Introduction
A consistent soil testing program is a valuable best management practice (BMP) that allows sugarcane growers to make sound economic fertilization decisions. However, soil testing in Florida has two limitations. First, soil tests are either not available or are not calibrated for nitrogen and micronutrients. Second, soil samples are routinely taken only before sugarcane is planted and rarely are soil samples collected for ratoon crops. Generally, soil samples are not routinely taken from fields with actively growing sugarcane plants since the practice of banding fertilizers in the furrow at planting, along with subsequent sidedress applications of fertilizer sources during the growing season, makes it very difficult to obtain a representative soil sample.
Use of leaf nutrient analysis in combination with visual evaluation of malnutrition symptoms can complement a grower's soil testing program and add additional information that will improve nutrient management decisions. Leaf analysis provides a picture of crop nutritional status at the time of sampling, while soil testing provides information about the continued supply of nutrients from the soil. Leaf analysis allows for early detection of nutritional problems and enables the grower to add supplemental fertilizer to the current year's crop or to adjust next year's fertilizer application. It is also used to help diagnose a nutritional problem in a particular field or localized area of a field where poor growth or other symptoms have been observed. Although specific fertilizer recommendations are not provided for a given leaf nutrient analysis, deficiencies or imbalances indicate where additions or changes in the fertility program are needed. Leaf analysis and knowledge of visual symptoms can be used along with soil-test values and fertilizer and crop records to make improved decisions regarding fertilization. Nutrient management for sugarcane using leaf analysis is discussed in a companion publication by McCray and Mylavarapu (2020) (https://edis.ifas.ufl.edu/ag345).
Leaf Analysis Evaluation Methods
There are two methods for evaluating the nutrient status of sugarcane, the Critical Nutrient Level (CNL) approach and the Diagnosis and Recommendation Integrated System (DRIS). Leaf sampling and preparation procedures are discussed in a companion EDIS publication by McCray et al. (2021) (https://edis.ifas.ufl.edu/sc076).
The CNL approach defines a nutrient concentration below which the nutrient is considered to limit production. It refers specifically to the concentration of a particular nutrient in a particular plant part at a specific stage of growth at which production losses reach 5%–10%. For Florida sugarcane, the top visible dewlap (TVD) leaf blade is sampled during the grand growth period of June to August. When using this approach, it is particularly important to collect leaf samples at the specified growth stage used for reference standards because nutrient contents change during the crop growth cycle. The CNL approach may also include using a nutrient's optimum range, defined as the range of concentration of a nutrient considered optimum for production. Within this range, there should be no deficiency or excess of a given nutrient. Sugarcane leaf nutrient critical values and optimum ranges are given in Table 1.
DRIS calculates indices relative to zero by comparing leaf nutrient ratios with those found in a high-yielding population. In the mid-1980s a DRIS application for Florida sugarcane was developed (Elwali and Gascho, 1983; 1984). DRIS requires a large number of observations of plant tissue nutrient concentrations and associated crop yields, which are used to define separate low-yielding and high-yielding populations and are also used to determine nutrient ratio means for the high-yielding population. A calibration formula uses the means and standard deviations of the nutrient ratios to calculate relative indices for individual nutrients that can range from negative to positive. When a relative index for a specific nutrient is equal to zero, then the associated nutrient ratios are similar to those of the high-yielding test population. The more negative an index for a given nutrient, the more likely the nutrient is present at insufficient levels relative to other nutrients. A positive index indicates the nutrient is present in excess relative to other nutrients. The Nutrient Balance Index (NBI) can be calculated by adding the absolute value of all individual indices together. As the NBI increases, the more out of balance a leaf analysis is considered to be. DRIS incorporates a measure of the balance between nutrients and can indicate problems that are not as obvious with the CNL approach. It also has the advantage of not being as sensitive to the stage of growth as the CNL approach, which allows a wider time frame in which to collect samples. It is important to note that the use of one approach does not exclude the use of the other. DRIS is simply another valuable tool that can be used to examine nutrient balance, and offers additional interpretations beyond the evaluation of leaf nutrient concentrations alone.
Because of the large number of calculations required to determine DRIS indices, a computer program is required. An Excel spreadsheet programmed for sugarcane DRIS calculations is available at the University of Florida/IFAS Everglades Research and Education Center (EREC) website (http://erec.ifas.ufl.edu/). At the UF/IFAS EREC website homepage, the Sugarcane DRIS Calculator is listed under the heading "Soil Testing Laboratory." Click on the DRIS Calculator and you will have the option of opening or saving the Excel spreadsheet programmed for the calculations. The nutrient concentrations required for the calculations are nitrogen, phosphorus, potassium, calcium, magnesium, iron, manganese, zinc, and copper. Questions about the DRIS spreadsheet can be directed to Mabry McCray (jmmccray@ufl.edu).
A cooperative research effort is being made between UF/IFAS scientists and Florida sugarcane growers to use leaf nutritional analysis to improve growers' fertility programs. Trials in grower fields indicated that there was not a consistent yield response to a mid-season summer fertilizer supplement based on spring leaf analysis (McCray et al. 2010). A more cost-effective use of leaf analysis appears to be with the adjustment of the next amendment or fertilizer application, generally for next year's crop or at the next sugarcane planting, rather than adding an additional fertilizer supplement to the current crop. As improvements are made in our ability to use sugarcane leaf nutritional data, updates will be made available in EDIS.
Field Identification of Nutritional Problems
Visual symptoms of nutrient deficiencies and toxicities can often be the first sign that a particular field or location within a field has a nutritional problem. Recognizing these visual symptoms is an important step when designing corrective action. Further evaluations can be pursued with detailed leaf and soil sampling. The pictures of visual symptoms included in this document can also be found in the publication "Sugarcane Nutrition," by D. L. Anderson and J. E. Bowen (1990). These photographs are from various researchers from sugarcane growing areas around the world. The elements included are arranged alphabetically.
