Overview
Nitrogen is natural and necessary, yet nitrogen levels above natural levels can cause algal blooms and eutrophication of coastal systems. Bivalve shellfish, including oysters (Crassostrea virginica), can help mitigate the effects of too much nitrogen through filtration, accumulation of nitrogen in their shells and tissues, and enhancement of sediment denitrification. Denitrification is a natural process that converts nitrogen that can be used by algae, like nitrate (NO3-), to harmless dinitrogen gas (N2). Strategies to remove nitrogen and improve degraded water quality are multifaceted, and impacts vary depending on the system’s location, hydrology, and nitrogen load. Restoration and aquaculture of bivalve shellfish, like oysters, represent one potential tool for reducing nitrogen and improving water quality in coastal systems. The purpose of this document is to describe how oysters and oyster reefs remove nitrogen. Because oysters build reef structure, which traps organic matter and serves as habitat for other organisms, they may have a different effect on nitrogen removal than other shellfish. The document is intended for policymakers, environmental organizations, and coastal residents who want to know about oysters' role in improving water quality through nitrogen removal.
Nitrogen Pollution and Eutrophication
Nitrogen (N) pollution is one of the most challenging environmental problems facing coastal waterbodies. Nitrogen is essential for life, but land-based fertilizer use has at least doubled the amount of nitrogen available to organisms on earth (Vitousek et al. 1997). At the same time, human development has decreased the filtration capacity of the land through the conversion of wetlands and increased impervious surfaces, thus increasing nitrogen inputs into the watershed through stormwater runoff. As a result, coastal waterbodies often have too much nitrogen. An overabundance of nitrogen can lead to excessive algal growth, a process known as eutrophication. The algae can “bloom” (for a detailed description of what constitutes an algal bloom, see EDIS TP-231), which may block sunlight and negatively impact seagrass and other marine organisms. Algal blooms are often followed by a period of decomposition when the high densities of algal cells begin to die and decay. The microbial process of decomposition consumes oxygen, which in turn causes low oxygen or, in extreme cases, no oxygen, leading to “dead zones.” While toxins in the algae sometimes kill marine life, many fish kills associated with an algal bloom are a consequence of the fish suffocating within these dead zones due to a lack of oxygen.
In Florida, the prevalence and intensity of harmful algal blooms are increasing (EDIS SGEF216), and over 300 miles of the state’s 1,539 miles of coastal waters exceed the state recommended amount of nitrogen (Wade and Borisova 2022), illustrating the widespread problem nitrogen pollution has on Florida’s waterways.
How do oysters remove nitrogen?
Oysters filter large amounts of water every day. Under controlled laboratory conditions, an individual adult oyster can filter over 40 gallons of water per day. However, the filtration rate may vary widely in the wild and filter 3.0–12.5 gallons of water (Gray et al. 2021; Ehrich and Harris 2015). Filtration removes sediment, algae, and other particles suspended in the water, resulting in clearer and cleaner water (Figure 1a). Because oysters are filter feeders, removing algae and organic matter from the water, they have the potential to remove, process, and absorb nitrogen that would otherwise contribute to eutrophication.
Oysters can remove nitrogen by incorporating nitrogen in algae and other filtered particles into their shell and tissue (Figure 1b). This process of assimilation would store nitrogen for a while until the oyster dies and decomposes. Nitrogen in the oyster shell and tissue is a long-term nitrogen sink if the oyster is harvested and removed from the marine environment, such as in an aquaculture context. The harvesting of oysters and associated nitrogen removal is called bioextraction (Figure 1c).
Material not incorporated into the oyster tissue or shell is rejected as waste and released back into the environment. Oysters excrete nitrogen waste as ammonium and small amounts of urea and amino acids, which algae can use to grow in the water column (Dame, Zingmark, and Haskin 1984; Dame, Spurrier, and Wolaver 1989). Oysters also produce biodeposits. Biodeposits are feces (digested material) and pseudofeces (undigested material but expelled) that contain nitrogen along with other waste products (Figure 1d). Biodeposits are heavy and sink to the sediments near oysters. The oyster reef structure, or on-bottom aquaculture gear, helps concentrate and collect biodeposits. Over time, biodeposits may be buried in the sediment, resulting in another way to store nitrogen and prevent it from being used by algae (Figure 1e).
