass-based cropping systems (Wright et al. 2018). These cover crops are useful to reduce root-knot nematode population densities. Table 1 lists cover crops, which demonstrated low or intermediate susceptibility to M. arenaria race 1, M. incognita race 1, and M. javanica (McSorley 1999; McSorley and Dickson 1995; McSorley et al. 1994). Even though these cover crops are effective against root-knot nematodes, they might increase other harmful plant-parasitic nematodes that are also present in the soil. For example, densities of the sting nematode (Belonolaimus longicaudatus) and stubby-root nematode (Paratrichodorus minor) increased on ‘SX-17’ sorghum-sudangrass. ‘Mississippi Silver’ cowpea was able to reduce P. minor and M. incognita but increased B. longicaudatus. The most effective cover crop that simultaneously reduced root-knot, stubby-root, and sting nematodes was determined to be velvetbean (McSorley and Dickson 1995). Results vary in different locations as well. In Kenya, a plant related to sunn hemp (C. ochroleuca) that successfully reduced populations of root-knot nematodes appeared to increase the occurrence of lesion nematodes (Pratylenchus zeae), whereas jack bean (Canavalia ensiformis), hyacinth bean (Lablab purpureus), and velvet bean appeared to reduce both species of nematodes (Arim et al. 2006).
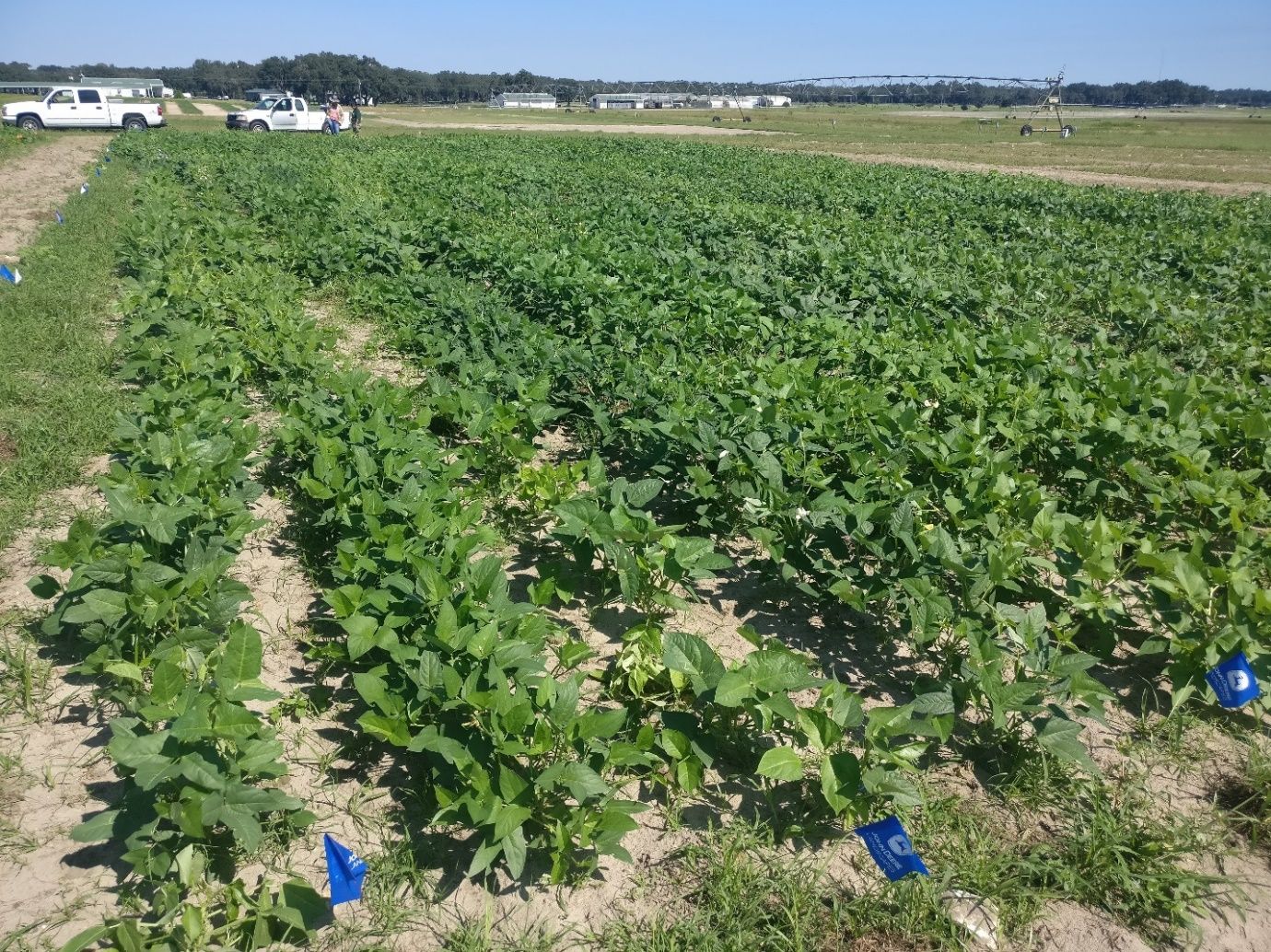
Credit: Esteban Rios, UF/IFAS
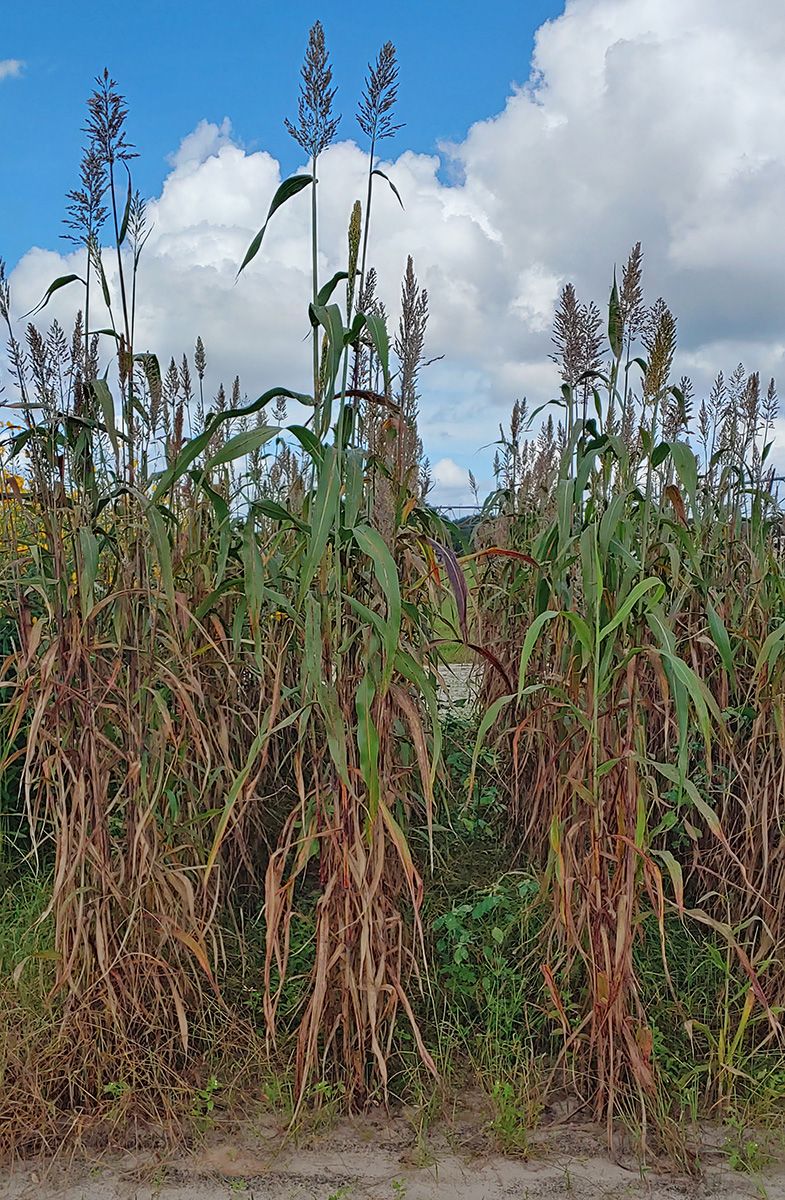
Credit: Zane Grabau, UF/IFAS
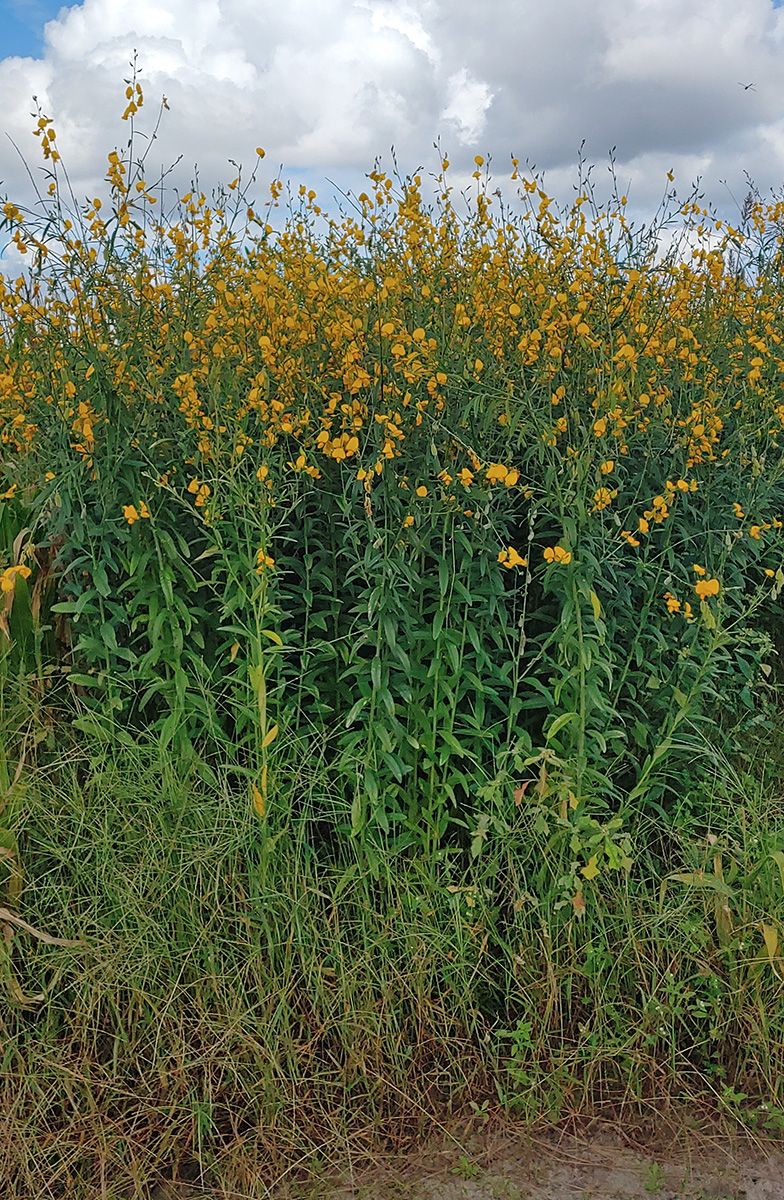
Credit: Zane Grabau UF/IFAS
The response of nematodes is greatly influenced by the cultivar of a crop plant species. Therefore, even if the same plant species is used, results may vary if the cultivar is different. Sorghum is often recommended as a cover crop to decrease population levels of root-knot nematodes and is widely used for this purpose (Dover et al. 2021). However, use of an uncommonly used cultivar (‘Asgrow Chaparral’) actually increased population levels of root-knot nematodes (M. incognita) in a field experiment in north Florida (McSorley and Gallaher 1992). Furthermore, reactions