The state of Florida ranks first in the country for market value of fresh tomatoes. Tomato accounted for 33.1% of Florida's total vegetable crop value in 2008 (Olson et al. 2010; Olson and Santos 2010; Simonne 2009). Harvesting of fresh market tomatoes in Florida for the 2007-2008 season resulted in 41,832,000 cartons (25 lb/carton) from 31,500 harvested acres (32,400 planted acres). The crop value was $622,251,000 (Fla. Agri. Stat. Bulletin 2009).
Fertilizer is a major part of the crop production expenses for tomato, but it is critical for successful crop yields and high fruit quality. Recommended target nutrient rates are currently 200-150-225 lb/acre N, P2O5, K2O with phosphorus (P) and potassium (K) rates adjusted downward or eliminated if soils can supply some or all of these nutrients as determined by soil testing with the Mehlich-1 soil test extractant (Mylavarapu 2009; Olson et al. 2010).
This publication does not present new fertilization recommendations but rather updates the previously published research literature review by the same title and includes information on new research conducted in the last decade. Current tomato fertilization recommendations are based on published field research, and a compilation of the literature contained in this document will assist with making valid fertilizer recommendations that are commercially viable and that reduce the risk of environmental consequences in adjacent water bodies. As environmental regulation becomes more commonplace, the importance of fertilization recommendations based on published research increases.
This publication documents the previous written literature, some of which now appears only in updated electronic format. Abstracts of all publications will be placed on the website https://bmp.ifas.ufl.edu for future reference.
Fertilizer recommendations do not only contain the recommended rate of fertilizer but also include the management strategies for getting the most from the fertilizer investment while protecting the environment. These principles for fertilization of vegetables are summarized by Hochmuth and Hanlon (2010a). Rate is only a part of the modern fertilizer recommendation. Sound fertilizer recommendations also consider fertilizer materials, placement, and timing of application, among other aspects (Hochmuth and Hanlon 2010b).
More than 60 years worth of tomato fertilization research has been conducted in Florida. During this time, many changes have occurred in tomato production practices, including changes in cultivars and the introduction of new cultural systems, such as polyethylene mulch and drip irrigation. The research reported here covers tomato production with polyethylene mulch. Tomato crop and fertilizer management recommendations, such as plant and row spacing, have changed over time following new developments in research (Montelaro 1978; Hanlon and Hochmuth 1992; Hochmuth 1988a, b; 1996a; 1996b; Hochmuth and Hanlon 1989; 1995a; 1995b; 2000; Hochmuth and Smajstrla 1997; Hochmuth et al. 1994a; Kidder et al. 1989; Olson et al. 2010; Simonne 2009; Simonne and Hochmuth 2008; 2010). The most current recommendations for nutrient management in tomato production are presented in the Commercial Vegetable Production Handbook for Florida (Olson and Simonne 2010). UF/IFAS recommends a target of 200 lb/acre N, 150 lb per acre P2O5, and 225 lb per acre K2O only when soil concentrations of phosphorus (P) or potassium (K) are very low, based on the results of Mehlich-1 (M-1) soil testing (Hochmuth and Hanlon 1995a; 2000).These recommendations have been in effect for the last decade; however, the recommendations were revised through the years. The recommendation of 120-160-160 N-P2O5-K2O was in place since the 1970s (Montelaro 1978) but was revised in 1989 to 175-160-160, and that was later revised in 1997 to 200-150-225 (Hochmuth and Hanlon 1989; 1995b; Hochmuth and Cordasco 2000). Commercial growers often apply higher rates than recommended (Everett 1976; Rhoads et al. 1996) to reduce the risk of yield reductions when facing unfavorable production conditions.
The early research focused largely on yield and fruit quality (size and shape) in response to fertilization. Since the early research was conducted and the first literature review in 1999, there have been increasingly strong interests in incorporating more environmental impacts (i.e., water quality) into the fertilizer research. The state (Fla. Dept. of Agr. and Cons. Serv. 2005; Simonne and Hochmuth 2010) has been formalizing Best Management Practices (BMPs) and encouraging growers to implement BMPs. Part of the definition of a BMP includes both economics and environmental components based upon the best available science.
This literature review includes all available published documentation concerning tomato yield and fertilizer use in Florida. We chose to present all the research without being selective as to years and types of studies to avoid bias in the presentation. Inclusion of all Florida literature also shows the development of the commercial production system with respect to fertilizer use. Commercial yields have been increasing primarily because of the proper selection of new cultivars with traits that resist disease and pest pressures. Since the basic plant has not changed, nutrient requirements have been slow to change. In fact, nutrient use efficiency (due to new production systems) has increased during the last few decades while commercial fertilizer rates have not.