Aluminum (Al)
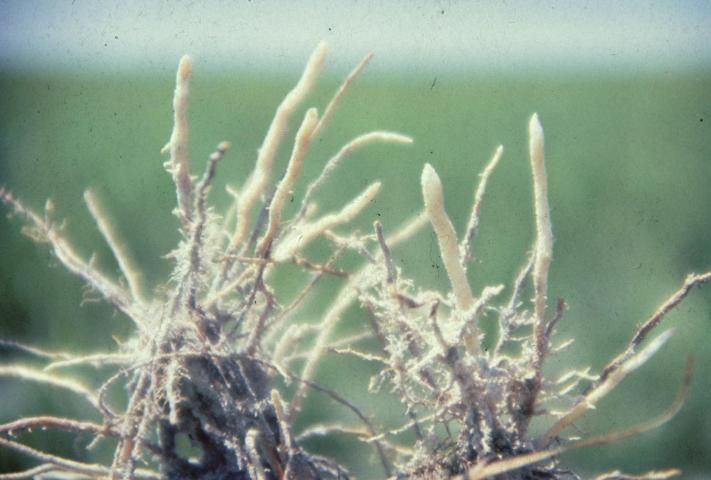
Credit: D. L. Anderson
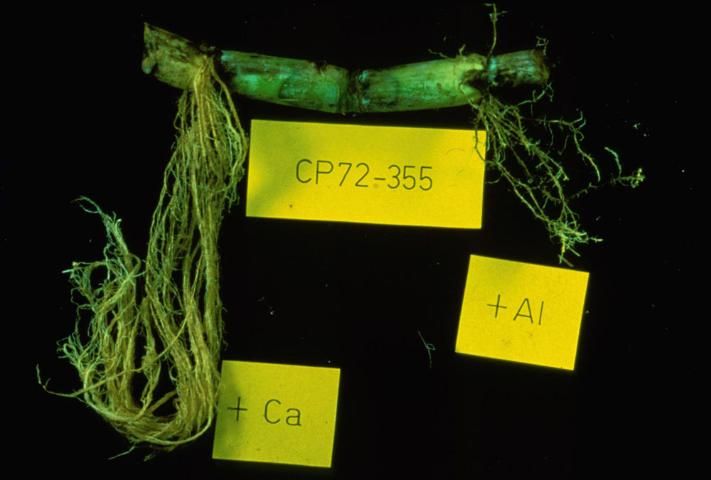
Credit: D. L. Anderson
Boron (B)
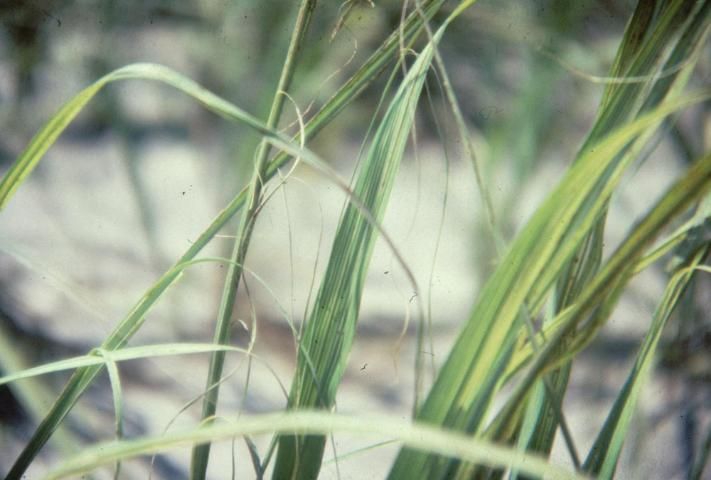
Credit: D. L. Anderson
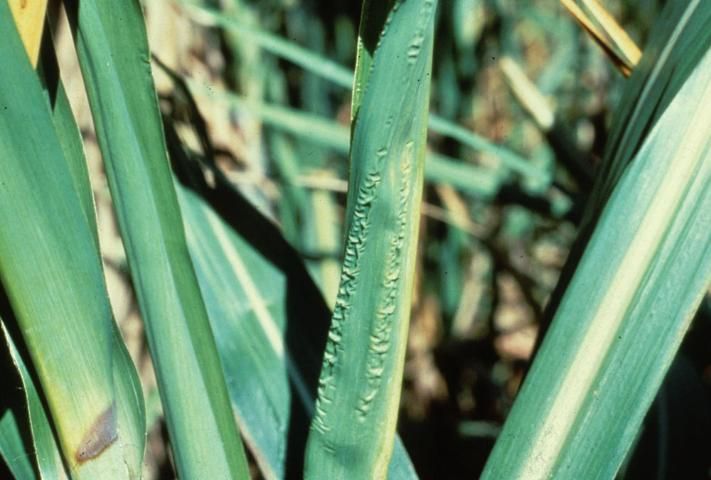
Credit: J. Orlando Filho
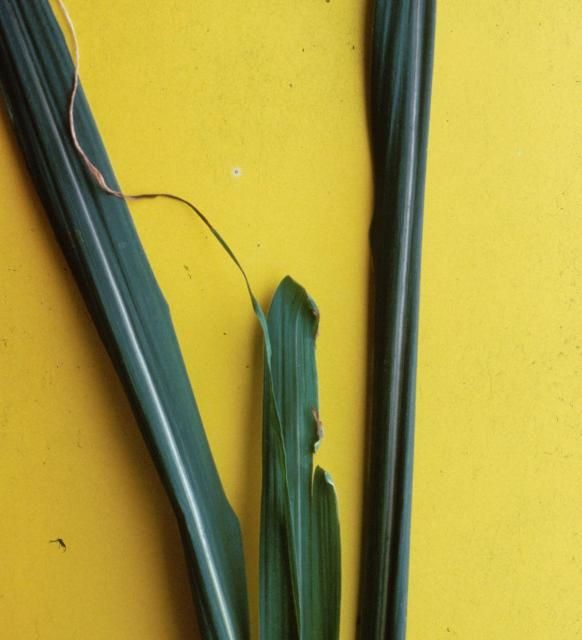
Credit: J. E. Bowen
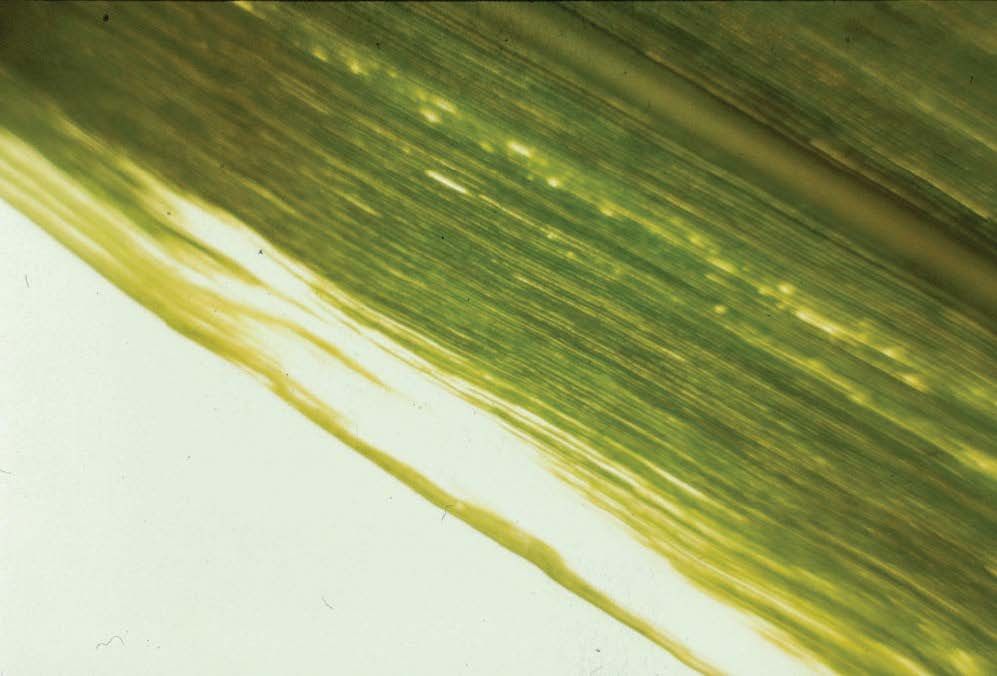
Credit: J. E. Bowen
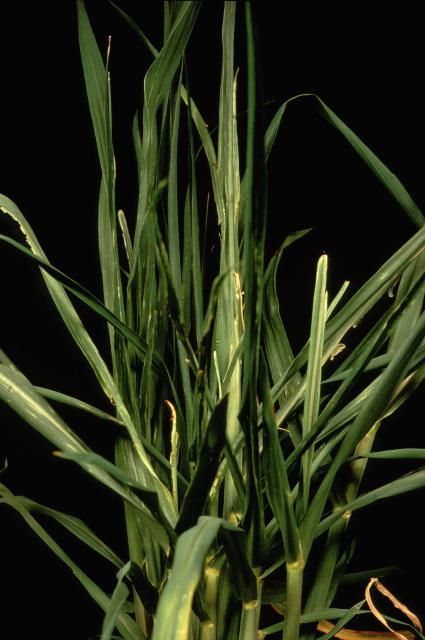
Credit: G. J. Gascho
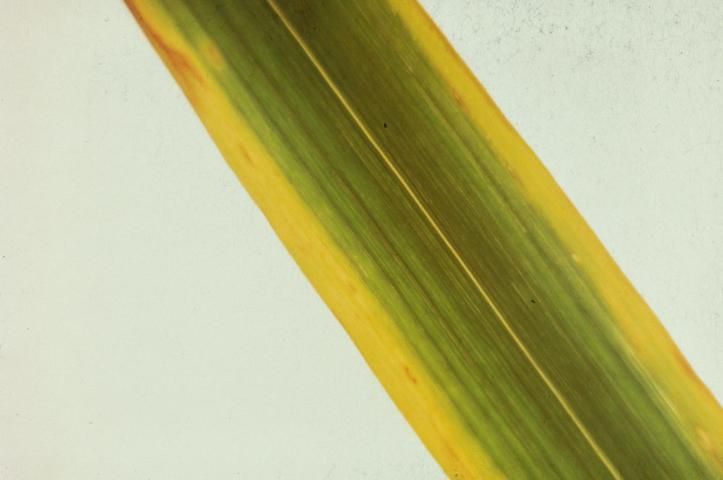
Credit: J. E. Bowen
Calcium (Ca)
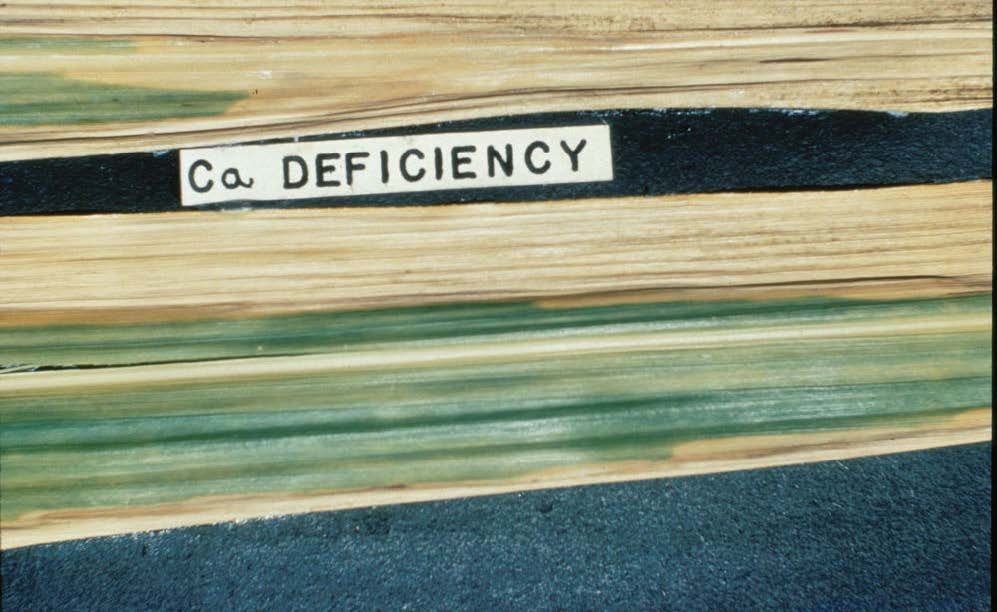
Credit: G. Samuels
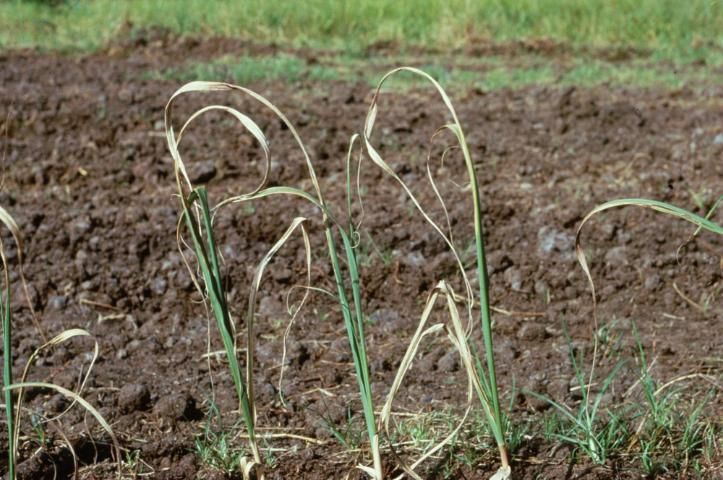