of plants seem also to be site dependent. Cover crops that decrease nematode populations in one field may not be effective in another, depending on local nematode species and their local populations and variants present. Since weeds can harbor nematodes, it is important to have a dense cover crop stand that blocks out weeds. For more comprehensive information on cover crop production benefits, please refer to the publication of the Sustainable Agriculture Research and Education program on cover crops (Magdoff and van Es 2021). For more information on cover crops for managing nematodes, see the following Ask IFAS publications on cover crops and root-knot nematodes (Gill and McSorley 2017), cowpea (Wang et al. 2021), sorghum and its relatives (Dover et al. 2021), and sunn hemp (Wang et al. 2022).
Tillage and Fallowing
Tillage and the practice of fallowing fields may appear as alternatives to cover crops for nematode management. Tillage inverts and mixes soil and exposes deeper soil layers to the sun. This practice can kill nematodes in the upper soil layers by desiccation since nematodes depend on moisture for survival. However, it will not reach nematodes that have retreated into moderate or deeper soil layers. Nematodes can retreat to depths greater than 12 inches (30 cm) in Florida soils and can migrate upward once a susceptible host is planted. Once a field has been fallowed, nematodes will move into deeper soil layers to avoid drying and may enter an inactive stage that enables them to survive periods without food and in addition protects them from desiccation. McSorley and Gallaher (1993) compared the effects of tillage versus crop rotation on nematode densities in tropical corn (Zea mays) cultivar ‘Pioneer X304C’ in North Florida. Tillage did not have significant effects on nematode densities, while a rotation crop of sorghum cultivar ‘DeKalb BR64’ reduced population levels of root-knot nematodes (M. incognita). These results demonstrate that tillage is not a reliable method for nematode management in Florida. Fallowing can deprive nematodes of food if the field is kept 