Since nutrient and water management are linked, fertilization research is summarized by irrigation method. Reviews such as this publication may be used in the process of determining or revising recommendations. The selection of a fertilization recommendation addresses commercial yield and quality, the economics of crop production, and protection of the environment. Equally as important is to have a mechanism available to the grower to adjust production practices because of leaching rains and extension of the growing season for additional harvests when economic conditions are favorable. To address all of these concerns, UF/IFAS vegetable recommendations are given as a single target fertilizer rate and a series of footnotes that explain the addition of fertilizer during the growing season and address leaching rains and additional harvests. This single target fertilizer rate recommendation is better than a range because most individuals would naturally select the highest value in the range. Therefore, it is logical to select a single target rate that avoids unneeded fertilizer applications that often reduce crop nutrient efficiency and increase the potential for environmental degradation.
This publication is an updated version of a previous research literature review by Hochmuth and Cordasco (2000, reviewed 2008) that covered fertilizer research through 1996. This new literature review adds the research reports conducted since 1996 and expands on the role of irrigation in managing fertilizer for crop production efficiency and environmental protection. The audience for this publication includes Extension specialists and agents, commercial vegetable producers, consultants, and governmental agencies.
Data Summary Method
Evaluation of tomato yield responses to varying rates of applied fertilizer required a standardized method of summarizing statewide yields, which were expressed in research reports variably as kg/ha, lb/acre, Mg/ha, and cartons or boxes per acre (25 lb box). In addition, vegetable yields vary depending on season, cultivar, and location in the state. Relative yield (RY), a calculated percentage, was chosen as the unit to express tomato yield responses to fertilization. Relative yield is an accepted scientific method for summarizing and presenting data across wide sources and reports (Brown 1987).
In his book, C. Black (1992) summarizes the advantages and disadvantages of the RY approach. There are valid statistical concerns about RY, but he concludes the RY approach can be useful in displaying general relationships when it is applied properly and cautiously. Black demonstrates examples in his book where RY yield is helpful and where it is not. We chose the RY approach because we wanted to display the historical data without making biased decisions about what to include in the presentation. Plotting absolute yield data in original units obviously would result in a scatter of data that renders any general interpretation impossible. Black (1992) points out that decisions can always be improved with further research, but the data on hand are the best we have at the time.The highest yield for each fertilizer experiment was assigned a 100% value, and other yields were expressed as a percentage of the highest yield. The actual yield corresponding to 100% RY was presented in the number of 25 lb boxes per acre. The RYs were plotted against rates of nutrient to determine how tomato yields responded to fertilizer in Florida. The RY presentation allowed data from a variety of experiments to be included in the graphical summary of yield responses. For most studies, RYs of 90%–100% were not significantly different.
Sometimes the argument is made that growers have expectations of greater yields than those obtained in research projects. Realistic, regularly obtainable yields are a more reliable measure than expected yields or "yield goals." Research on this subject has documented that 20% of growers actually reached their yield goals, and only 50% reached 80% of their yield goals (Schepers et al. 1986). Therefore, growers rarely achieve their stated yield goals; this means that fertilizer rates should not be set on perceived or desired yield goals, but rather on realistic goals based on research. This practice of using yield goals to set fertilizer rates is not recommended in Florida, so the effect of over-fertilization has been avoided by grounding expectations in measurement of observed yields. In effect, the current Florida fertilizer recommendation includes a component that addresses growers' concerns about yield expectation versus actual production capability. Scientists throughout the years have conducted many replicated demonstrations studies in growers' fields using true commercial production practices, and those studies are included in this review. In times past, excessive fertilizer was justified as inexpensive insurance against yield loss, and less attention was given to the environmental consequences. Research with numerous crops has shown that nutrient use efficiency declines as nutrient rate, especially N, increases. Even given the best of production systems, N use efficiency rarely exceeds 70% of the applied N. Concepts and practices for managing nutrients in vegetable production were summarized by Hochmuth (1992a, b; 2000). Further, it has been suggested that the yields in older studies were much lower than yields obtained today. However, there are older research reports that showed yields were as high as yields achieved today. While fertilizer rate is reported in pounds per acre (lb/acre), the reader should keep in mind that the bed system used in each field may vary and require adjustments to the fertilizer rate. To address this issue, the linear bed foot concept can be used to convert among the various bed designs with and without spray rows or ditches (Hanlon and Hochmuth 1989; Hochmuth and Hanlon 2009). Additional cultural practices for commercial tomato production can be found in Chapter 2 of the Vegetable Production Guide (Simonne and Hochmuth 2010).
Keeping nutrients in the soil profile and available for tomato plant uptake requires proper irrigation management. Information is regularly updated because of the critical nature of irrigation and drainage management with respect to nutrient uptake by the plant and possible loss of nutrients to the environment (Simonne et al. 2010).
Another tool for measuring nutrients that contributes to appropriate management of fertilizer includes plant tissue and/or plant sap sampling. For interpretation of plant and sap values, see Hochmuth (1994a, b) and Hochmuth et al. (1991b; 2009).