Biodeposits that settle on the sediment surface can also stimulate denitrification (Newell, Cornwell, and Owens 2002; Smyth, Geraldi, and Piehler 2013). Denitrification is a natural way to remove bioavailable or reactive nitrogen from the environment. Denitrification is the microbial transformation of nitrogen forms that can be used by algae, such as nitrate (NO3-), to harmless dinitrogen gas (N2) (Figure 1f). Oyster reefs and oyster aquaculture help to enhance sediment denitrification because of the production and concentration of oyster biodeposits (Figure 2A; Smyth et al. 2016; Ray and Fulweiler 2020). While sediment denitrification can occur without oysters (Figure 2B), the accumulation of biodeposits leads to areas with oysters having higher denitrification rates than areas without oysters (Figure 2B; Kellogg et al. 2014). In addition to the presence and active filtration by live oysters, the physical structure of the reef and reef-associated organisms contribute to the high rates of denitrification associated with oyster reef ecosystems (Kellogg et al. 2013; Smyth et al. 2016). Oyster reefs reduce flow, helping to concentrate biodeposits. Further, reefs act as a habitat, and other organisms may also increase organic matter deposition onto the sea floor. This reef structure, which traps organic matter and serves as a habitat for other organisms, is one reason why oysters may have a different effect on nitrogen removal than other shellfish species (Smyth et al. 2018).
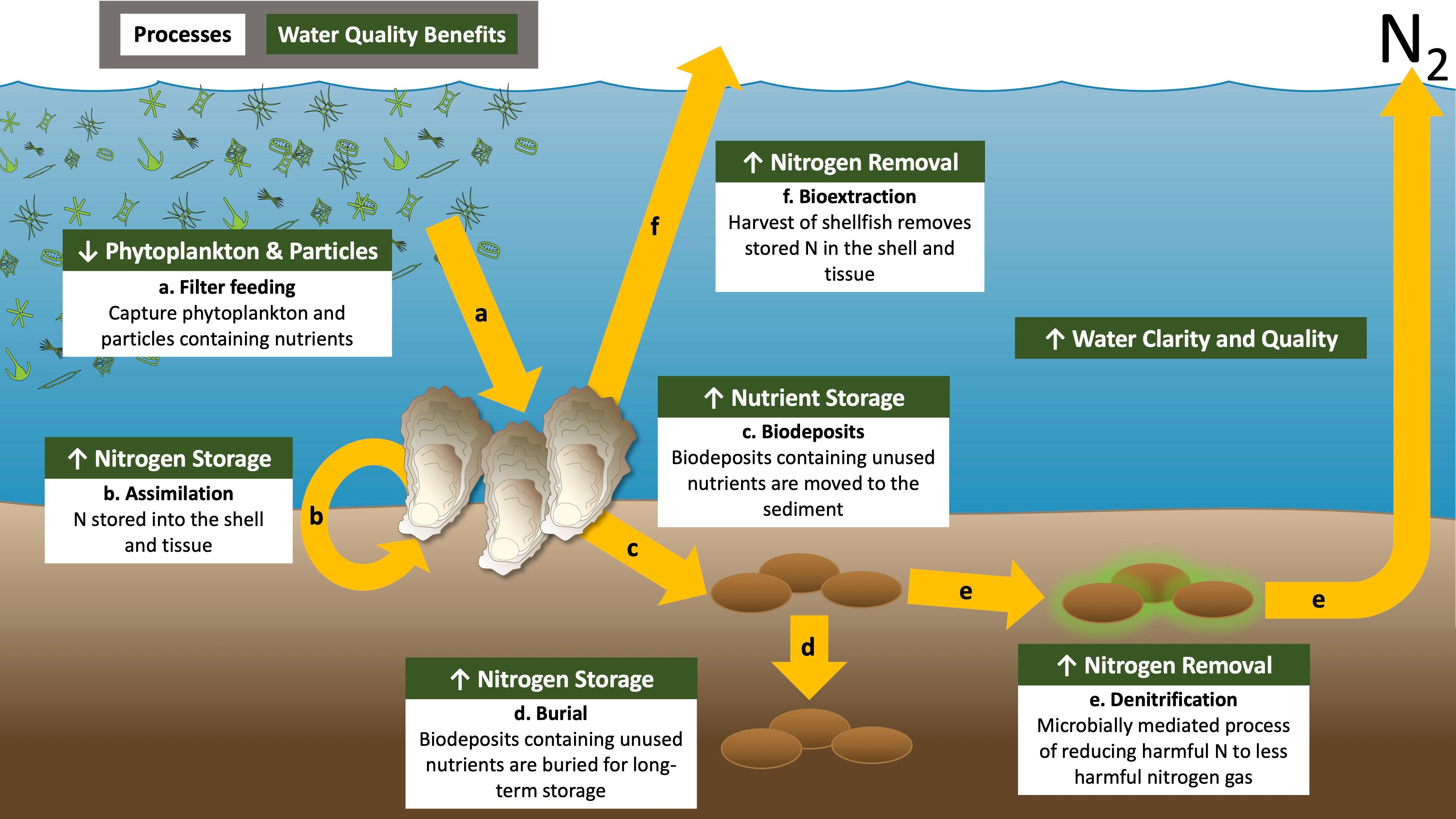
Credit: Modified from Kreeger, Gatenby, and Bergstrom (2018). Symbols courtesy of the Integration and Application Network (ian.umces.edu/symbols/), University of Maryland Center for Environmental Science.
