Introduction and Purpose
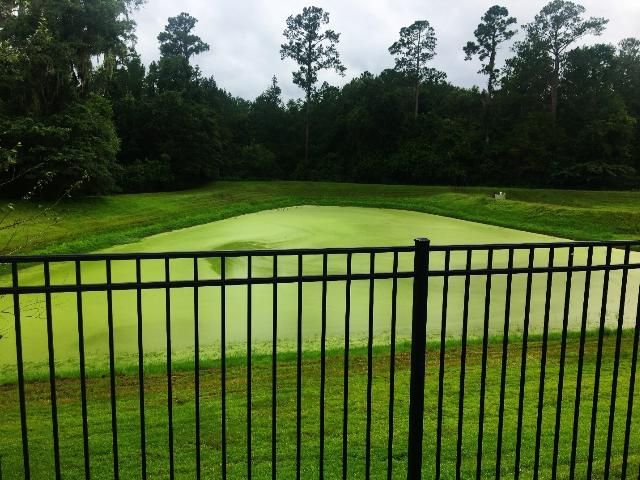
Credit: A. J. Reisinger, UF/IFAS
The majority of the global population currently resides in cities, and this is especially true in Florida. According to the 2010 US Census, more than 90% of Florida residents live in suburban or urban areas. Our increasingly urban world has led to an increased awareness of the importance of urban ecological processes and their potential impacts on natural resources. Urbanization has a particularly large effect on the nitrogen (N) cycle, reducing watershed N retention and increasing downstream N export. Excess N export can lead to eutrophication, with associated algal blooms, and periodic hypoxia in freshwater and coastal ecosystems. These effects are particularly evident in the state of Florida. In fact, through controls on N loading the City of Tampa was able to improve water quality, with subsequent improvements in water clarity and seagrass recovery (Greening et al. 2011). With 80% of Florida's residents living within 10 miles of the coast, Florida's aquatic resources are directly affected by urbanization. For more information on eutrophication and the role of N, see EDIS publication SG118, Rethinking the Role of Nitrogen and Phosphorus in the Eutrophication of Aquatic Ecosystems (https://edis.ifas.ufl.edu/sg118).
Deterioration of coastal ecosystems as a result of increasing urbanization is accelerating. The impact of increased N inputs to coastal waters is far-reaching and affects tourism, recreational activities, and fisheries. Accordingly, regulatory agencies have developed policies to manage N inputs. These policies work to control N loading when the primary source of N is known, but not all loading can be traced to a specific point. For example, wastewater treatment plants are a known point source of pollution, while runoff due to sidewalks and roads is a non-point source. Point sources are known problems with management solutions; a non-point source is more difficult to control due to the diffuse nature of the problem. Although excess N comes from a variety of human activities, urban ecosystems can exhibit both point and non-point sources of N.
Despite urban landscapes representing a source of N to downstream environments, ecosystems within the urban landscape (e.g., lawns, forests, streams, retention basins) can retain and remove N through natural processes (Reisinger et al. 2016). Examples of these processes include N mineralization and immobilization, nitrification, and denitrification (each described in detail below). These processes are carried out by a variety of microorganisms, and each process requires specific environmental conditions. Understanding each process is important to better understand the broader N cycle in urban landscapes and develop potential solutions to N pollution.
This document describes the urban N cycle for state and county regulatory personnel tasked with developing and implementing nutrient management strategies for water quality protection. Readers of this document would benefit from already having a general understanding of nutrient pollution. Although the material covered here can be fairly complex, this document is intended to provide a more in-depth understanding of the N cycle for a reader with a basic understanding but who is looking for more specific details. Ultimately, this document is intended for individuals who work in urban environments and are concerned about nutrient pollution and water quality issues. For more information on N in residential landscapes, see EDIS publication SL254, Nitrogen in the Home Landscape (https://edis.ifas.ufl.edu/ss479).
The Urban Nitrogen Cycle
Nitrogen is a fundamental nutrient required by every living thing on Earth. Ultimately, there are two reasons a plant, animal, or microbe needs N:
- They need N to grow or maintain their cells: N is a fundamental component of life, is required for various biological processes, and is a large component of cellular structures.