Credit: G. Samuels
Chlorine (Cl)
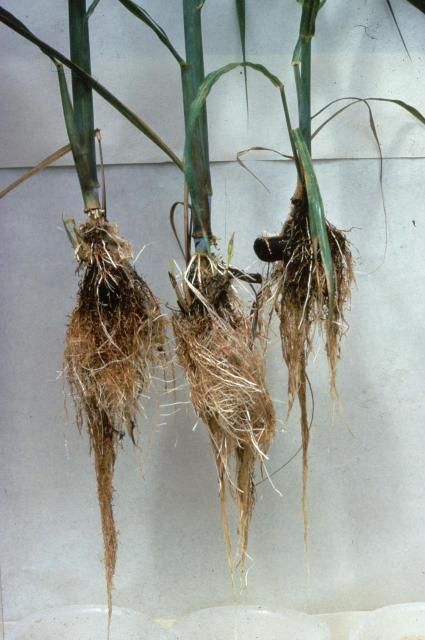
Credit: J. E. Bowen
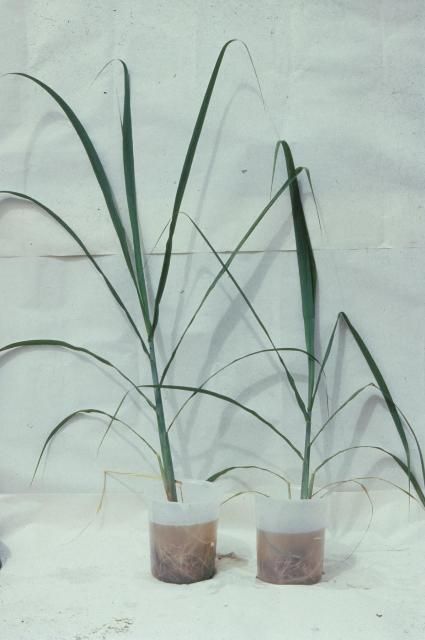
Credit: J. E. Bowen
Copper (Cu)
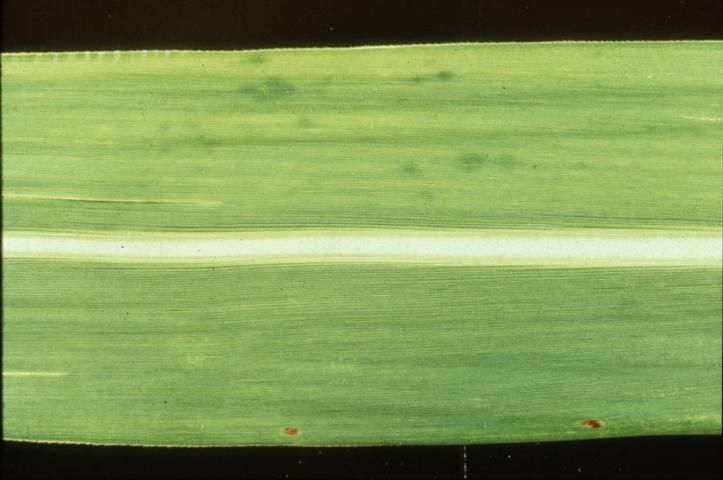
Credit: G. J. Gascho
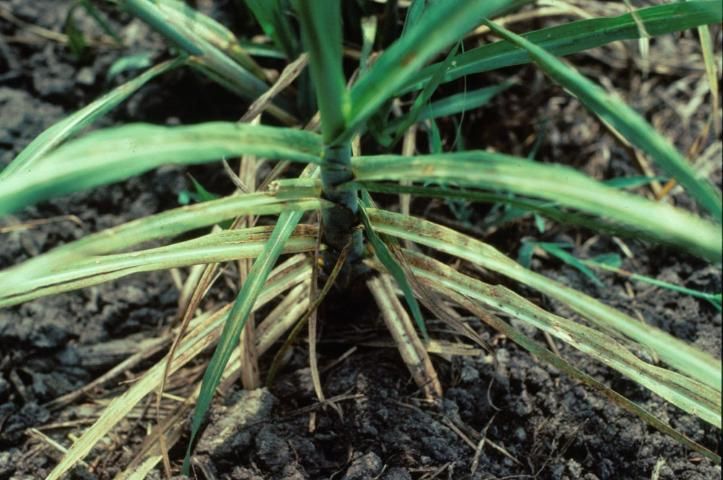
Credit: D. L. Anderson
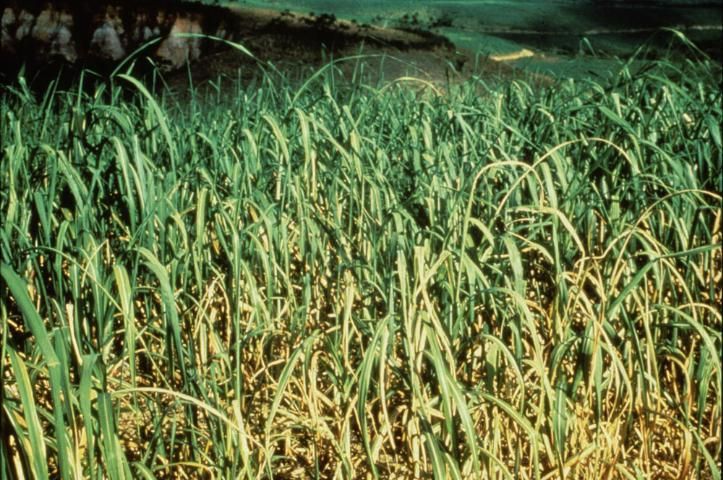
Credit: J. Orlando Filho
Iron (Fe)
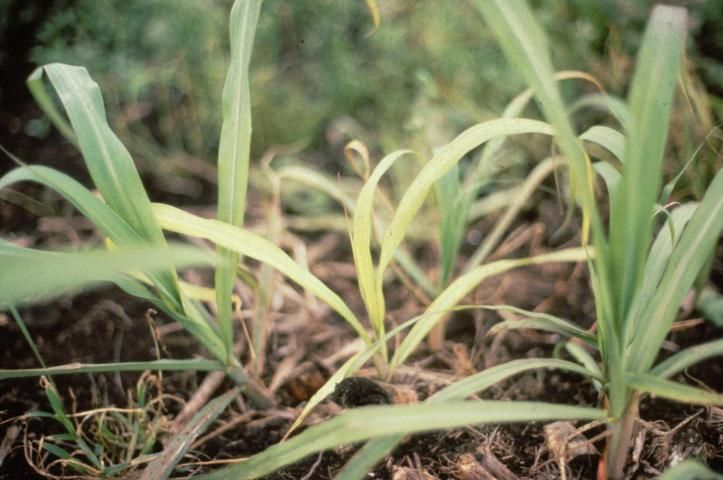
Credit: D. L. Anderson
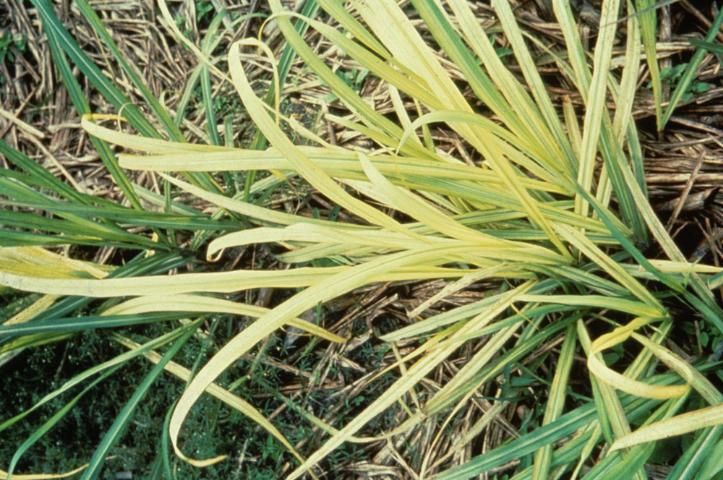
Credit: J. Orlando Filho
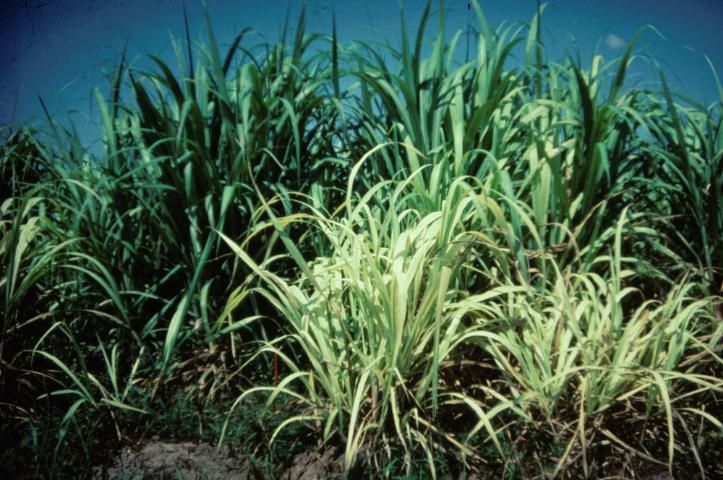
Credit: D. L. Anderson
Magnesium (Mg)
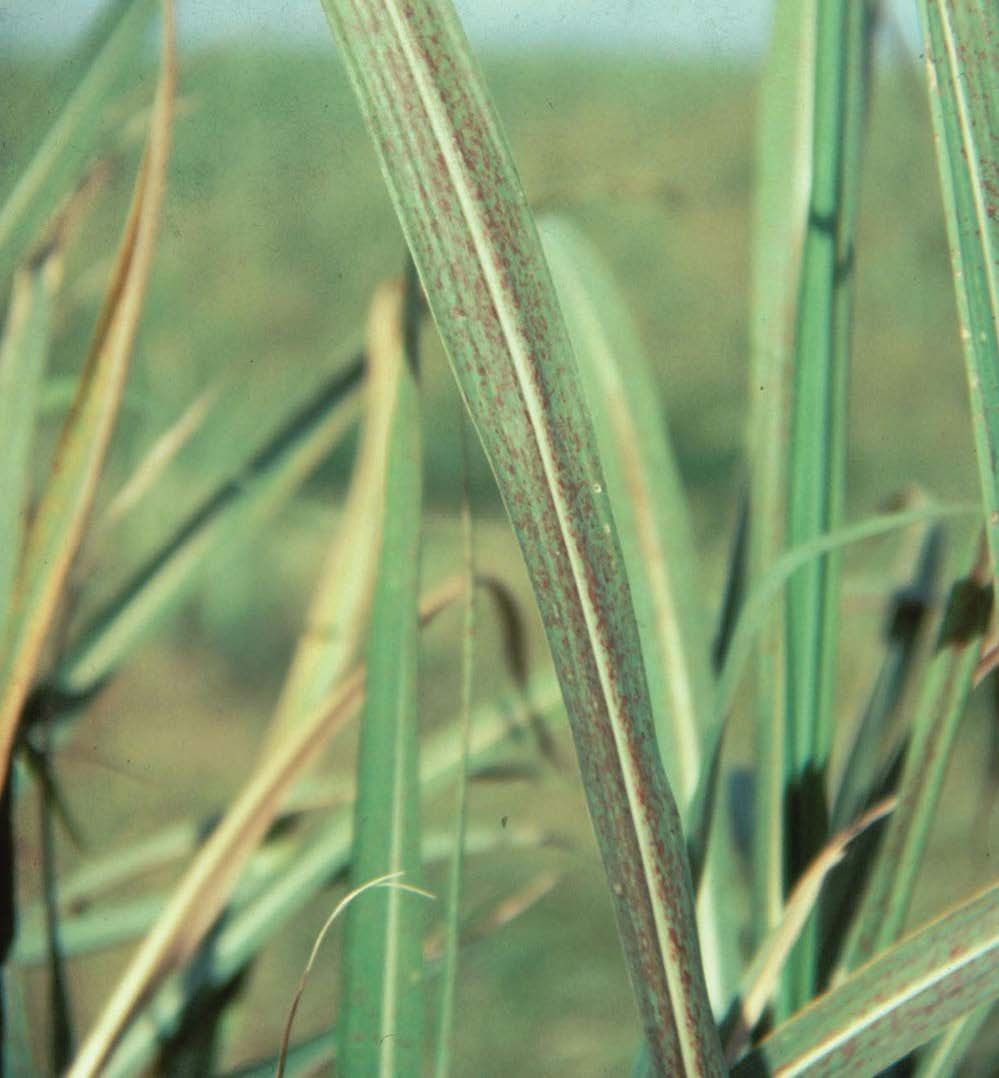