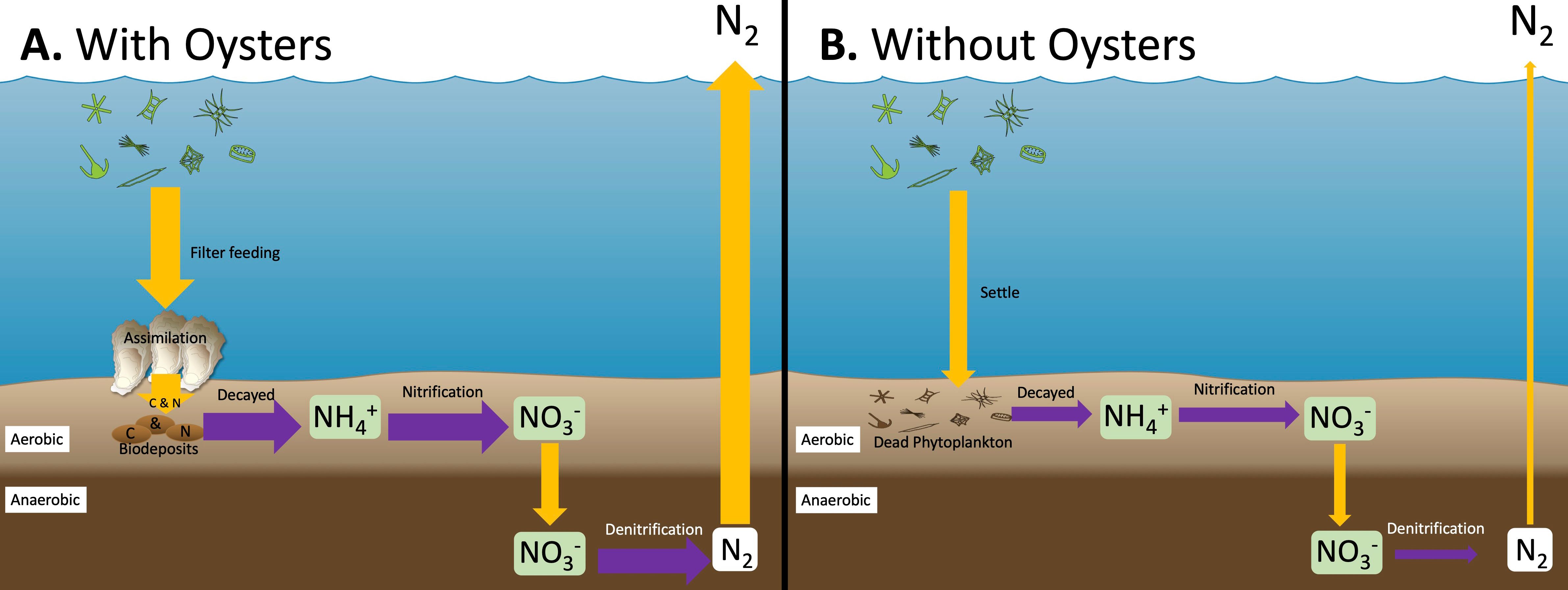
Before nitrogen contained in biodeposits can be removed via denitrification, a series of other reactions occur. First, the biodeposits are decomposed by microbes, resulting in the production of ammonium (NH4+). Ammonium is a form of nitrogen that is used by algae. When oxygen is present, the microbial process of nitrification transforms the ammonium into nitrate. Nitrate and ammonium are both forms of nitrogen that contribute to eutrophication. Unless conditions are right for denitrification, this nitrogen will return to the water column, where it can fuel algal growth. The transformation of nitrate to unusable N2 gas occurs only when sediment oxygen is low, and there is a supply of organic material, like biodeposits. Denitrification is microbial respiration where nitrate is used to break down organic matter for energy instead of oxygen. Microbes that perform denitrification prefer to use oxygen then nitrate to respire because it gives them more energy. Nitrate used in denitrification can come directly from the water column or nitrification. For more information on the nitrogen cycle, see EDIS SL471. While oysters are filtering and helping to remove nitrogen as N2 gas, they also produce nitrogen in the form of ammonium through waste products and the breakdown of the biodeposits. As a result, oysters and oyster reefs are not 100% efficient at removing nitrogen. Because nitrogen is essential for life, some nitrogen must remain in the system, and any denitrification increase is expected to decrease total nitrogen cycling within the system.