- They can obtain energy from the transformation of N from one form to another: Under certain environmental conditions, microbes can convert N between forms to gain energy. This can be thought of in the same way as breathing—just as humans convert oxygen to carbon dioxide and gain energy from this conversion, microbes can "breathe" nitrogen under certain conditions.
Although N is needed by every living thing, it's often in short supply and can be the limiting factor for growth of plants and animals within an ecosystem. Nitrogen naturally cycles between organic and inorganic forms, and many of these transitions are driven by microbial processes. Depending on the source and transformations occurring within the environment, N can exist as either organic or inorganic forms. There are multiple sources of nitrogen within urban environments, including things like atmospheric deposition (nitrogen that enters the environment from the atmosphere via either rainwater or particles [dust]), fertilizer applications, yard waste, and compost (green arrows in Figure 2). Once N is in the urban landscape, there are different paths it can take with differing environmental implications (Hobbie et al. 2017; blue arrows in Figure 2). Below, we will discuss N transformations that can occur in the environment, different N input sources in urban environments, and ultimately how N moves across urban landscapes.
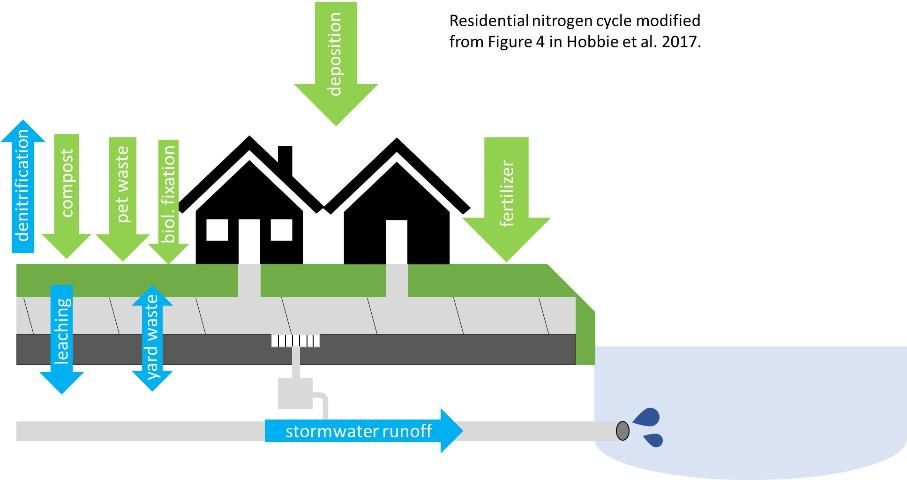
Credit: This figure was modified from Hobbie et al. (2017).
Nitrogen Transformations in Urban Landscapes
Multiple natural processes can change the form of N in urban landscapes. Depending on the process, these changes can either permanently remove N from the system or increase/decrease the potential for N to have environmental impacts. These processes are highly complex, but the descriptions below are intended to give someone without technical expertise a general understanding of different N cycling processes and their impacts on how N moves through urban environments and can be exported to sensitive downstream environments, such as lakes or coastal ecosystems.
Nitrogen fixation—The atmosphere is 78% nitrogen gas (N2), but this gas is not usable by most forms of life because the two nitrogen atoms are held together tightly by a strong triple bond. Nitrogen must be fixed (combined with carbon, hydrogen, or oxygen) before it can be used by organisms. For that nitrogen to become accessible to organisms, the triple bond must be broken. Breaking this triple bond requires a large amount of energy, but it can occur naturally via lightning or biological nitrogen fixation.
Human activity has at least doubled the amount of reactive nitrogen available on Earth, primarily through the production of fertilizer. Fertilizer is produced by a process known as the Haber-Bosch process, which uses tremendous amounts of pressure and energy to break the triple N2 bond and create inorganic N fertilizer from the nitrogen gas in the atmosphere. The discovery of the Haber-Bosch process fueled the green revolution and is largely responsible for the rapidly growing human population. It is estimated that food grown using N fertilizer produced via Haber-Bosch is responsible for feeding approximately 50% of the global population, and as much as 80% of the N found in the average human was produced by the Haber-Bosch process (Smil 2001, Howarth 2008).