100% weed free and therefore can reduce population levels. But clean fallowing is a poor practice for soil conservation and nutrient runoff. In the absence of plants that keep soil in place, wind will be able to carry some of the topsoil away. Additional soil will be lost with rainwater runoff. Rain will also carry soil nutrients into lakes and rivers, which will degrade water quality and interfere with aquatic ecosystems.
Solarization
A promising technique is the use of heat to decrease not only nematode densities, but also other harmful organisms and weed seeds. This strategy can involve pasteurization, steaming, or solarization of the soil before planting. Of these, solarization (Krueger and McSorley 2018) is probably the most practical (Figure 9). It involves the covering of the soil with clear plastic. Transparent plastic sheets allow short-wave radiation from the sun to penetrate the plastic. Once the light passes through the plastic and is reflected from the soil, the wavelength becomes longer and cannot escape through the plastic. The trapped light facilitates heating of the soil to temperatures detrimental to most living organisms. There are different types of plastic sheets available, mainly differing in their thickness (insulation) and ability to let light through (transparency). Black, opaque, or translucent plastics are not suitable for solarization. Thin, transparent plastic sheets appear to achieve the best results. Katan (1981) recommended thicknesses between 25 to 30 μm. Temperatures can rise up to anywhere between 35°C and 60°C (95°F–140°F) during the summer months (Katan 1981, Stapleton 1991) when air temperatures are close to 32°C (89.6 F) or higher. However, cloud cover and rain limit solar radiation and may diminish the success of solarization (DeVay 1991; Katan 1980). Soil temperatures only rise to detrimental levels in the first 10 to 30 cm of soil (Katan 1987), and even in this range temperatures drop off as depth increases. The plastic has to be sealed to prevent air movement underneath the plastic, which would prevent temperatures from rising sufficiently.