Growers may tend to over-irrigate their crops, which may also contribute to the loss of vital N needed for plant growth into the environment. Conventional sub-irrigation practice raises the already high water table to allow water movement to the planting beds. Stanley and Clark (1991; 2003) advocated the fully enclosed sub-irrigation system (FES) that uses subsurface drainage tubing to sub-irrigate crops. This system has the ability to reduce irrigation water use by 30%–40%. The FES system also has the potential to reduce N loss after periods of heavy rainfall when elevated water tables drop, taking with it dissolved N from the planting beds (Stanley and Clark 2003). The FES may apply to other crops grown with subsurface irrigation, such as pepper.
Nitrogen Mixed Fertilizer Trials
Responses of tomato to rates of mixed N-P-K fertilizers are presented in Figure 1. The data were graphed as a function of the N content of the fertilizers. Most of these studies were conducted with sub-irrigation (seepage irrigation). Fertilizer rate was a factor in two Immokalee experiments conducted in the fall of 1970 and spring of 1971 (Everett 1971). Increased use of polyethylene mulch on several thousand south Florida tomato production acres resulted in disposal problems estimated at $50–$70 per acre. Residual polyethylene in the field was caught up in tillage equipment and interfered with seeding and transplanting operations. These problems led to interest in biodegradable paper and polyethylene-coated paper mulches. Tan or black paper mulches (impregnated with a fungicide to retard decomposition) were uncoated or coated with 0.25 mil of polyethylene film on one or both sides. Single-side coated mulches were applied either coated side up toward the sun or down toward the soil. Yields from tomatoes mulched with these biodegradable mulching materials were compared to yields from plants mulched with 1.5 mil of black polyethylene. Yields were also compared for plants fertilized with N-P2O5-K2O fertilizer formulations of 130-72-157 (low), 220-72-261 (medium), or 310-72-364 (high). All plots received 40 lb/acre N from a 5-8-8, N-P2O5-K2O fertilizer placed 3 inches to each side of the bed center and 3 inches deep. A second application of an 18-0-25, N-P2O5-K2O fertilizer was applied 9 inches to each side of bed center at rates of 500, 1000, or 1500 lb/acre. This fertilizer side-dress application was divided into two side-dress applications for the unmulched beds. Unstaked, subsurface-irrigated plants were grown for one to three harvests. Row or water-furrow spacing was not indicated.
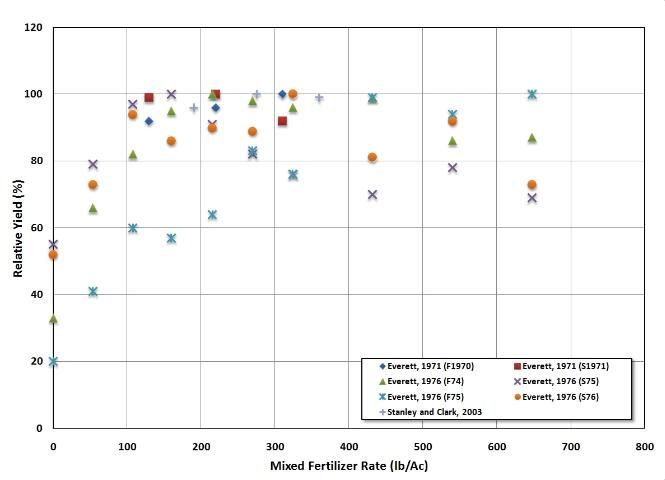
Mulch type and fertilizer rate did not interact significantly for either experiment. Yields did not respond to increased fertilization in these experiments where the author noted a limited, 1 to 3 pick. Harvest season reduced the demand for fertilizer. Yields averaged 1,227 cartons/acre across fertilizer and mulch treatments in fall 1970. In the spring 1971 season, yields were maximized with application of 130–220 lb/acre N, while yields fell slightly with application between the medium and high fertilizer treatments (220–310 lb/acre N) from 1,664 to 1,536 cartons/acre (significant at 5% probability). In the fall 1970, yields were best from plants mulched with uncoated tan paper (1,464 cartons/acre) or black paper (1,376 cartons/acre). Yields from plants with all other mulch treatments were not significantly greater than yields from unmulched plants (1,184 cartons/acre). Drought conditions and low temperatures in spring 1971 accentuated the benefits of mulch. Yields were significantly better (1% probability) from all mulched plants, regardless of mulch type, compared to unmulched plants. Mulched plants produced 400–856 cartons/acre more tomatoes than the unmulched plants; higher yields resulted from plants in black mulches than from those in tan mulches. The highest yields in this dry season resulted from tomato plants mulched with black paper (polyethylene side to the soil), 1,920 cartons/acre compared to yields from unmulched plants, 1,064 cartons/acre. Similar yields resulted where polyethylene-coated papers were placed coated side up or coated side down. Paper mulches performed well with these unstaked tomatoes largely because of the abbreviated season (3.5 months) and reduced foot and machine traffic compared with the tomatoes grown with the staked cultural system.