Mineralization—Mineralization is the balance of N being used by microbes for growth and N being released into the environment as organisms decompose. This balance controls how much N is available for the growth of plants and microbes. If there is not enough N available, a supplement of N via fertilizer or other N sources (see below) is needed for plant and microbial growth. If there is more N being released than being used by plants and microbes, fertilizer is not necessary, and some of the extra N can be exported to the environment.
Denitrification—Denitrification is a microbial process that converts nitrate (NO3-) into gaseous forms of N. The final product of denitrification is dinitrogen gas (N2), which constitutes 78% of our atmosphere. In addition, there are multiple intermediate products, including nitrous oxide (N2O), a potent greenhouse gas. Denitrification is beneficial if you are concerned with nutrient runoff and pollution because it removes excess nitrogen, which would otherwise fuel algal growth, from the environment. Denitrification can be thought of in the same way as breathing, but microbes are using NO3- instead of oxygen. The microbes that carry out denitrification actually prefer to use oxygen to "breathe," so denitrification only occurs when there is no oxygen in the environment. Denitrification also requires a source of carbon for the microbes to carry out the process. These environmental conditions are commonly found in waterlogged soils or sediments. Adding a carbon source to a stormwater management structure is a common practice to enhance denitrification and remove N to improve downstream water quality.
Ammonia volatilization—Ammonia volatilization is a physical/chemical process that commonly takes place when N is either present in an organic form known as urea or as an ammonium (NH4+) based fertilizer. Urea may originate from manure, organic fertilizers, or the decomposition of organic compounds such as plant material, whereas NH4+-based fertilizers are inorganic fertilizers. Under warm temperature and alkaline soil conditions, urea and NH4+ are rapidly converted to ammonia (NH3) gas, which is subsequently emitted to the atmosphere. As urea is a common source of N in turfgrass fertilizer, volatilization can be a major pathway for N to leave turfgrass landscapes. If you are applying NH4+ or urea-based fertilizers on your landscape, volatilization can be reduced (decreasing the loss of N to the atmosphere) by using a slow-release N source or irrigating your landscape immediately after applying the fertilizer. For more information of volatilization and other N transformations relevant to Florida turfgrass, see EDIS publication ENH1282, The Fate of Nitrogen Applied to Florida Turfgrass (https://edis.ifas.ufl.edu/ep546).
Other N cycling processes—Multiple other biological processes are important components of the N cycle, but these are either less relevant or less well-studied in urban environments and therefore are not the focus of this document. Examples of these additional processes include nitrification, dissimilatory nitrate reduction to ammonium (DNRA), and anaerobic ammonium oxidation (anammox). All together, these processes account for the N cycle. Different components of the N cycle are more or less important in different environments. Regardless of the environment, understanding the biological mechanisms driving N dynamics is crucial for optimizing the beneficial aspects of N in the environment while minimizing potential environmental impacts.
Nitrogen Inputs
As mentioned above, there are multiple ways that N can enter urban landscapes. These inputs include both natural and human-derived sources, although even the natural sources are likely impacted by human activities in urban environments. Depending on the transformations (described above) and transport (described below) of the N, it can have minimal or very large environmental impacts. Just because N comes into urban landscapes via different inputs doesn't mean it will affect downstream areas.
Fertilizer application—Nitrogen-based fertilizers are used in urban landscapes to promote healthy lawns, shrubs, and trees. Fertilizers are used in residential, commercial, industrial, and institutional locations as well as recreational areas requiring green space, such as golf courses or city parks. In Florida, the majority of N-based fertilizers—83% from July 2011 to June 2012—are used for agricultural activities. Of the remaining 17% of N-based fertilizers used for nonfarming activities in Florida, 6% is used for lawn care, 5% for golf courses and athletic fields, and 4% for gardens. For more information on fertilizer usage in Florida, see EDIS publication ENH1277, Florida Fertilizer Usage Statistics (https://edis.ifas.ufl.edu/ep541).