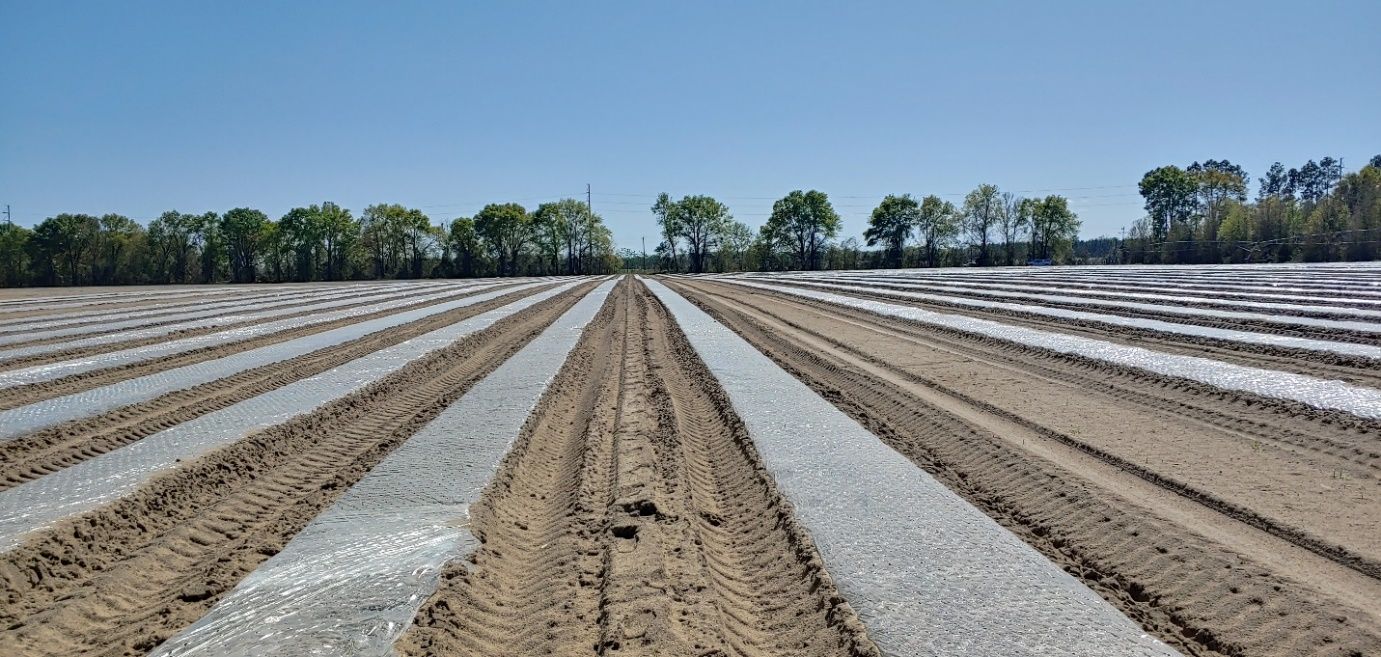
Credit: Zane Grabau, UF/IFAS
The soil should remain covered for a minimum of four weeks, but increasing solarization time improves effectiveness. This helps to heat the soil at a greater depth, which means that more nematodes will be affected by it (McGovern and McSorley 1997). Furthermore, it also ensures that an adequate accumulation of solarized hours is achieved, which is important in regions like Florida where the sky is often overcast during the summer months.
A combination of a suitable cover crop and solarization seems to achieve best results. Wang et al. (2006) showed that following a cowpea cover crop with solarization accomplished better results than solarization alone. In fact, the effectiveness of this combined treatment was comparable to methyl bromide fumigation, which in conventional agriculture was the most effective treatment used against soil-borne diseases and pests. The disadvantage of solarization is its negative impact on beneficial soil organisms, since they will meet the same fate as their harmful counterparts. But recovery is usually attained quickly through rapid recolonization. Furthermore, other beneficials such as Bacillus, Pseudomonas, and Trichoderma are able to survive the high temperatures generated by solarization (Katan 1987). Solarization generates considerable plastic waste, which, when farming with a sustainable approach in mind, is a drawback. However, if they are handled carefully, the plastic sheets may be used multiple times.