The effects of increased rates of mixed N and K fertilizer on yield and fruit size of polyethylene mulched, stake-grown tomatoes were evaluated through two spring and fall seasons in Immokalee (AREC) (Everett 1976). Beds spaced 6-feet on center received 0, 54, 108, 162, 216, 270, 324, 432, 540, or 648 lb/acre N and 0, 75, 150, 225, 300, 375, 450, 600, 750, or 900 lb/acre K2O from NH4NO3 and KNO3 (70% NO3-N and 30% NH4-N). An 18-inch fertilizer band was applied from 10% of N for treatments through 324 lb/acre and 5% of N for treatments from 432 to 648 lb/acre. The remaining fertilizer was applied in two surface bands 9 inches to each side of the plant row. Subsurface irrigation was applied in all experiments to Immokalee fine sand soils. Common N and K fertilizer rates used by area growers at the time were cited as 350 lb/acre N and 475 lb/acre K2O.
Marketable yield increases were observed only between 0–54 lb/acre N in fall 1974 (571–1130 cartons/acre, respectively). In spring 1975, yields were similar with or without N fertilizer, averaging 1,058 cartons/acre. Heavy rains this season damaged roots and limited the crop to two harvests. Significant yield increases occurred in fall 1975 with N rates through 648 lb/acre N (2,800 cartons/acre, 100% RY) from 64% RY with 216 lb/acre N. The spring 1976 experiment yields with 0 lb/acre N were nearly twice those of previous seasons with 0 lb/acre N. The author cited upward movement of nutrients with subsurface irrigation and the presence of a spodic soil horizon known to impede nutrient leaching. These same factors likely increased soluble salt content that resulted in reduced yields with higher N rates in spring 1976 (73% RY with 648 lb/acre N compared to 100% RY, 2,734 cartons/acre, with 324 lb/acre N). Nitrogen fertilization did not affect fruit weight in any season, except with the initial N fertilization of 54 lb/acre N in fall 1975. The author concluded that tomatoes grown on previously cropped land reached optimum yield in the range of 162–270 lb/acre N.
Two spring tomato experiments were conducted on beds previously mulched for a fall tomato crop to test fertilizer rates and placement methods in a two-crop system (Everett 1978). Tomatoes grown in both the fall (first planting) and spring (second planting) were unstaked, single-harvest, "ground" tomatoes. Yields from the second planting were 50%–60% lower than yields from the first planting, but estimated production costs were reduced 70%–75% by reusing the mulched beds. Fertilizer was placed in a hole punched through the polyethylene at rates of 0, 0.5, 1.0, 2.0, or 4.0 oz per hole for the second crop. Fertilizer holes were 8 inches to one side of the plant, 8 inches to both sides of the plant, or halfway between the plants (in the "drill"). Nitrogen treatments of 0, 65, 130, or 260 lb/acre (18-0-25 N-P2O5-K2O fertilizer) were calculated based on 5,808 plants/acre (5-foot row spacing). Fields were subsurface irrigated to maintain soil moisture at field capacity before planting the spring tomato crop (3 weeks after removing fall season plants).
Yields increased in both spring seasons with N treatments between 0–65 lb/acre to 90%–94% RY each season. Yield increases with N treatments greater than 65 lb/acre were not significant. High or 100% RYs, occurred with 260 lb/acre N (714 cartons/acre) and with 130 lb/acre N (781 cartons/acre) in each respective spring season. Fruit size increased with 130 and 260 lb/acre N in the first season and increased only with 65 lb/acre N in the second season. Fertilizer placement did not affect yield or fruit size in either experiment season.
Nitrogen
Overhead Irrigation
In areas of the state where sub-irrigation is not possible, overhead (sprinkler) or drip irrigation systems are used. Although fertilizer leaching losses were reduced with polyethylene mulching on overhead-irrigated fields, researchers found that soluble salt injury reduced yields when all of the fertilizer was applied before mulching (Locascio et al. 1984). In a 1980 spring trial on Sparr sand, researchers sought to avoid this early plant fertilizer injury yet apply sufficient N for the late-season demand of fruit set and development. Four preplant N sources, including KNO3-Ca (NO3)2, NH4NO3, sulfur-coated urea (SCU), or isobutylene diurea (IBDU), were applied preplant-broadcast and incorporated in single N-source treatments or paired with another source for sixteen treatments, each applied at 200 lb/acre N. Prepared beds were spaced four feet apart.
Yield was greatest with IBDU combined with KNO3-Ca (NO3)2 in a 2 to 1 ratio (3,177 cartons/acre). Similar yields resulted with all combinations of IBDU or SCU and KNO3-Ca (NO3)2. Intermediate yields resulted with controlled-release nitrogen (CRN) sources (IBDU or SCU applied singly) and lowest yields resulted with soluble N sources (NH4NO3 or KNO3-Ca(NO3)2) applied singly. Intermediate to low yields resulted with all combinations of NH4NO3 with other N sources, likely due to soluble salt effects of NH4NO3 on early plant growth. Researchers concluded that the N release rate of IBDU was superior to SCU based on optimum tomato yields and that yields with CRN sources were highest only when combined with soluble N sources.