How much nitrogen can oysters remove?
Oysters remove nitrogen directly through assimilation into biomass and indirectly through burial and enhanced denitrification. Estuary-specific conditions, including salinity, temperature, hydrology, flow, and food availability, affect oysters' clearance and growth rates, thereby influencing their ability to cycle and remove nitrogen (Newell 2004). Due to local differences, it is hard to provide a single estimate of exactly how much nitrogen an oyster or an oyster reef can remove. Site-specific measurements are necessary to determine the efficacy of oyster reef restoration or aquaculture as a method of nitrogen reduction in coastal waters.
One method to assess the net effect of sediments on system-wide biogeochemistry is to assess denitrification efficiency. Denitrification efficiency measures the likelihood that bioavailable nitrogen will be transformed into nitrogen gas via denitrification and removed. Denitrification efficiency at oyster reefs can range from 20% to 100% (Piehler and Smyth 2011; Ray, Henning, and Fulweiler 2019). Denitrification efficiency is calculated as the amount of N2 production (denitrification) relative to the total inorganic nitrogen production and expressed as a percent. Efficiency greater than 50% indicates that it is more likely than not that nitrogen will be removed by denitrification. Any denitrification efficiency greater than 0% means some nitrogen was transformed into N2 gas and removed; the higher the efficiency, the greater the chances are that more nitrogen will be removed rather than recycled or remain in the system. The range in percentages means that some oyster sites are good at removing nitrogen while some are better at recycling nitrogen and returning useable nitrogen to the water column. While nitrogen removal can vary, a recent review found that the effect (difference from what is naturally occurring) of oyster ecosystems on sediment denitrification was similar across all sites and regardless of whether data were collected from oyster reefs or aquaculture, even though the actual rate of denitrification was different among sites (Ray and Fulweiler 2020).
While denitrification is highly variable, nitrogen content in shell and tissue is more stable. A review found that oyster tissue and shell nitrogen contents were similar in estuaries from Virginia to New Hampshire. On average, oyster tissue contained 8.2% nitrogen, and oyster shell contained 0.2% nitrogen (Cornwell et al. 2016). Because shell morphology and tissue weight determine nitrogen content and this value can vary, there is a large range in nitrogen stored in oysters and oyster reefs. Each location where oysters are grown has unique conditions, which can lead to variabilities in nitrogen removal processes.
Nitrogen removal through harvest (bioextraction) tends to exceed denitrification annually. For example, at an aquaculture farm in Virginia, harvest accounted for 90% of the total nitrogen removal at the farm (Lunstrum, McGlathery, and Smyth 2018). For restored reefs in New Hampshire, sequestration into shells accounted for 91% of the nitrogen removal attributed to the presence of the oyster reef (Bricker et al. 2019). Yet a study in Harris Creek, Virginia, found that denitrification was responsible for 73% of the nitrogen removal from restored reefs (Kellogg, Brush, and Cornwell 2018).
Researchers have been able to scale up measurements of nitrogen removal by reefs and aquaculture to determine the amount of the nitrogen load to the water body that oysters could remove. When those measurements have been conducted, oysters can remove anywhere from less than 1% to 15% of the nitrogen load to the waterbody (Carmichael, Walton, and Clark 2012; Smyth, Thompson, et al. 2013; Dvarskas et al. 2020). Restoration of oyster reefs can increase nitrogen removal. For example, in the Choptank River, Maryland, if oysters occupied all area suitable for oysters, oyster-mediated nitrogen removal would remove 48% of the nitrogen load to the river (Kellogg et al. 2014). However, the scale of the project required to reach desired N reduction targets is not always feasible, and the exact numbers of the area and oyster-mediated nitrogen removal will be site-specific. Assessing whether oysters are a viable means of mitigating nitrogen pollution in a particular estuarine environment will rely heavily on site-specific measurements and characteristics.