Biological Control
Biological control is the management of plant-parasitic nematodes by living organisms such as bacteria, fungi, predatory nematodes, or other invertebrates. Biological control is mainly accomplished by attempting to build up beneficial organisms through the use of various soil amendments as described in the next section. The introduction of beneficial soil organisms to the soil, or inundation, is the second biological control strategy and is increasingly common as more commercial nematicides containing live microbes or derived from microbes are being introduced as described in the section titled “Commercial Nematicidal Organic Products.” Pasteuria spp., which are bacterial parasites of various plant-parasitic nematodes occurring naturally in Florida soils, may be promising biological control agents. In a 7-year experiment with tobacco, Weibelzahl-Fulton et al. (1996) showed that M. incognita and M. javanica were suppressed exclusively by P. penetrans. Tobacco plants treated with P. penetrans had fewer galls, egg masses, and eggs than plants that either did not receive P. penetrans or lacked the biological control agent because the soil in which the plants were grown had been autoclaved. Nematode-trapping fungi are also potential candidates for biological control. Their adhesive knobs, rings, or net structures trap nematodes and kill them. Other types of fungi may parasitize nematode eggs. Similarly, Purpureocillium (formerly Paecilomyces) fungi can also parasitize nematodes (Crow 2013). Certain Bacillus bacterial species have been found to produce compounds that are nematicidal or stimulate plant defense against nematodes (Xiang et al. 2018).
Many of these microbial predators can be found in a healthy soil environment, as can predators such as mites and predatory nematodes. A wide range of nematode natural enemies occur in Florida soils (McSorley et al. 2006). Most predators are generalists, meaning they feed on a variety of prey species during their lifetime. For biological control purposes, generalist predators are a disadvantage compared with specialists, because the generalists will not only feed on the key pest, but on other suitable organisms as well, including beneficials. Furthermore, if there is not enough food available, generalist predators will disperse, possibly causing the pest to rebound. On the other hand, generalist predators can keep many different kinds of pests at low population densities. Currently, no predatory nematodes or mites are commercially available for augmentive releases for nematode control in crop production systems.
Organic Amendments in Relation to Biological Control
Biological control is difficult in soil because it is a complex environment. Many of the possible organisms that could provide biological control lack specificity and therefore will not focus on a particular organism and may even interfere with beneficials. Therefore, biological control of nematodes is achieved mainly by conservation of existing biological control; meaning that the soil environment is modified to aid the survival and reproduction of nematode natural enemies that are already present. Primarily this is accomplished through the addition of organic amendments (Figure 10). Organic amendments can improve the soil environment to aid biological control, benefit general plant health by helping with water retention and providing additional nutrients, and affect nematodes directly and negatively through detrimental decomposition products. The impact of organic amendments on nematodes is often inconsistent and unpredictable. In most cases when organic amendments are applied, they are helpful mainly as a plant nutrient source and do not directly aid in nematode management. However, even if nematodes are unaffected by the added amendments, plant health may improve due to other favorable properties of amendments. McSorley and Gallaher (1995) observed that amendments consisting of yard waste compost (four- to six-month-old wood fragments, leaves, grass clippings) used as mulch or incorporated into the soil improved yield of squash and okra even though plants experienced heavy galling. Although nematode densities in composted treatments were similar to those in non-amended soil, plants benefited greatly from the improved retention of soil moisture. Kimpinski et al. (2003) showed similar results. Amendments (compost and manure) increased yield in barley and potatoes in Canada, but this was not necessarily due to reduced nematode numbers.
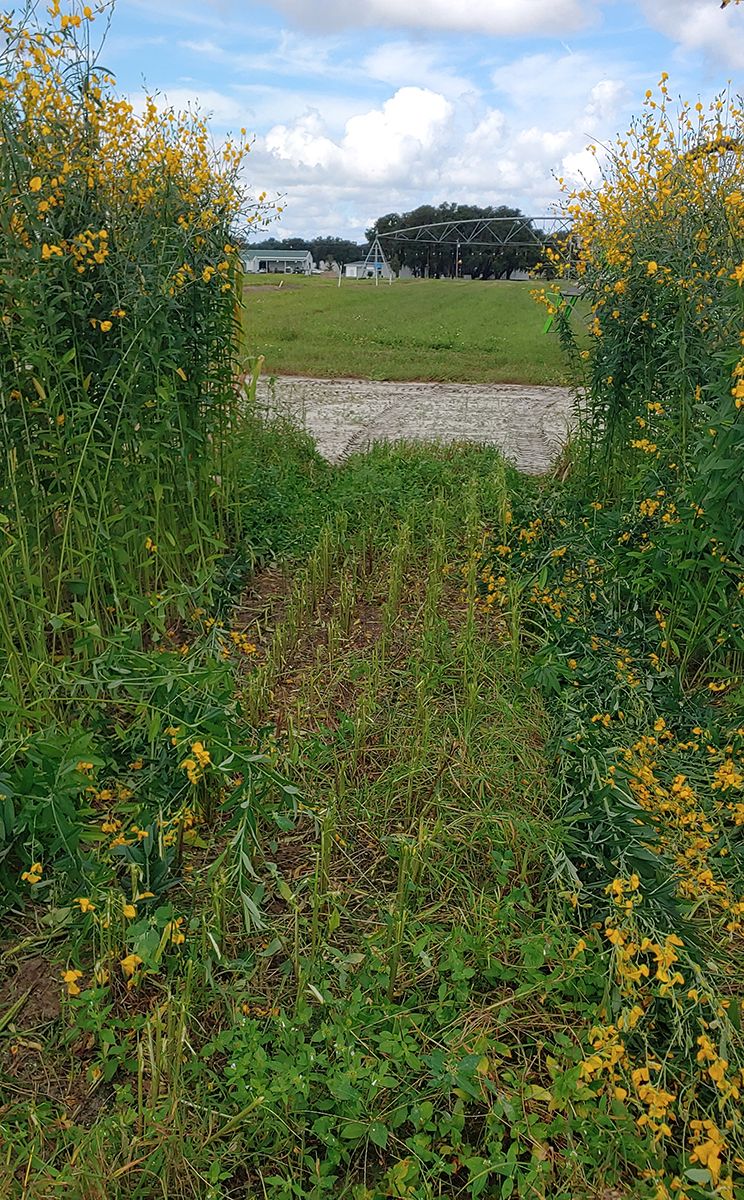
Credit: Zane Grabau UF/IFAS