In spring 1981, tomatoes grown on Plummer sand were evaluated to see how mulch, N source, and irrigation method (overhead or drip) affected tomato yields (Sweeney et al. 1987). Nitrogen sources were a 50:50 mix of NH4NO3 and SCU or 100% NH4NO3. Mulched and sprinkler-irrigated tomatoes received 100% of the N and K preplant. Unmulched and sprinkler-irrigated tomatoes received 50% of the N and K preplant/incorporated and 50% side-dressed in two equal applications. Drip-irrigated tomatoes received 50% of N and K preplant and 50% through the drip line. Plant N recovery was measured by a 15N-labeled NH4NO3, which was applied 100% preplant or in the split-N treatment as either the 50% preplant-applied N or the 50% fertigated N. Rates of N, P2O5, and K2O were 200-230-220 lb/acre, respectively, for beds spaced 4 feet on center.
Irrigation method did not affect the marketable fruit yields of tomato. However, the use of polyethylene mulch increased marketable fruit yields 25% (2,445 cartons/acre) compared to unmulched plants (1,842 cartons/acre). Likewise, mulch use enhanced N recovery by vegetative plant tissues, which extracted 30% of the applied preplant N compared to 12%–14% N recovery with the unmulched plants averaged across irrigation methods. Nitrogen recovery in the fruit was not affected by mulch or irrigation method. Researchers found that less fertilizer N was needed when mineralized N from soil organic matter was accounted for. Plant, fruit, and vegetative tissues recovered 30–60 lb/acre of soil N and an additional 110–150 lb/acre N from the 200 lb/acre of applied N. In this study, marketable yields were affected by N source. Twenty-one percent more fruits were harvested from plants fertilized with NH4NO3 (2,400 cartons/acre) than plants fertilized with 50:50, NH4NO3: SCU (1,884 cartons/acre).
Unaccounted-for N amounted to 10 lb/acre N from mulched and overhead-irrigated beds. Researchers found that much more N was unaccounted for and presumed leached from drip-irrigated mulched or unmulched beds and from overhead-irrigated unmulched beds averaging 93 lb/acre of unaccounted-for N. Leaching losses from drip-irrigated beds occurred despite seasonal water use of 7 inches compared to 16 inches of applied water with overhead irrigation. All tomatoes received 21 inches of rainfall, eight of which were moderate rainfall events (1–2 inches each).
Subsurface Irrigation
Tomato responses to rates of N with seepage (subsurface or sub-) irrigation are presented in Figure 2. Two tomato cultivars, a breeding line '7060' and 'Horizon', were used in studies (fall 1983 and spring 1984) involving N and K rates (Csizinszky and Scott 1985). Rates were 1, 1.5, and 2.0 X where the 1X was 225 lb/acre N and 187 lb/acre K2O. The cultivar '7060' did not respond to N rates in the fall. In the spring season, increasing N rate reduced marketable fruit yield and increased cull production of both varieties.
Starter fertilizer was applied at 20 lb/acre N in Manatee County in fall 1988, 30 lb/acre N in Palm Beach County in winter 1988-1989, and 40 lb/acre N in Manatee County in spring 1989 (Hochmuth et al. 1989). The starter fertilizer was supplemented with banded NH4NO3, KNO3, and Ca(NO3)2. Nitrogen rates calculated on the basis of a 6-foot bed center for the Manatee studies were applied in bands, and the beds were mulched with polyethylene. Water table height was continually measured by a water stage recorder.
Fall 1988 yields at Manatee County for a three-harvest season were lower than yields at the winter and spring sites. Yields were not significantly different with total (starter plus band-placed fertilizer) N rates from 160 to 280 lb/acre. A 98% RY (752 cartons/acre, based on 13-foot row spacing) was achieved with 160 lb/acre N. Large fruit made up only 16% of the total marketable yield in this fall season of high rainfall. The yield with the grower fertilizer rate of 366 lb/acre N was 15% lower than with the reduced N rates (160–280 lb/acre N). Leaf N concentrations were all high at first flower sampling and remained high through early harvest, increasing linearly as N rate increased.
In a winter 1988-1989 planting in Palm Beach County, fertilizer rates and yields were based on beds spaced on 5.5-foot centers. Increasing N rates from 160 to 280 lb/acre had no effect on yield. High yield occurred with 160 lb/acre N, 100% RY (1,153 cartons/acre). Leaf N concentrations at all sampling dates were high and not different with increasing N rates. Large fruit accounted for 20% of the total marketable yield.
Spring 1989 yields at Manatee County, which was a two-harvest season, were based on 3,350 LBF (13-foot row spacing). A 99% RY (1,308 cartons/acre) occurred with 180 lb/acre N and was not significantly improved with N rates of 240 and 300 lb/acre. Yields with the grower N rate of 402 lb/acre did not exceed yields with 180 lb/acre N.