Credit: D. L. Anderson
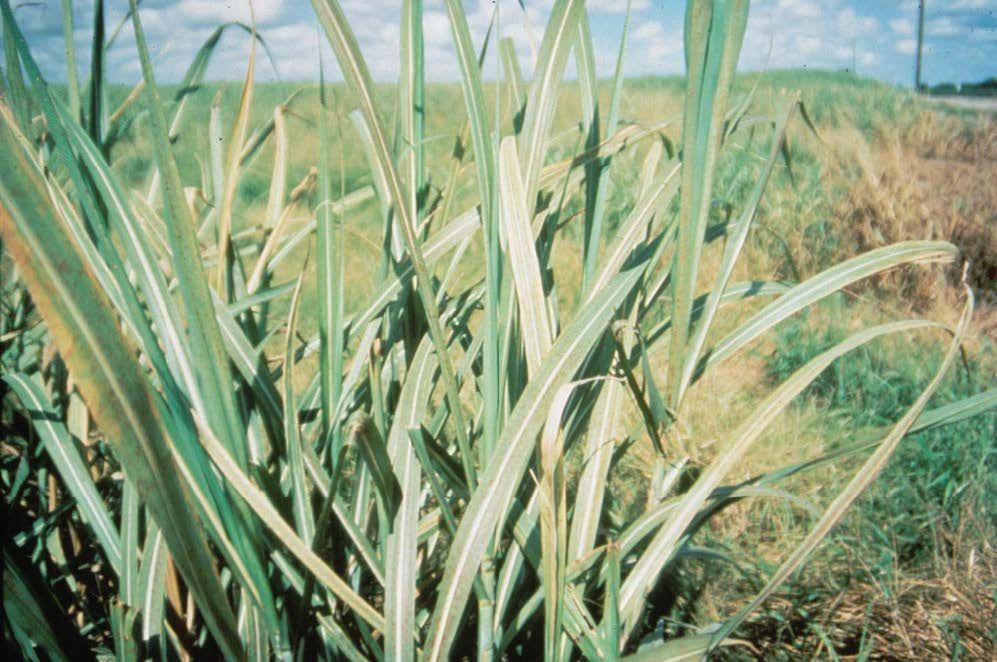
Credit: D. L. Anderson
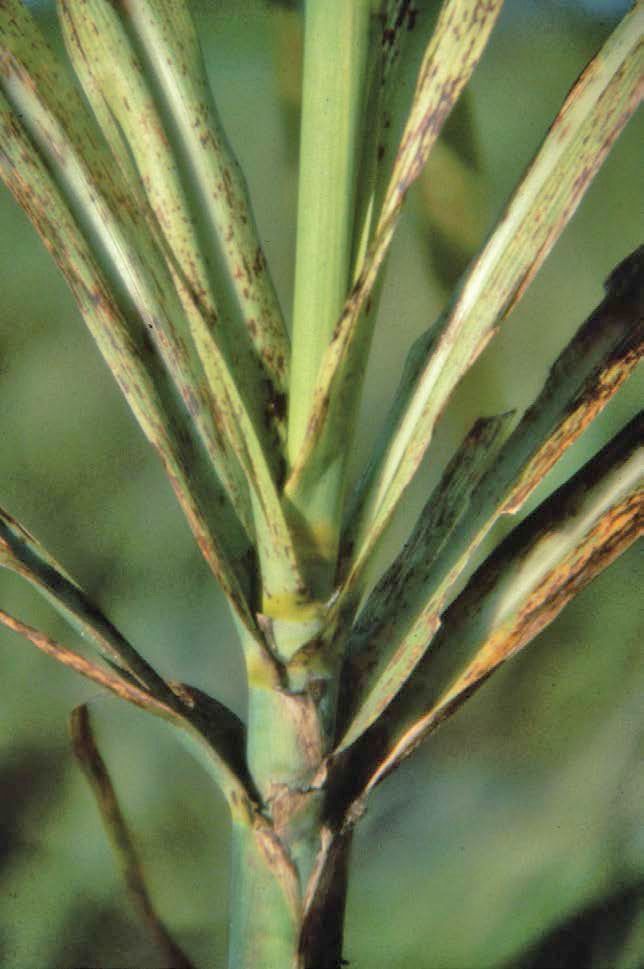
Credit: D. L. Anderson
Manganese (Mn)
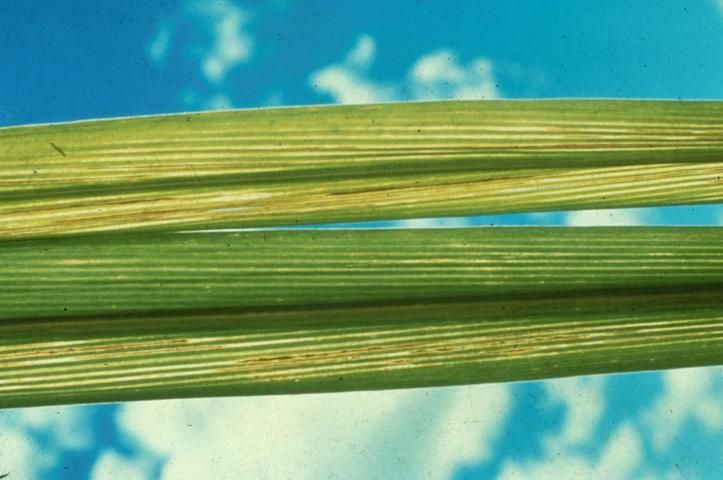
Credit: J. Orlando Filho
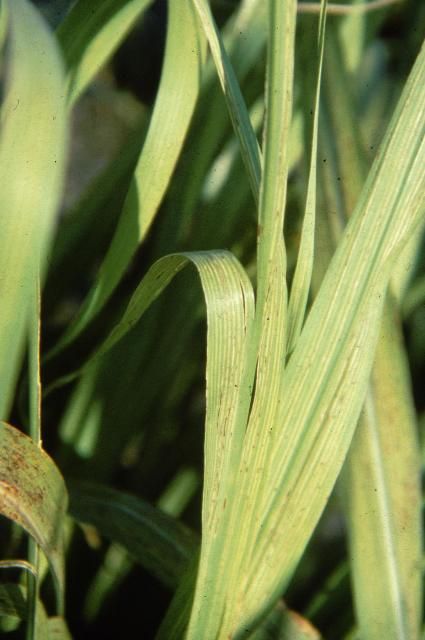
Credit: D. L. Anderson
Molybdenum (Mo)
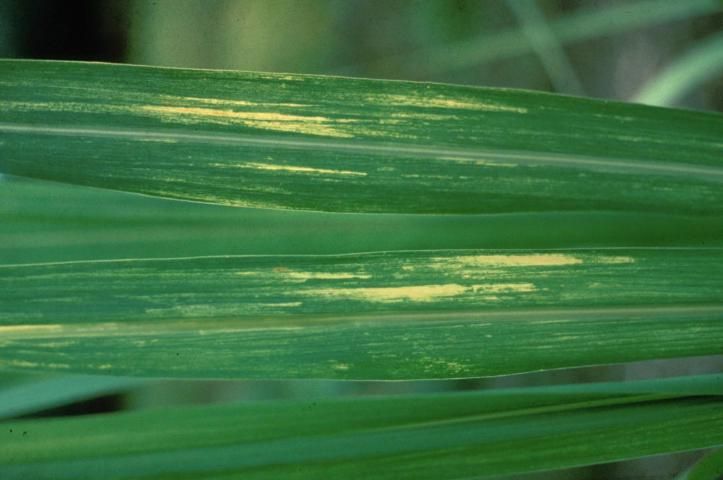
Credit: J. E. Bowen
Nitrogen (N)
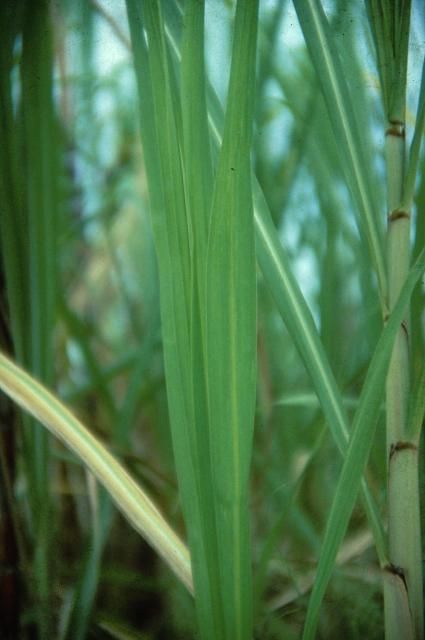
Credit: D. L. Anderson
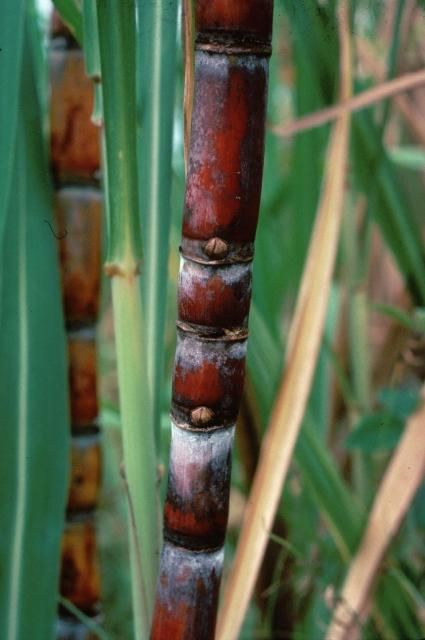
Credit: J. E. Bowen
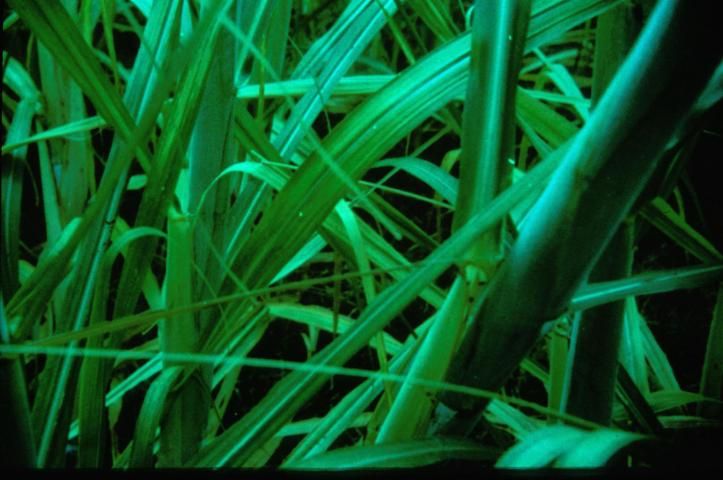
Credit: P. Bosshart
Phosphorus (P)
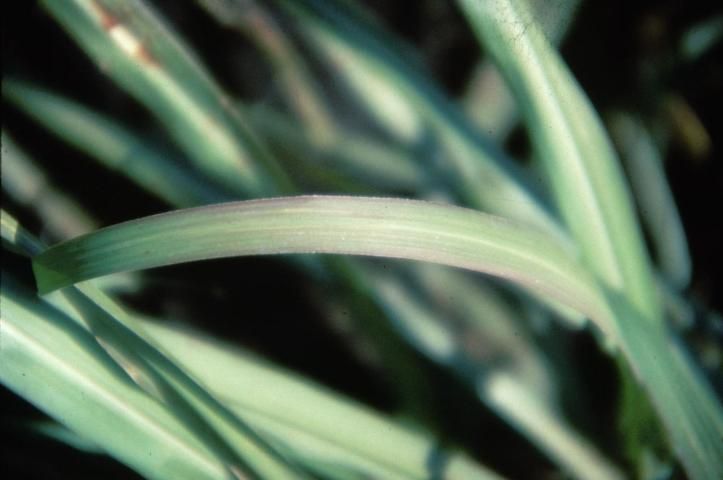