Factors That Influence the Ability of Oysters to Remove Nitrogen
It is essential to recognize the limitations of using oyster reef restoration and oyster aquaculture to meet nitrogen removal goals. Though conditions of various water bodies may be suitable for oysters, there is no guarantee that oysters will survive there, let alone remove appreciable amounts of nitrogen. Additionally, maximum benefits for nitrogen removal will occur when live oysters are harvested because the nitrogen stored in the shell and tissue represents a more considerable amount of nitrogen than the nitrogen removed through denitrification. However, it is often the case that areas where nitrogen loads are highest are usually also closed for shellfish harvest. Under these conditions, nitrogen removal by adding oysters would only be attributed to denitrification because the oysters would need to be left in place. However, it is critical to remember that oysters provide many other benefits, so whether their total N removal is high or low, they still provide valuable ecosystem services such as filtration and habitat creation (Grabowski et al. 2012). Oyster survival and subsequent environmental benefits, including nitrogen removal, depend on a variety of different factors, such as:
Conditions for oyster growth: Not all locations will have suitable conditions for oysters based on salinity, temperature, and flow (Rose et al. 2021).
Water Quality: While oysters can improve water quality, they also need sufficiently good water quality to live and grow. For example, extended low-oxygen events can result in oyster death. Areas with high bacterial counts will prohibit the harvest of oysters for consumption, so nitrogen removal via bioextraction, which tends to be the biggest nitrogen removal term, cannot occur if the removal of the oysters does not happen (Rose et al. 2021).
Food: Oysters also need ample food supply (phytoplankton/algae) to thrive. If there are no phytoplankton/algae, then oysters cannot facilitate the removal of nitrogen from the water because they remove the nitrogen that is initially contained in the algae. Limited food supply would limit the nitrogen removal services oysters provide through filter-feeding (Newell 2004).
Oyster Density: The oyster effects on denitrification rates are density-dependent. Extremely high oyster densities can increase total biodeposition. The accumulation in oyster biodeposits will lead to an overall decrease in sediment denitrification because heavy biodeposit and organic matter accumulation can lead to low-oxygen zones and the collection of toxins, like sulfide, that limit denitrification and enhance the recycling of nitrogen back to the water column (Figure 3; Newell 2004). Though unsuitable environmental conditions (i.e., low oxygen) and extreme oyster densities can decrease nitrogen removal efficiency, oysters can still remove nitrogen and improve water quality at low to moderate densities.