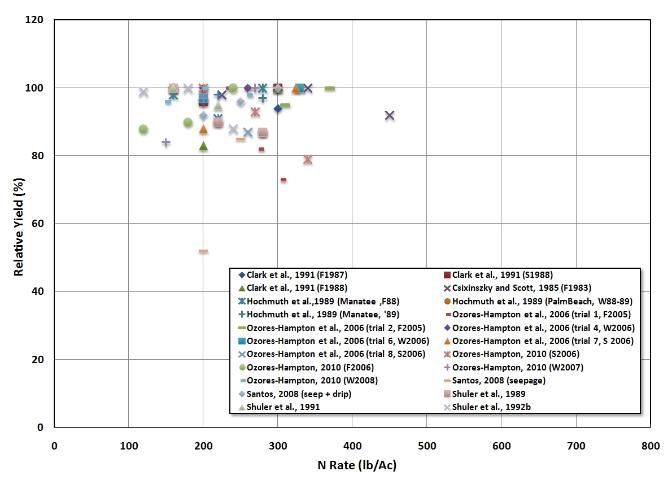
Leaf N concentrations were adequate and not different with increasing N rates. Large fruit comprised 61% of the total marketable yield. This season was marked by early drought that dropped the water table to 36 inches below the bed surface on four occasions.
Fertilizer containing 30-110-60 lb/acre N, P2O5, K2O was broadcast preplant on Oldsmar sand (0.62% organic matter) at Boynton Beach in the fall 1988 (Shuler et al. 1989). Additional fertilizers from KNO3, Ca(NO3)2, and NH4NO3 were applied in double bands resulting in total N rates of 160, 220, or 280 lb/acre. These rates were tested against the grower program of 336 lb/acre N and 672 lb/acre K2O. Bed centers were 5.25 feet apart, mulched with black polyethylene, and planted with 'Sunny' tomatoes. Water levels were monitored using a water table recorder.
The greatest yield response, 100% RY (1,922 cartons/acre), occurred with 160 lb/acre N. Yields dropped to 90% RY with 220 lb/acre N, to 87% RY with 280 lb/acre N, and to 91% RY with the grower fertilization program. Leaf N concentrations were high and not different through all growth stages with all N rates.
An on-farm research/demonstration study was conducted in southwest Florida on a farm using seepage irrigation (Clark et al. 1989). The trial was conducted for three seasons, fall 1987, spring 1988, and fall 1988. This work was one of the early studies conducted on a farm to help growers evaluate drip irrigation as an alternative to seepage irrigation. This trial used three levels of irrigation management (drip-irrigation with -10 and -15 centibars on a tensiometer and seepage with water table management). Two levels of fertilizer were used, 200 and 300 lb/acre nitrogen. Drip irrigation provided for similar yields with seepage irrigation but with less water used. Drip-applied N at 200 lb/acre resulted in similar or better yields compared with 300 lb/acre N with the seepage system. Reducing fertilizer from the high grower rates in combination with more precise irrigation application made possible with drip irrigation maintained current yields while potentially reducing N leaching (Clark et al. 1991).
Low organic matter (0.6%) Myakka sand fields in Boca Raton were planted in fall 1990 with 'Sunny' tomatoes (Shuler et al. 1991). Nitrogen rates of 160 and 220 lb/acre were compared to the grower rate of 328 lb/acre N (502 lb/acre K2O). Beds were spaced 5 feet apart and fertilizer rates and yields were expressed on 5-foot bed spacing. Preplant fertilizer, 48-160-40 lb/acre N, P2O5, K2O, was broadcast and covered with black polyethylene mulch. The remaining N was applied in double bands 20 inches apart. Nitrogen sources were KNO3, Ca(NO3)2, and NH4NO3.
Yield response was greatest with 160 lb/acre N providing 100% RY (1,597 cartons/acre). Relative yield dropped 5% with 220 lb/acre N. The grower yield was 92% of maximum yield. Leaf N concentrations through early fruit set ranged from 4.6% to 5.7%, higher than the adequate range of 2.5% to 4.0% (Hochmuth et al. 1991b). Nitrogen concentrations in leaves were not different through early fruit set with all N rates including the higher grower N rate. At harvest, leaf N concentrations fell to adequate levels with all N rates. Large fruit accounted for 20% of total marketable yield. Incidence of graywall increased from 150 to 195 cartons/acre with N rates between 160 and 220 lb/acre (significant at 5% probability).
Three N rates of 120, 180, and 240 lb/acre were tested at Boynton Beach during fall-winter 1991-1992 (Shuler et al. 1992). Beds were spaced 5 feet apart, and fertilizer rates and yields were expressed on 5-foot bed spacing. Broadcast fertilizer containing 40-200-40 lb/acre N, P2O5, K2O was applied in a 12-inch center band before bed shaping. White-on-black polyethylene mulch was pulled back for application of additional double-band fertilizer treatments of 80, 140, or 200 lb/acre N for total N rates of 120, 180, or 240 lb/acre.