The addition of organic amendments may stimulate the entire soil food web, including beneficial biological control organisms. When soil is amended with nutrient-rich organic matter, microorganisms may immobilize the released nutrients (Swift et al. 1979). Free-living nematodes that feed on these bacteria and fungi may play an important role in the release of nutrients tied up in such a way (Ingham et al. 1985). Soil-inhabiting microorganisms and nematodes therefore may act together to influence the rate of decomposition and release of nitrogen from crop residues, including nitrogen-rich leguminous crops like sunn hemp. Wang et al. (2004) investigated effects of sunn hemp decomposition on the soil nematode community. Litterbags that allowed nematodes to pass in and out were filled with sunn hemp hay and buried in soil. Periodic analysis of the bags showed that sunn hemp decomposition was fairly rapid, and most was completed within two weeks. Population densities of nematodes feeding on bacteria increased greatly during decomposition, and this was followed by an increase in omnivorous nematodes that fed on them. However, plant-parasitic nematodes were neither affected by the sunn hemp decomposition nor by the omnivorous nematodes. In fact, plant-parasitic nematode numbers increased over time with increasing crop biomass, which may indicate that sunn hemp residue does not have a direct detrimental effect on plant-parasitic nematodes. In another recent study, Chellemi (2006) used urban plant debris (green waste from public landfill deposited by homeowners and landscape companies) to amend pepper fields in southeast Florida. As a result, the combined density of plant-parasitic nematodes was reduced, and other disease-causing plant pathogens (Pythium spp. and Phytophthora spp.) were reduced as well. These examples illustrate the potential use of organic amendments for direct or indirect management of nematodes, but they also indicate that outcomes can be complex and unpredictable.
Commercial Nematicidal Organic Products
As described above, some bacteria and fungi have been identified as biological control agents against plant-parasitic nematodes. Increasingly, these biological control agents are being developed into commercial nematicides certified for use in organic production. Some of these nematicides may be useful for helping manage plant-parasitic nematodes as part of an integrated pest management strategy including the other strategies described in this publication. These products can be grouped into two categories (1) formulations of live microorganisms, and (2) formulations of dead or inactivated microorganisms. The live organism products are intended to work by inundation, introducing the live organism into the soil allowing the microorganisms to parasitize plant-parasitic nematodes. Often these products must be handled carefully to ensure the microorganisms remain alive. For instance, they may be refrigerated until the product is applied. Purpureocillium lilacinus formulations (commercially available from a company called MeloCon LC) are an example of a commercial product containing live organisms that has shown some efficacy in managing plant-parasitic nematodes (Crow 2013). For a full list of organic nematicides labelled for Florida horticulture crops, see the Ask IFAS publication https://edis.ifas.ufl.edu/publication/NG033 (Watson and Desager 2019). Not all labelled products may be effective. Consult UF/IFAS efficacy data in UF/IFAS crop-specific nematode management guides or by contacting the corresponding author of this article.
Organic nematicides with formulations of dead microorganisms typically contain compounds produced by the microorganisms that are toxic to nematodes. These products are similar to many chemical nematicides in that they generally require the toxic compounds to come in contact with the nematodes and do not require the microorganism to be alive. Formulations of heat-killed Burkholderia bacteria (Majestene) are one example of this type of product that has shown some efficacy in UF/IFAS trials (Grabau and Noling 2021). Similarly, there are plant-derived commercial products available, such as essential oils of plants in the Brassicaceae family. They are also intended to work primarily by contact toxicity. While some plants are known to contain products toxic to nematodes, such as Brassicas (Dutta et al. 2019), not all formulations derived from these plants have been tested, so confirming product efficacy using UF/IFAS resources described in the previous paragraph is important.