Studies of nitrogen sources in urban landscapes throughout the United States have found that N fertilizer typically represents the dominant N input to urban areas. For example, 53% of N inputs into a suburban neighborhood in Baltimore, MD, were estimated to be from lawn fertilizer (Law et al. 2004). Similarly, 37%–59% of total N inputs into urban watersheds in St. Paul, MN, were from household lawn fertilizer (Hobbie et al. 2017). Although fertilizer may be the major N input into urban watersheds, that does not necessarily mean it is having the largest environmental effect. Although a large amount of N enters urban watersheds as fertilizer N, only a small fraction of this fertilizer moves through the landscape as either leaching or runoff. A large proportion of the fertilizer N is incorporated into plant biomass or soil organic matter pools, with the latter accumulating in the system for years to decades (Raciti et al. 2011). Results from an experiment performed at the University of Florida found little leaching of nitrate (NO3-) through the soil under turfgrass plots regardless of fertilizer application rates (Trenholm et al. 2012), but these results were from ideal experimental conditions. It is less clear how much N is lost from lawns in "real-world conditions." For example, a study of N runoff from residential communities in the Tampa Bay region found that the contribution of N-based fertilizer to N in stormwater runoff was highly variable, ranging from <1%–39% of total N in runoff (Yang and Toor 2017). While it is still unclear how much of the N that is added to a yard as fertilizer can move to other ecosystems and impact environmental quality, following Florida-Friendly Landscaping™ (FFL) recommendations on fertilizer application best practices (i.e., fertilizing only when needed rather than on a routine schedule) to minimize fertilizer use will reduce the inputs of N into urban watersheds. Reducing N inputs should subsequently and reduce the potential for this N to have environmental impacts. For more information on Florida Friendly Landscaping™, visit the EDIS Florida-Friendly Landscaping™ topic page at https://edis.ifas.ufl.edu/topic_ffl.
Atmospheric deposition—As described above, the atmosphere of the Earth is 78% N gas (N2), but this gas is not usable by most forms of life because the two N atoms are held together tightly by a triple bond. However, there are also trace amounts of other forms of nitrogen in the atmosphere, including ammonia, nitrate, and nitrogen oxides. These molecules interact with water vapor in the atmosphere, so when it rains, there is N dissolved in the rainwater. In addition, there are tiny dust particles floating in the atmosphere with various chemicals associated with them. Some of these particles contain N. As these particles settle to the ground, the N associated with them enters the urban landscape too. Finally, lightning in the atmosphere has enough energy that it can break the triple bond of N2 gas, converting it into nitrate that then dissolves in rainwater and falls to the Earth. The process of lightning converting N2 gas into nitrate is a form of nitrogen fixation, but N transformed through this process will reach urban environments via atmospheric deposition.
Although the overall concentrations of N in the atmosphere and in rainwater are fairly low, atmospheric deposition can represent a significant proportion of the total N moving through urban areas. In fact, atmospheric deposition accounted for 19%–34% of total N inputs into urban watersheds in Minnesota, second only to household fertilizer sources (Hobbie et al. 2017). Furthermore, as atmospheric deposition occurs primarily during periods when water is running off the landscape, such as during the wet season, it can be responsible for a large proportion of the total N exported during storm events. In fact, atmospheric deposition was the largest source of NO3- in stormwater runoff from a Tampa Bay community, accounting for 35%–64% of total NO3- found within stormwater (Yang and Toor 2017).
Pet waste—As urban developments give rise to increased housing density, the number of pets per area is increasing in urban areas. Pet feces exhibit high concentrations of N. This large amount of N in pet waste coupled with the increasing concentration of pets in urban areas and the estimate that 40% of pet waste ends up in the landscape (Fissore et al. 2011) means that pet waste can be a significant contributor of N inputs in urban watersheds, particularly the more residential/suburban watersheds. In fact, Hobbie et al. (2017) estimate that 28% of total N inputs into St. Paul, MN, watersheds are from pet waste. This goes to show how important it is to "scoop the poop."