Credit: D. L. Anderson
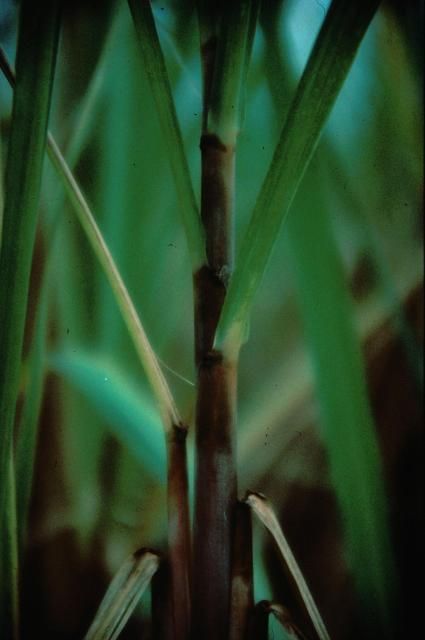
Credit: D. L. Anderson
Potassium (K)
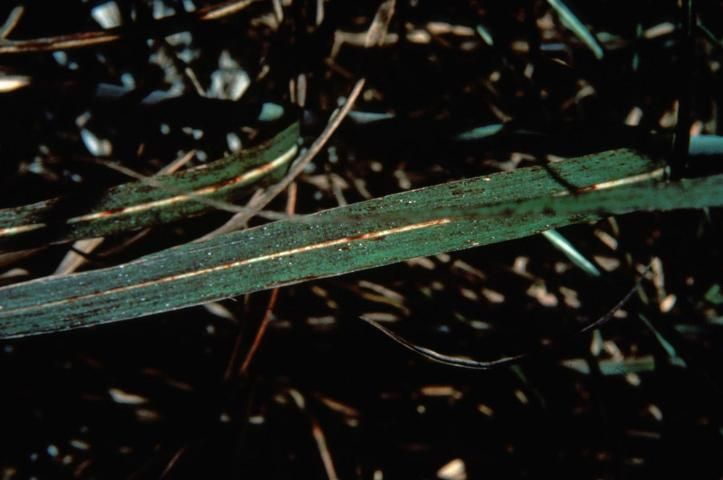
Credit: D. L. Anderson
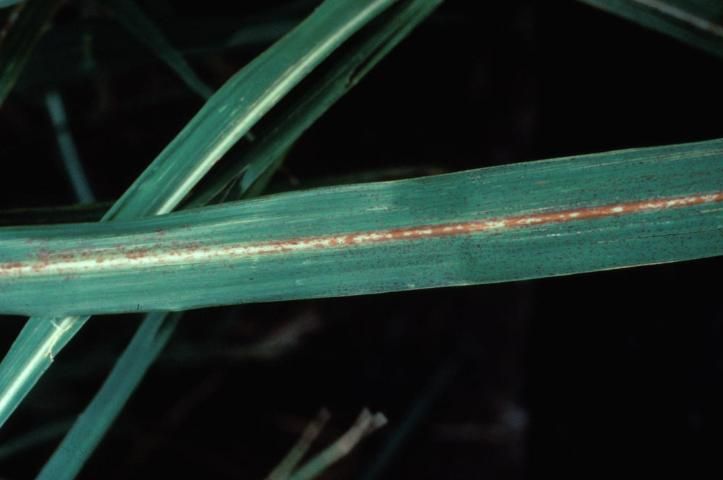
Credit: D. L. Anderson
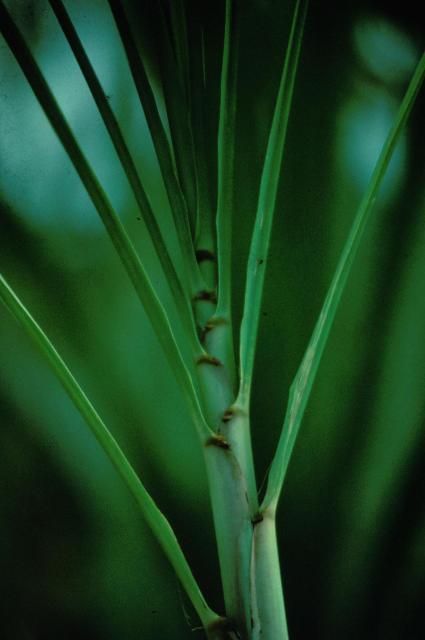
Credit: D. L. Anderson
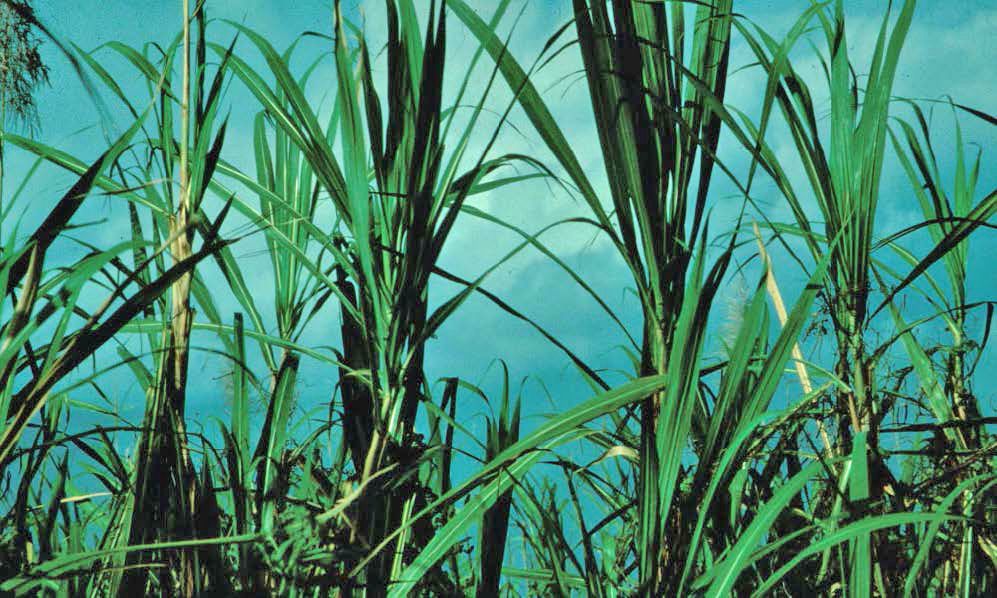
Credit: D. L. Anderson
Sodium (Na)
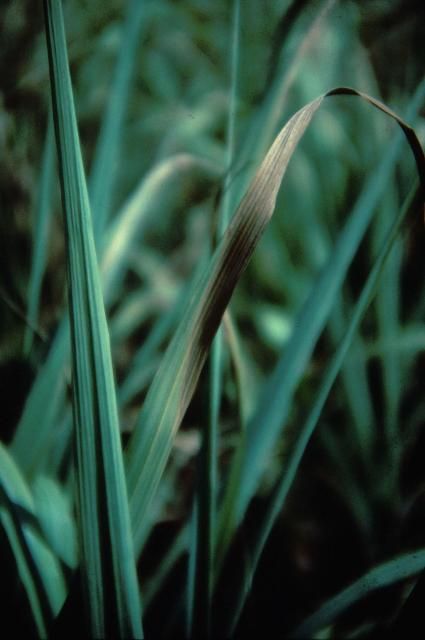
Credit: D. L. Anderson
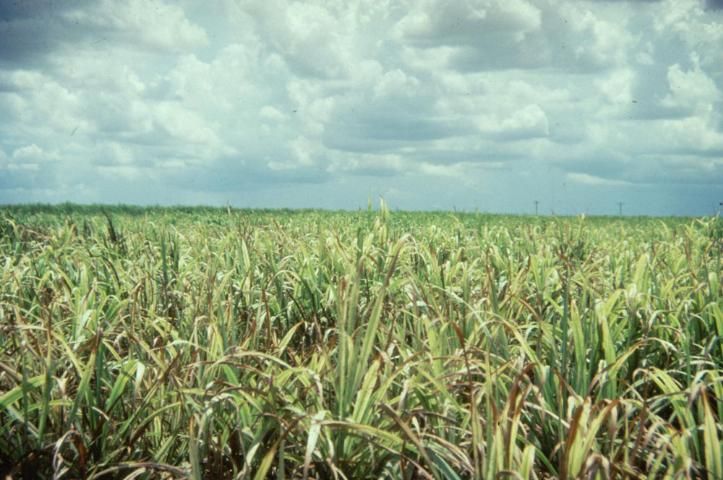
Credit: M. K. Schon
Silicon (Si)
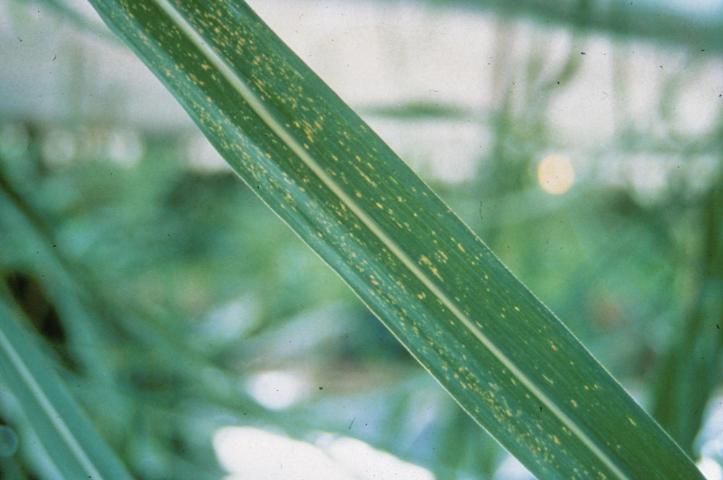
Credit: J. E. Bowen
Sulfur (S)
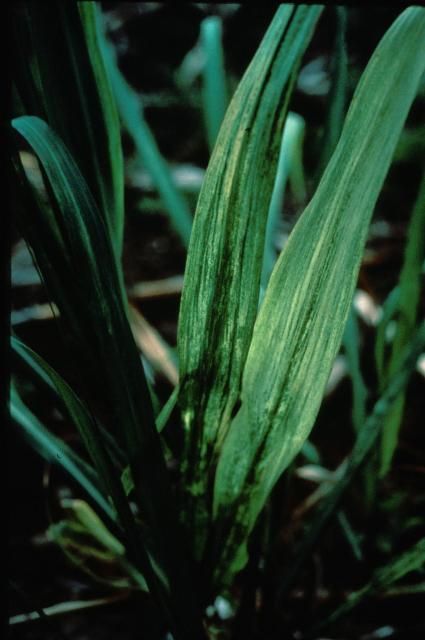