Management of Infected Plants
Once plants are infected with nematodes, there is little that can be done to remove or reduce nematodes. Therefore, prevention and sanitation are critical to controlling nematodes. The improvement of plant health is an important cultural technique to lessen detrimental effects on plants caused by plant-parasitic nematodes. Proper irrigation, fertilization, and organic amendments used as surface mulches or incorporated into the soil are important. Furthermore, removal of weed hosts and old crop plants immediately after harvest can reduce nematode densities for the future. Nematodes are a long-term pest, which cannot be eradicated once they become established in a site. They can only be kept at low levels with carefully selected management tactics that are often specific to the managed site.
A variety of additional methods may have some effect on nematodes. These include methods such as use of chitin, sesame residues, flooding, or microwave energy. Some of these (flooding and microwaving, for instance) may be restricted to specialized situations. Performance of these methods may be inconsistent or ineffective in some situations. Amendments such as chitin or sesame residue may provide nutrients that are beneficial to overall plant health regardless of any effects on nematodes. In these cases, only small test areas should be subjected to new materials to determine their effectiveness under local conditions. Many of the methods described above such as host plant resistance and rotation are more dependable under a variety of conditions.
References
Arim, O. J., J. W. Waceke, S. W. Waudo, and J. W. Kimenju. 2006. “Effects of Canavalia ensiformis and Mucuna pruriens Intercrops on Pratylenchus zeae Damage and Yield of Maize in Subsistence Agriculture.” Plant and Soil 284:243–251. https://doi.org/10.1007/s11104-006-0053-9
Magdoff, F., and H. van Es. 2021. Cover Crops, chap. 10 In: “Building Soils for Better Crops: Ecological Management for Healthy Soils,” Handbook Series Book 10, Sustainable Agriculture Research and Education, University of Maryland Printing Services, College Park, MD, 4th Edition.
Chellemi, D. O. 2006. “Effect of Urban Plant Debris and Soil Management Practices on Plant Parasitic Nematodes, Phytophthora Blight and Pythium Root Rot of Bell Pepper.” Crop Protection 25:1109–1116. https://doi.org/10.1016/j.cropro.2006.02.012
Crow, W. T. 2013. “Effects of Commercial Formulation of Paecilomyces lilacinus Strain 251 on Overseeded Bermudagrass Infested with Belonolaimus longicaudatus.” Journal of Nematology 45:223–227.
DeVay, J. E. 1991. “Historical Review and Principles of Soil Solarization,” chap. 2 In Soil Solarization, edited by J. E. DeVay, J. J. Stapleton, and C. L. Elmore. FAO Plant Production Paper 109, FAO, Rome.
Dover, K., K. –H. Wang, Z. J. Grabau, and R. McSorley. 2021. “Nematode Management Using Sorghum and Its Relatives.” ENY-716/IN531. EDIS 2021 (6). https://doi.org/10.32473/edis-IN531-2021
Dutta, T. K., M. R. Khan, and V. Phani. 2019. “Plant-Parasitic Nematode Management via Biofumigation Using Brassica and Non-Brassica Plants: Current Status and Future Prospects.” Current Plant Biology 17:17–32 https://doi.org/10.1016/j.cpb.2019.02.001
Gallaher, R. N., and R. McSorley. 1993. “Population Densities of Meloidogyne incognita and Other Nematodes Following Seven Cultivars of Cowpea.” Nematropica 23:21–26.
Gill, H. K., and R. McSorley. 2017. “Cover Crops for Managing Root-Knot Nematodes.” ENY-063/IN892. EDIS 2017. https://edis.ifas.ufl.edu/publication/IN892
Grabau, Z. J., and J. W. Noling. 2021. Nematode management in cole crops. ENY-024/NG024. EDIS 2021 (1):13. https://doi.org/10.32473/edis-ng024-2020
Ingham, R. E., J. A. Trofymow, E. R. Ingham, and D. C. Coleman. 1985. “Interactions of Bacteria, Fungi, and Their Nematode Grazers: Effects on Nutrient Cycling and Plant Growth.” Ecological. Monographs 55: 119–140. https://doi.org/10.2307/1942528
Katan, J. 1980. “Solar Pasteurization of Soils for Disease Control: Status and Prospects.” Plant Disease 64:45–54. https://doi.org/10.1094/PD-64-450
Katan, J. 1981. “Solar Heating (Solarization) of Soil for Control of Soil Borne Pests.” Annual Review of Phytopathology 19:211–236. https://doi.org/10.1146/annurev.py.19.090181.001235
Katan, J. 1987. “Soil Solarization,” in Innovative Approaches to Plant Disease Management, edited by I. Chet. John Wiley & Sons, New York, p. 77.
Kimpinski, J., C. E. Gallant, R. Henry, J. A. Macleod, J. B. Sanderson, and A. V. Sturz. 2003. “Effect of Compost and Manure Soil Amendments on Nematodes and on Yields of Potato and Barley: A 7-Year Study.” Journal of Nematology 35:289–293.