Carrying Capacity: Too many oysters (i.e., extreme densities) may exceed the carrying capacity, meaning there is insufficient food to support the oyster population (Newell 2004). Extreme oyster densities can cause competition for food (Figure 3). Competition for food when oyster densities are extreme can limit oyster growth (Newell 2004).
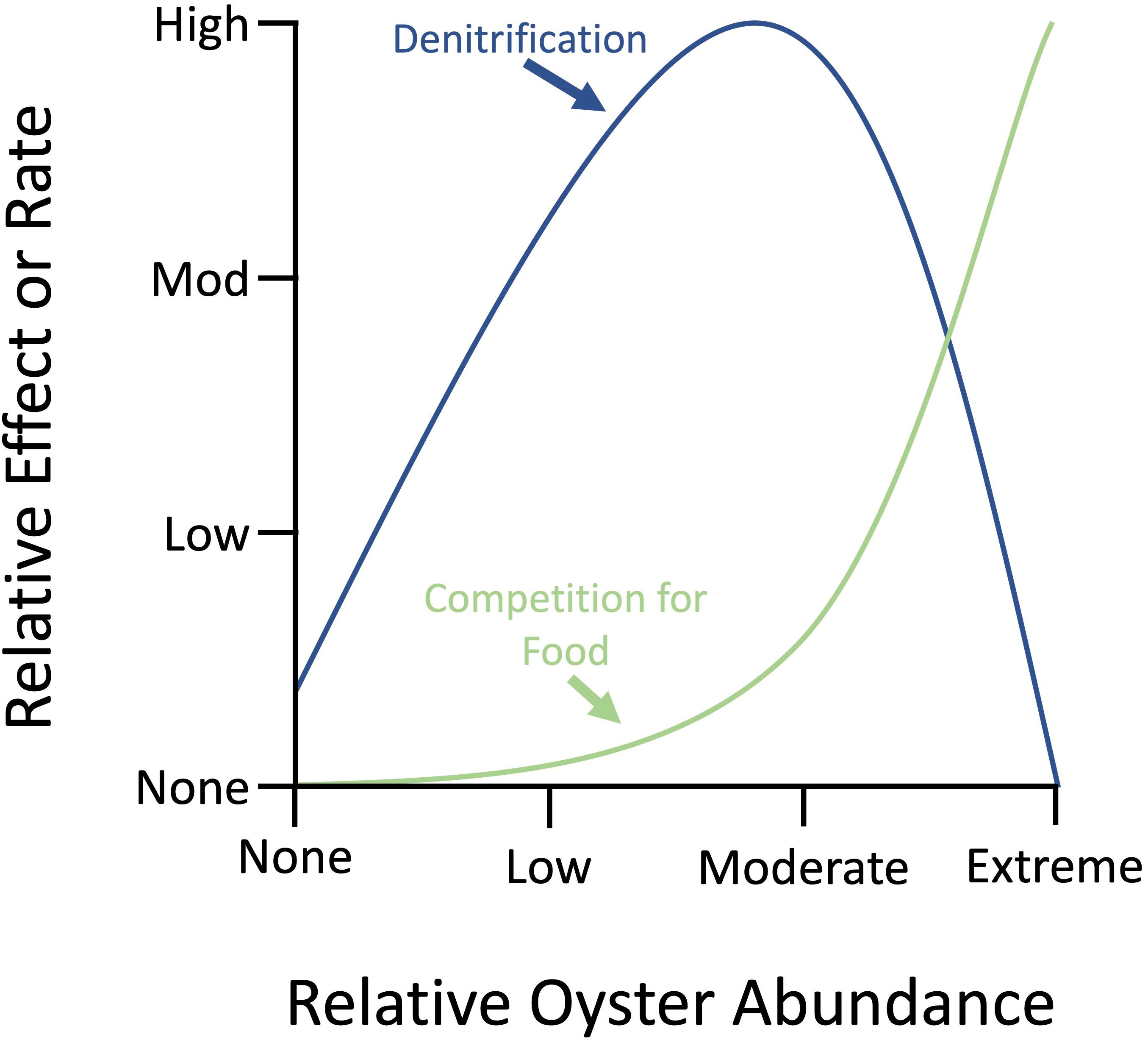
Credit: Modified from Newell (2004)
Summary
Nitrogen pollution and eutrophication have led to increasing interest from local governments, environmental organizations, researchers, and the public to consider increasing oysters (and other bivalve shellfish) in coastal systems as a way to improve water quality. Oysters and oyster reefs can help meet nitrogen reduction goals because they remove nitrogen directly through assimilation into biomass and indirectly through the burial of biodeposits and enhanced sediment denitrification. However, factors such as oyster density and size and local conditions such as temperature, salinity, and hydrology can affect the nitrogen removal efficiency of these organisms and habitats. Site-specific measurements are necessary to evaluate the effectiveness of oyster-mediated nitrogen removal as an in-water mitigation strategy. The effectiveness may change significantly across regions given local conditions. Removal will also change, given the type of oysters. For example, oyster aquaculture can remove nitrogen through bioextraction, while enhanced denitrification is more applicable to oyster reefs that may not be harvested. Although oysters can remove nitrogen, increasing oyster biomass through reef restoration or aquaculture is not a solution to nitrogen pollution but an additional tool to be used with other forms of nitrogen control, including critically important efforts to reduce the amount of nitrogen entering the waterways from land in the first place. Understanding the processes that affect nitrogen removal rates and how results vary by water body is increasingly important as nitrogen pollution and eutrophication continue and as increasing numbers of stakeholders are looking to bivalve shellfish restoration and aquaculture as a potential tool to restore water quality.
Glossary
Assimilation: Nitrogen incorporated into the shell and tissue of an oyster.
Biodeposits: The collection of feces (digested) and pseudofeces (ingested but expelled) containing nitrogen and other nutrients and waste products.
Bioextraction: Removal of nitrogen in the shell and tissue when oysters are harvested, usually in aquaculture.
Carrying capacity: The number of organisms, such as oysters, that an environment can support without negatively impacting the environment and organism.
Dead zones: Areas low in oxygen where organisms cannot survive.
Decomposition: The process of decay that breaks down organic matter (i.e., biodeposits) into ammonium (NH4+) and available carbon.
Denitrification: The microbially mediated process of reducing harmful nitrogen from nitrate (NO3-) to harmless dinitrogen gas (N2).