Credit: J. E. Bowen
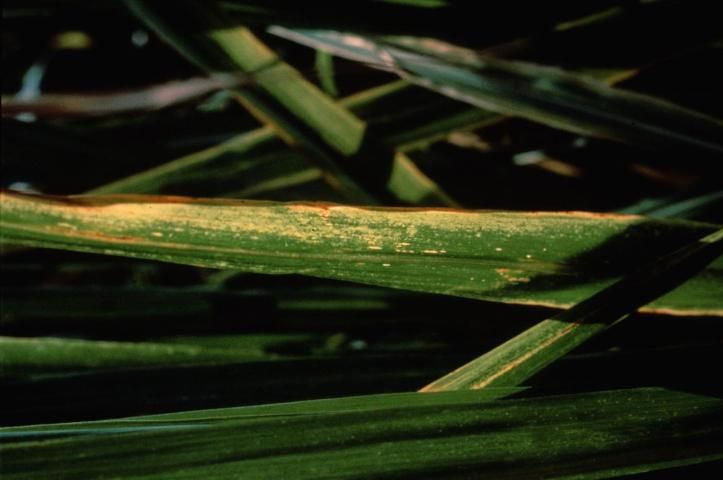
Credit: J. E. Bowen
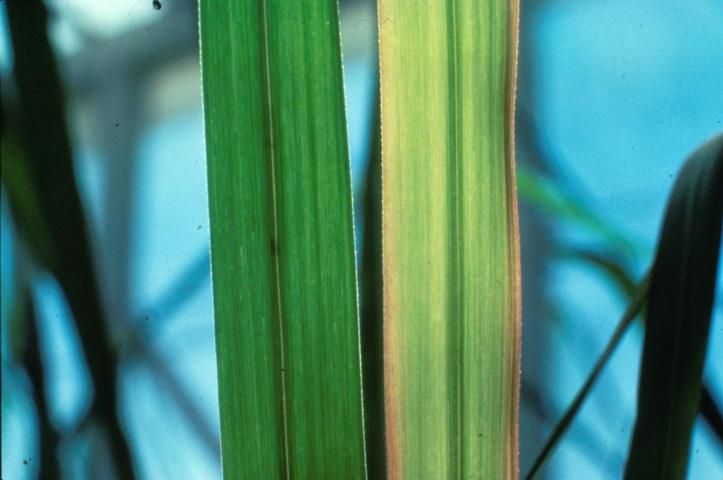
Credit: A. Hurney
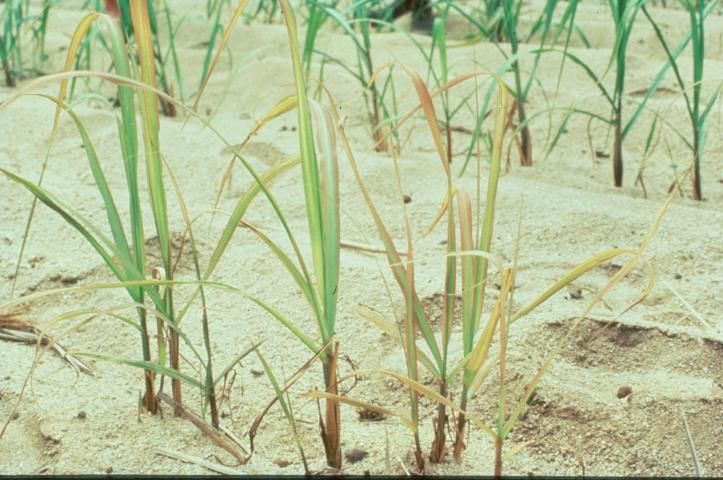
Credit: A. Hurney
Zinc (Zn)
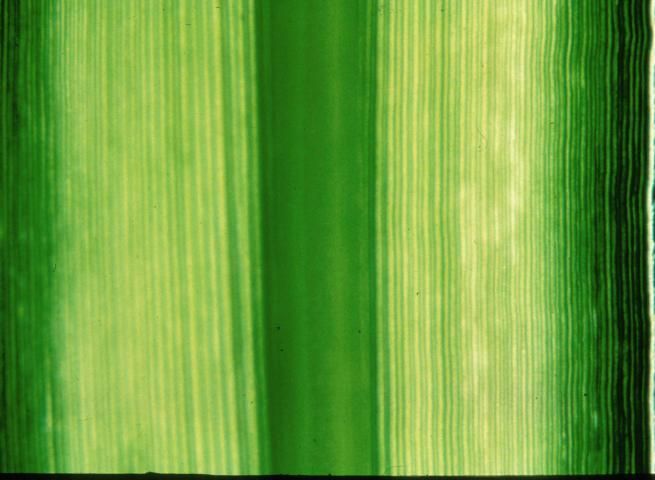
Credit: J. Reghenzani
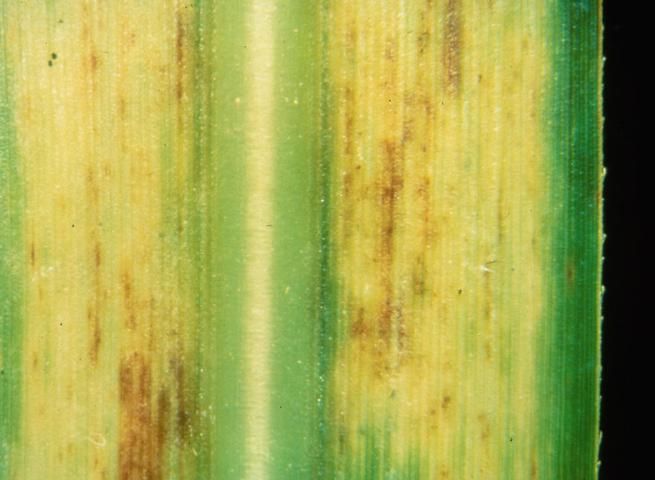
Credit: J. Reghenzani
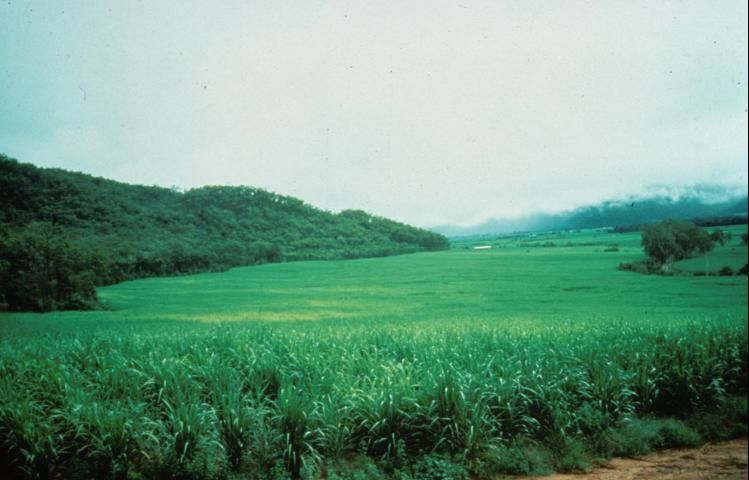
Credit: J. Reghenzani
References and Further Reading
Anderson, D. L. and J. E. Bowen. (1990). Sugarcane Nutrition. Potash and Phosphate Institute, Atlanta, GA.
Beaufils, E. R. (1973). Diagnosis and Recommendation Integrated System (DRIS). A general scheme