Added N at rates higher than 120 lb/acre had no significant effect on yield or fruit quality of 'Sunny' tomato. Plants across all N rates yielded 29%–31% number-one grade large fruit. Equivalent yields were produced with 120 and 180 lb/acre N, 1,638 and 1,642 (100% RY) cartons/acre, respectively. Leaf N concentrations were exceeded at first flower with the adequate concentration of 4.0% and at early fruit set with concentrations greater than 6.0% and 5.0% measured at each stage. Graywall affected 10% or less of the fruit and did not increase with N rate.
Tomatoes in four of the subsurface-irrigated trials summarized above reached maximum yields with 160 lb/acre N, while in two other trials, yields were maximized with 120 and 180 lb/acre N. In all trials, yields were maximized with N approximating the 1995 UF/IFAS recommended 175 lb/acre N for tomato (Hochmuth and Hanlon 1995a), and less than the 1997 current recommendation of 200 lb/acre N. Increasing N to more than 175 lb/acre rarely increased yields or fruit quality. Leaf tissue sampling showed that tomato generally absorbed N at higher than the adequate level. Increased N sometimes increased incidence of graywall in 'Sunny' tomatoes.
In southwest Florida (Collier, Hillsborough, and Manatee Counties), a fertilizer trial was conducted to determine whether the current UF/IFAS recommended N rate of 200 lb/acre (with possible supplemental additions) was still best for good tomato yields and economic viability. Ozores-Hampton et al. (2005; 2006a) conducted 10 trials on grower farms with seepage-irrigated crops (6 trials) and drip-irrigated crops (4 trials). There were different plant varieties used (mostly 'Florida 47' and 'Sebring'), and plot size varied by grower from 0.1 to 50 acres. Comparisons were made between the yields generated from the UF/IFAS recommended rate (200 lb/acre N) and the rates used by the growers. Rates ranged from 200 to 418 lb/acre N. Tomatoes were harvested from at least 6 small plots in each treatment area and graded by standard USDA specifications. There were no true replications in any of the tests, so statistical comparisons of treatment means were not possible. The authors described how they compared within plot variability and between plot variability. The categories for fruit size were extra-large (5 x 6), large (6 x 6), and medium (6 x 7).
There was no significant difference in biomass of the plants grown with the lower N rate (UF/IFAS rate) and the grower rates at 30 and 60 days after transplanting. Tomato sap NO3-N was significantly higher for plants grown with the grower rates. Without replication, it is impossible to determine treatment differences. Despite this fact, the authors made assumptions about the economic differences in terms of crop value by ascribing a value to the various grade categories of fruit sizes. There were no yield results presented, and there were no measurements of environmental impacts among the various N treatments.
In the 2005-2006 growing season, Ozores-Hampton et al. (2006b) continued a fertilizer study to measure the effect of N rates on fruit yield. The study involved 8 farms in southwest Florida with N fertilizer rates from 200 lb/acre (the recommended rate) to grower rates up to 330 lb/acre (Ozores-Hampton et al. 2006b). For the seepage-irrigated trials, N treatments were arranged in a completely random design with three replications. For the drip-irrigated fields, there were two irrigation zones, one each for the N treatment. There was no replication, but twelve sampling plots were identified in each zone. N treatments for the seepage trials were formulated with custom-made fertilizers that kept P and K rates constant across the N treatments. Additional N was applied to the drip-irrigated fields as a supplement (recommended by UF/IFAS guidelines) following Hurricane Wilma.
As was the case in the 2004-2005 study, there was no significant difference in biomass of the plants grown with the lower rate (UF/IFAS rate) and the grower rates at 30 and 60 days after transplanting, except for one field where bigger plants resulted with the grower N rate. There were no differences between N treatments for any harvest or total-season fruit yields in the fall crops, except for one site where the production of extra-large fruits was greater with the recommended rate. In the winter, there were no differences for first harvest at two sites but greater yields of extra-large and total fruits with the recommended rate at a third site. There were no treatment effects on the second-harvest yields in the winter. Yields of third pick large, medium, and total fruits in the winter season were greater with the grower N rates at two sites, but there were no treatment effects at the third site.
Two sites were used in the spring of 2006. For first pick, yields of large and total fruit were greater with the grower N rate at one site, but there were no treatment effects at the second farm. Total yields of second harvest fruits across all size categories were not affected by N rate at either farm. Yields of large fruits in the second harvest were greater with the grower rate at one location but not the other farm. Extra large fruit yields were greater with the recommended rate at one location. For the third pick, fruit yields were greater with the grower N rate at one farm, but there were no treatment effects at the second farm.
Total-season fruit yields (sum of three picks for all fruit size categories) were only rarely affected by N treatment. There were no treatment effects at either farm for the fall crops. In the winter, there were no differences between N rates at 2 of 3 farms. At the third farm, seasonal production of extra-large and medium fruits was greater with the grower N rate, but total seasonal fruit production was not affected by N rate. In the spring at one farm, the grower N program led to greater yields of large, medium, and total season fruit production. At the second farm, the recommended N rate resulted in more extra-large fruit, but the other size categories and total yields were not affected by N treatment.