Eutrophication: The increase of nutrients in a waterbody that results in algal growth.
Nitrification: The microbially mediated process of oxidizing NH4+ to NO3-.
References
Bricker, S. B., R. E. Grizzle, P. Trowbridge, J. M. Rose, J. G. Ferreira, K. Wellman, C. Zhu, E. Galimany, G. H. Wikfors, C. Saurel, R. L. Miller, J. Wands, R. Rheault, J. Steinberg, A. P. Jacob, E. D. Davenport, S. Ayvazian, M. Chintala, and M. A. Tedesco. 2019. “Bioextractive Removal of Nitrogen by Oysters in Great Bay Piscataqua River Estuary, New Hampshire, USA.” Estuaries and Coasts: Journal of the Estuarine Research Federation 43:23–38. https://doi.org/10.1007/s12237-019-00661-8
Carmichael, R. H., W. Walton, and H. Clark. 2012. “Bivalve-Enhanced Nitrogen Removal from Coastal Estuaries.” Canadian Journal of Fisheries and Aquatic Sciences 69 (7): 1131–1149. https://doi.org/10.1139/f2012-057
Cornwell, J., J. Rose, M. L. Kellogg, M. L. Luckenbach, S. B. Bricker, K. T. Paynter, C. M. Moore, M. Parker, L. P. Sanford, B. Wolinski, A. Lacatell, L. Fegly, and K. Hudson. 2016. Panel Recommendations on the Oyster BMP Nutrient and Suspended Sediment Reduction Effectiveness Determination Decision Framework and Nitrogen and Phosphorus Assimilation in Oyster Tissue Reduction Effectiveness for Oyster Aquaculture Practices.
Dame, R. F., J. D. Spurrier, and T. G. Wolaver. 1989. “Carbon, Nitrogen and Phosphorus Processing by an Oyster Reef.” Marine Ecology Progress Series 54:249–256. https://doi.org/10.3354/meps054249
Dame, R. F., R. G. Zingmark, and E. Haskin. 1984. “Oyster Reefs as Processors of Estuarine Materials.” Journal of Experimental Marine Biology and Ecology 83 (3): 239–247. https://doi.org/10.1016/S0022-0981(84)80003-9
Dvarskas, A., S. B. Bricker, G. H. Wikfors, J. J. Bohorquez, M. S. Dixon, and J. M. Rose. 2020. “Quantification and Valuation of Nitrogen Removal Services Provided by Commercial Shellfish Aquaculture at the Subwatershed Scale.” Environmental Science & Technology 54 (24): 16156–16165. https://doi.org/10.1021/acs.est.0c03066
Ehrich, M. K., and L. A. Harris. 2015. "A Review of Existing Eastern Oyster Filtration Rate Models.” Ecological Modelling 297:201–212. https://doi.org/10.1016/j.ecolmodel.2014.11.023
Grabowski, J. H., R. D. Brumbaugh, R. F. Conrad, A. G. Keeler, J. J. Opaluch, C. H. Peterson, M. F. Piehler, S. P. Powers, and A. R. Smyth. 2012. “Economic Valuation of Ecosystem Services Provided by Oyster Reefs.” BioScience 62 (10): 900–909.
Gray, M. W., D. Pinton, A. Canestrelli, N. Dix, P. Marcum, D. Kimbro, and R. Grizzle. 2021. “Beyond Residence Time: Quantifying Factors That Drive the Spatially Explicit Filtration Services of an Abundant Native Oyster Population.” Estuaries and Coasts 45:1343–1360. https://doi.org/10.1007/s12237-021-01017-x
Kellogg, M. L., M. Brush, and J. Cornwell. 2018. An Updated Model for Estimating the TMDL-Related Benefits of Oyster Reef Restoration. https://www.conservationgateway.org/Documents/Harris_Creek_Model_and_Oyster_Reef_Restoration_Benefits.pdf
Kellogg, M. L., J. C. Cornwell, M. S. Owens, and K. T. Paynter. 2013. “Denitrification and Nutrient Assimilation on a Restored Oyster Reef.” Marine Ecology Progress Series 480:1–19. https://doi.org/10.3354/meps10331
Kellogg, M. L., A. R. Smyth, M. W. Luckenbach, R. H. Carmichael, B. L. Brown, J. C. Cornwell, M. F. Piehler, M. S. Owens, D. J. Dalrymple, and C. B. Higgins. 2014. “Use of Oysters to Mitigate Eutrophication in Coastal Waters.” Estuarine, Coastal and Shelf Science 151:156–168. https://doi.org/10.1016/j.ecss.2014.09.025
Kreeger, D. A., C. M. Gatenby, and P. W. Bergstrom. 2018. “Restoration Potential of Several Native Species of Bivalve Molluscs for Water Quality Improvement in Mid-Atlantic Watersheds.” Journal of Shellfish Research 37 (5): 1121–1157. https://doi.org/10.2983/035.037.0524
Lunstrum, A., K. McGlathery, and A. Smyth. 2018. “Oyster (Crassostrea virginica) Aquaculture Shifts Sediment Nitrogen Processes toward Mineralization over Denitrification.” Estuaries and Coasts 41:1130–1146. https://doi.org/10.1007/s12237-017-0327-x
Newell, R. 2004. “Ecosystem Influences of Natural and Cultivated Populations of Suspension-Feeding Bivalve Mollusks: A Review.” Journal of Shellfish Research 23:51–61.