Organic debris—Grass clippings and leaf litter are not external N sources to a watershed and do not represent new N entering a system. However, there is a substantial amount of N within plant biomass, and these sources of N can be mobilized into urban stormwater runoff when they collect on impervious surfaces. Organic debris can leach organic N and ammonium as the debris interacts with stormwater, thus becoming a source of stormwater N enrichment. The extent that these materials contribute N to stormwater will depend on neighborhood management practices, such as whether or not grass clippings are kept off of streets and sidewalks or if local street sweeping occurs after leaves fall from trees. A UF/IFAS study showed that total N in summer rainy season stormwater was 3–4 times higher in a neighborhood that had leaf litter accumulated in the streets compared to a neighborhood without leaf litter accumulation (Liao, Lusk et al. unpublished data). In a separate study in a residential neighborhood in the Tampa Bay, Florida, area, particulate organic N was often the dominant N form in summer stormwater runoff, originating from oak leaves and acorns (76% of particulate organic N) and St. Augustine grass clippings (24% of particulate organic N) (Lusk et al. In Press).
Other sources—Other potential sources of N in urban landscapes are often considered to be minimal but include biological nitrogen fixation or compost. Some microbial and plant species are capable of converting atmospheric N2 gas into N available through growth via the process of N fixation. Perennial peanut (Arachis glabrata) is an example of a plant commonly used in Florida landscapes that can fix nitrogen. Overall, N fixation appears to be a minimal source of N in urban watersheds, but under certain conditions—for example, if a neighborhood uses nitrogen-fixing species such as perennial peanut as a ground cover—it could be more impactful than in areas without large amounts of N-fixing species (Hobbie et al. 2017). Although N-fixation represents an N input into the urban environment, much of this N remains within plant tissue or soils for longer periods of time and may not move through the urban watershed.
Reclaimed water used for irrigation can also be a source of N to urban watersheds. Though a statewide accounting of how much N is added to urban landscapes via reclaimed water irrigation has not been conducted, we know that reclaimed water can contain higher concentrations of N than potable water. When irrigation exceeds the demands of the plants, or if sprinkler heads unintentionally apply water to sidewalks or streets, the N in reclaimed water may become a component of urban stormwater. For more information about nutrients in reclaimed water, see EDIS publication SL337, Reclaimed Water Use in the Landscape: What's in Reclaimed Water and Where Does It Go? (https://edis.ifas.ufl.edu/ss542).
Compost is another potential source of N for urban watersheds. Compost is increasingly being used to improve the soil health of residential landscapes. Although compost materials differ based on the composting process and the source materials, in general compost will provide a source of organic matter to soils while also contributing organic nutrients and a microbial community that can improve soil health. Depending upon the source of the composted materials, this N may or may not be an external N source to the watershed. For example, if the compost you apply on your yard was created from grass clippings and food wastes from within your community, there is no additional N entering the system. Rather, this N that would otherwise be a waste material has been repurposed for an additional beneficial use. The relative importance of compost as a N source for an urban watershed will depend on the amount of compost used in a watershed and the materials used to produce the compost.
Nitrogen Transport in Urban Landscapes
Nitrogen can have positive or negative impacts on the landscape depending on when, where, and how it is used/applied. When applied correctly, N fertilizer can provide lush landscapes that improve our social well-being and feed our families. When applied incorrectly, N can fuel algal growth and cause major issues in surface-water quality. One factor driving the relative impacts of N on the landscape is how long N remains in a given location, or how quickly it is transported to another environment.
In any landscape, nitrogen can either percolate into the soil profile with rain water, potentially leaching into groundwater aquifers, or it can run off the landscapes into surface water ecosystems. In urban areas, increased impervious surfaces (i.e., buildings, roads, and sidewalks) limit the amount of water that moves through the soil profile, instead increasing the amount of water (and associated nutrients) that run off the surfaces. This increase in runoff is typically dealt with using various stormwater infrastructure, including things like stormwater ponds, constructed wetlands, swales, or conservation areas. As water moves through these different pathways, N and other pollutants move with the water. N can leach through the soil profile, can move through stormwater pipes, or can directly run off into surface waters. Reducing the amount of N that moves across the landscape and maximizing the processes that remove N during transport are essential for mitigating the impact of N from urban landscapes.