of experimentation based on principles developed from research in plant nutrition. Soil Sci. Bull. 1, Univ. of Natal, Pietermaritzburg, South Africa. 132 pp.
Elwali, A. M. O. and G. J. Gascho. (1983). Sugarcane response to P, K, and DRIS corrective treatments on Florida Histosols. Agron. J. 75: 79–83. https://doi.org/10.2134/agronj1983.00021962007500010020x
Elwali, A. M. O. and G. J. Gascho. (1984). Soil testing, foliar analysis, and DRIS as guides for sugarcane fertilization. Agron. J. 76: 466–470. https://doi.org/10.2134/agronj1984.00021962007600030024x
McCray, J. M., P. R. Newman, R. W. Rice, and I. V. Ezenwa. (2021). Sugarcane leaf tissue sample preparation for diagnostic analysis. Gainesville: University of Florida Institute of Food and Agricultural Sciences. SS-AGR-259. https://edis.ifas.ufl.edu/sc076
McCray, J. M., S. Ji, G. Powell, G. Montes, and R. Perdomo. (2010). Sugarcane response to DRIS-based fertilizer supplements in Florida. J. Agronomy and Crop Sci. 196: 66–75. https://doi.org/10.1111/j.1439-037x.2009.00395.x
McCray, J. M., and R. Mylavarapu. (2020). Sugarcane nutrient management using leaf analysis. Gainesville: University of Florida Institute of Food and Agricultural Sciences. SS-AGR-335. https://edis.ifas.ufl.edu/ag345