Newell, R. I. E., J. C. Cornwell, and M. S. Owens. 2002. “Influence of Simulated Bivalve Biodeposition and Microphytobenthos on Sediment Nitrogen Dynamics: A Laboratory Study.” Limnology and Oceanography 47 (5): 1367–1379. https://doi.org/10.4319/lo.2002.47.5.1367
Piehler, M. F., and A. R. Smyth. 2011. “Habitat-Specific Distinctions in Estuarine Denitrification Affect Both Ecosystem Function and Services.” Ecosphere 2 (1): art12. https://doi.org/10.1890/ES10-00082.1
Ray, N. E., and R. W. Fulweiler. 2020. “Meta-analysis of Oyster Impacts on Coastal Biogeochemistry.” Nature Sustainability 4:261–269. https://doi.org/10.1038/s41893-020-00644-9
Ray, N. E., M. C. Henning, and R. W. Fulweiler. 2019. “Nitrogen and Phosphorus Cycling in the Digestive System and Shell Biofilm of the Eastern Oyster Crassostrea virginica.” Marine Ecology Progress Series 621:95–105. https://doi.org/10.3354/meps13007
Rose, J. M., J. S. Gosnell, S. Bricker, M. J. Brush, A. Colden, L. Harris, E. Karplus, A. Laferriere, N. H. Merrill, T. B. Murphy, J. Reitsma, J. Shockley, K. Stephenson, S. Theuerkauf, D. Ward, and R. W. Fulweiler. 2021. “Opportunities and Challenges for Including Oyster-Mediated Denitrification in Nitrogen Management Plans.” Estuaries and Coasts 44:2041–2055. https://doi.org/10.1007/s12237-021-00936-z
Smyth, A. R., N. R. Geraldi, and M. F. Piehler. 2013. “Oyster-Mediated Benthic-Pelagic Coupling Modifies Nitrogen Pools and Processes.” Marine Ecology Progress Series 493:23–30. https://doi.org/10.3354/meps10516
Smyth, A. R., N. R. Geraldi, S. P. Thompson, and M. F. Piehler. 2016. "Biological Activity Exceeds Biogenic Structure in Influencing Sediment Nitrogen Cycling in Experimental Oyster Reefs.” Marine Ecology Progress Series 560:173–183. https://doi.org/10.3354/meps11922
Smyth, A. R., A. E. Murphy, I. C. Anderson, and B. Song. 2018. “Differential Effects of Bivalves on Sediment Nitrogen Cycling in a Shallow Coastal Bay.” Estuaries and Coasts 41:1147–1163. https://doi.org/10.1007/s12237-017-0344-9
Smyth, A. R., S. P. Thompson, K. N. Siporin, W. S. Gardner, M. J. McCarthy, and M. F. Piehler. 2013. “Assessing Nitrogen Dynamics throughout the Estuarine Landscape.” Estuaries and Coasts 36:44–55. https://doi.org/10.1007/s12237-012-9554-3
Vitousek, P. M., J. D. Aber, R. W. Howarth, G. E. Likens, P. A. Matson, D. W. Schindler, W. H. Schlesinger, and D. G. Tilman. 1997. “Human Alteration of the Global Nitrogen Cycle: Sources and Consequences.” Ecological Applications 7 (3): 737–750. https://doi.org/10.1890/1051-0761(1997)007[0737:HAOTGN]2.0.CO;2
Wade, T., and T. Borisova. 2022. “Water Quality Credit Trading: General Principles.” EDIS 2022 (1). https://doi.org/10.32473/edis-fe824-2022