Summary and Conclusions
This document is intended to provide a broad overview of the complexities of the nitrogen cycle, with a particular focus on urban environments. Although this document is not intended to make the reader an expert, the information included will provide individuals with a general knowledge of different sources of N in urban landscapes as well as the various processes and terminologies associated with nitrogen cycling. Various management practices can be implemented to minimize our impacts on the nitrogen cycle. More information can be found related to nitrogen in urban environments in EDIS publications SL254, Nitrogen in the Home Landscape (https://edis.ifas.ufl.edu/ss479), and ENH979, Homeowner Best Management Practices for the Home Lawn (https://edis.ifas.ufl.edu/ep236).
A variety of water quality issues are currently facing the state of Florida, and nutrient pollution is suspected or known to be at least one of the causes of many of these issues. It is important to understand that there are multiple different sources of N in urban landscapes and that multiple processes occur in the urban environment that can potentially retain, remove, or transform N prior to its export downstream to sensitive water bodies or leaching into groundwater. By understanding the sources and transformations of N in urban landscapes, we hope that this document will lead to changes in our actions that may contribute to N pollution coming from urban areas.
References
Fissore, C., L. A. Baker, S. E. Hobbie, J. Y. King, J. P. McFadden, K. C. Nelson, and I. Jakobsdottir. 2011. "Carbon, Nitrogen, and Phosphorus Fluxes in Household Ecosystems in the Minneapolis-Saint Paul, Minnesota, Urban Region." Ecological Applications 21: 619–639. https://doi.org/10.1890/10-0386.1
Greening, H. S., L. M. Cross, and E. T. Sherwood. 2011. "A Multiscale Approach to Seagrass Recovery in Tampa Bay, Florida." Ecological Restoration 29: 82–93. https://doi.org/10.3368/er.29.1-2.82
Hobbie, S. E., J. C. Finlay, B. D. Janke, D. A. Nidzgorski, D. B. Millet, and L. A. Baker. 2017. "Contrasting Nitrogen and Phosphorus Budgets in Urban Watersheds and Implications for Managing Urban Water Pollution." Proceedings of the National Academy of Sciences of the United States of America 114: E4116. https://doi.org/10.1073/pnas.1618536114
Howarth, R. W. 2008. "Coastal Nitrogen Pollution: A Review of Sources and Trends Globally and Regionally." Harmful Algae 8: 14–20. https://doi.org/10.1016/j.hal.2008.08.015
Law, N. L., L. E. Band, and J. M. Grove. 2004. "Nitrogen Input from Residential Lawn Care Practices in Suburban Watersheds in Baltimore County, MD." Journal of Environmental Planning and Management 47: 737–755. https://doi.org/10.1080/0964056042000274452
Raciti, S. M., P. M. Groffman, J. C. Jenkins, R. V. Pouyat, T. J. Fahey, S. T. A. Pickett, and M. L. Cadenasso. 2011. "Accumulation of Carbon and Nitrogen in Residential Soils with Different Land-Use Histories." Ecosystems 14: 287–297. https://doi.org/10.1007/s10021-010-9409-3
Reisinger, A. J., P. M. Groffman, and E. J. Rosi-Marshall. 2016. "Nitrogen Cycling Process Rates across Urban Ecosystems." FEMS Microbiology Ecology 92 (12): fiw198. https://doi.org/10.1093/femsec/fiw198
Trenholm, L. E., J. B. Unruh, and J. B. Sartain. 2012. "Nitrate Leaching and Turf Quality in Established 'Floratam' St. Augustinegrass and 'Empire' Zoysiagrass." Journal of Environment Quality 41: 793. https://doi.org/10.2134/jeq2011.0183
Yang, Y. Y., and G. S. Toor. 2017. "Sources and Mechanisms of Nitrate and Orthophosphate Transport in Urban Stormwater Runoff from Residential Catchments." Water Research 112: 176–184. https://doi.org/10.1016/j.watres.2017.01.039