Krueger, R., and R. McSorley. 2018. “Solarization for Pest Management in Florida.” ENY-902/IN824. EDIS 2018. https://edis.ifas.ufl.edu/publication/in824
McGovern, R. J. and R. McSorley. 1997. “Physical Methods of Soil Sterilization for Disease Management Including Soil Solarization.” Ch. 12 in Environmentally Safe Approaches to Crop Disease Control, edited by N. A. Rechcigl and J. E. Rechcigl. CRC Lewis Publishers, Boca Raton, FL.
McSorley, R. 1999. “Host Suitability of Potential Cover Crops for Root-Knot Nematodes.” Journal of Nematology 31:619–623.
McSorley, R., and R. N. Gallaher. 1992. “Comparison of Nematode Population Densities on Six Summer Crops at Seven Sites in North Florida.” Journal of Nematology 24:699–706.
McSorley, R., and R. N. Gallaher. 1993. “Effect of Crop Rotation and Tillage on Nematode Densities in Tropical Corn.” Journal of Nematology 25:814–819.
McSorley, R., and D. W. Dickson, and J. A. de Brito. 1994. “Host Status of Selected Tropical Rotation Crops to Four Populations of Root-Knot Nematodes.” Nematropica 24:45–53.
McSorley, R., and D. W. Dickson. 1995. “Effect of Tropical Rotation Crops on Meloidogyne incognita and Other Plant-Parasitic Nematodes.” Journal of Nematology 27:535–544.
McSorley, R., and R. N. Gallaher. 1995. “Cultural Practices Improve Crop Tolerance to Nematodes.” Nematropica 25:53–60.
McSorley, R., K. H. Wang, N. Kokalis-Burelle, and G. Church. 2006. “Effects of Soil Type and Steam on Nematode Biological Control Potential of the Rhizosphere Community.” Nematropica 36:197–214.
Stapleton, J. J. 1991. “Thermal Inactivation of Crop Pests and Pathogens and Other Soil Changes Caused by Solarization.” Ch. 3 in Soil Solarization, edited by J. E. DeVay, J. J. Stapleton, and C. L. Elmore. FAO Plant Production Paper 109, FAO, Rome.
Swift, M. J., O. W. Heal, and J. M. Anderson. 1979. “Decomposition in Terrestrial Ecosystems,” in Studies in Ecology, vol. 5. University of California Press, Berkley, CA, 372 pp.
Wang, K. -H., R. McSorley, A. J. Marshall, and R. N. Gallaher. 2004. “Nematode Community Changes Associated with Decomposition of Crotalaria juncea Amendment in Litterbags.” Applied Soil Ecology 27:31–45. https://doi.org/10.1016/j.apsoil.2004.03.006
Wang, K. -H., R. McSorley, and N. Kokalis-Burelle. 2006. “Effects of Cover Cropping, Solarization, and Soil Fumigation on Nematode Communities.” Plant and Soil 286:229–243. https://doi.org/10.1007/s11104-006-9040-4
Wang, K. –H., R. McSorley, Z. J. Grabau, and E. Rios. 2021. “Management of Nematodes with Cowpea Cover Crops.” ENY-712/IN516. EDIS 2004 (13). https://doi.org/10.32473/edis-in516-2004
Wang, K. –H., R. McSorley, and Z. J. Grabau. 2022. Management of Nematodes and Soil Fertility with Sunn Hemp Cover Crop.” ENY-717/NG043. EDIS 2022. https://edis.ifas.ufl.edu/publication/NG043
Watson, T. T., and J. A. Deseager. 2019. “Non-Fumigant Nematicides Registered for Vegetable Crop Use.” ENY-033/NG033. EDIS 2020 (1):4. https://doi.org/10.32473/edis-ng033-2019
Weibelzahl-Fulton, E., D. W. Dickson, and E. B. Whitty. 1996. “Suppression of Meloidogyne incognita and M. javanica by Pasteuria penetrans in Field Soil.” Journal of Nematology 28:43–49. 1996.
Wright, D. L., J. J. Marois, S. George, and I. Small. 2018. “Sod/Livestock-Based Peanut/Cotton Production System: The Next Generation Conservation Cropping System.” SS-AGR-126/AG258. EDIS 2018. https://edis.ifas.ufl.edu/publication/AG258
Xiang, N., K. S. Lawrence, and P. A. Donald. 2018. “Biological Control Potential of Plant Growth-Promoting Rhizobacteria Suppression of Meloidogyne incognita on Cotton and Heterodera glycines on Soybean: A Review.” Journal of Phytopathology 166:449–458. https://doi.org/10.1111/jph.12712
Table 1. Cover crops, which demonstrated low or intermediate susceptibility to M. arenaria race 1, M. incognita race 1, and M. javanica (McSorley 1999; McSorley and Dickson 1995; McSorley et al. 1994; Krueger et al. 2007).