In these on-farm replicated trials, the authors rarely measured a significant difference between the grower and recommended N rates for effects on fruit yield in the three seasonal picks for fruit size categories or for total seasonal yields. Treatment effects across fruit categories, harvest, or total-season yield can be counted. There were 8 farm/season trials, three picks plus total seasonal data, and three size categories plus a total yield across sizes for each pick. Therefore, there were 128 instances where observations can be made about treatment comparisons. There were 6 instances in favor of the recommended rate and 18 instances where the grower rate was favored. There were 104 instances where no treatment effects were measured. Most of the instances when the grower program was favored occurred with the latest harvest in the season and the smaller fruit sizes (typically the least value). The recommended rate was favored most often with the largest fruit size category (typically the most value). Yields ranged from 1,600 25-lb cartons/acre in the fall to over 3,000 cartons/acre in the spring.
Ozores-Hampton et al. (2006a) instituted replicated trials in the 2005-2006 seasons. It is often impossible to predict when in a particular season a particular fruit size category will be most desired. Therefore, it is difficult to place an economic value on the crop. Even though there were few instances of significant treatment effects, the authors calculated yield thresholds that would pay for the extra costs of fertilizer with the grower fertilizer program. These thresholds can be calculated mathematically but are meaningless where there are no significant treatment effects on yield. The thresholds should not be used unless it is clear there are true treatment differences for yield.
The multi-farm trial conducted by Ozores-Hampton et al. (2006a, b) did not measure the environmental impacts of the various N treatments. Most of their data showed the recommended N rate was sufficient for highest yields of tomatoes with seepage irrigation or with drip-irrigated tomatoes in any season. There were a few instances where yields were favored with the higher N rates. These instances should be investigated further to determine why these farms needed more N. Other production practices, such as irrigation management, may need to be adjusted so that a farm is able to benefit from the recommended N rate.
N rates from 20 to 420 lb/acre were used in an experiment testing the impact of non-fertilizer N sources of reclaimed water and mineralized soil organic matter (Ozores-Hampton et al. 2007). The authors estimated that 56 lb/acre N was supplied from the reclaimed water plus N released from the soil organic matter at 2.8%. Petiole fresh sap nitrate concentrations were similar and sufficient with N rates from 116 to 476 lb/acre N. Yield of early extra-large fruits (70% of total) responded negatively to increasing N rate. Other yield parameters, including total yield and fruit quality (pulp pH, titratable acidity, and soluble solids content), did not respond to N rate.
The data in Figure 2 are grouped around the intermediate N rates because most of these data came from on-farm demonstration studies where only two rates of N were used. The objectives in most of the studies were to compare yield responses from tomatoes with the grower N rate and tomatoes with the UF/IFAS recommended rate. There were no N rate treatment effects for most of the studies in Figure 2.
Fall 2006 and spring 2007 trials were conducted at the Gulf Coast Research and Education Center with combination seepage/drip-irrigated tomato (Santos and Scott 2008) to determine appropriate N rate and plant spacing for 'Fla. 8153' tomato. N rates resulted from 50 lb/acre N applied in the bed with all three N rate treatments, plus drip-applied N of 154, 189, and 224 lb/acre N. Total-season N rates were 204, 239, and 274 lb/acre N. There was no season by N rate or plant spacing interaction. Yields were 2440, 2416, and 2608 25-lb cartons/acre with the 204, 239, and 274 lb/acre N rates, respectively. Yield was significantly greater with 274 lb/acre N than with the two lower rates. Yields were greater with 18-inch plant spacing than with 24 inches.
With sub-irrigation, tomato responded to N rates in all research trials going back to the early 1970s. Innovations in irrigation efficiency resulted with the fully-enclosed sub-irrigation (FES) system leading to reduced water needs. The same FES led to increases in N efficiency as water applications were reduced. Most studies involving N rates showed no differences between the recommended N rate of 160 or 175 lb/acre in early studies in the 1980s and 1990s. Many of these reports were from replicated on-farm studies. More recent replicated and non-replicated demonstration studies documented rare instances when tomato yield responded positively to N rates exceeding the current recommended N rate of 200 lb/acre. When yield increases resulted, it was most often with the late-season third pick and with the smallest size tomato category.
Drip Irrigation
Drip irrigation has become a standard irrigation management technology for much of the tomato production in Florida. Since the 1980s, several studies have been conducted that resulted in the development of recommendations for nutrient management with drip irrigation (fertigation) for plastic-mulched tomato (Hochmuth 1992a; Hochmuth and Smajstrla 1997). These recommendations from Florida also have become guidelines for tomato production in the country with modifications for local situations (Hartz and Hochmuth 1996; Hochmuth and Hartz 1996). Florida has led the nation in conducting fertilization research with drip irrigation (Locascio et al. 1992). Tomato responses to N rates with drip irrigation are presented in Figure 3.
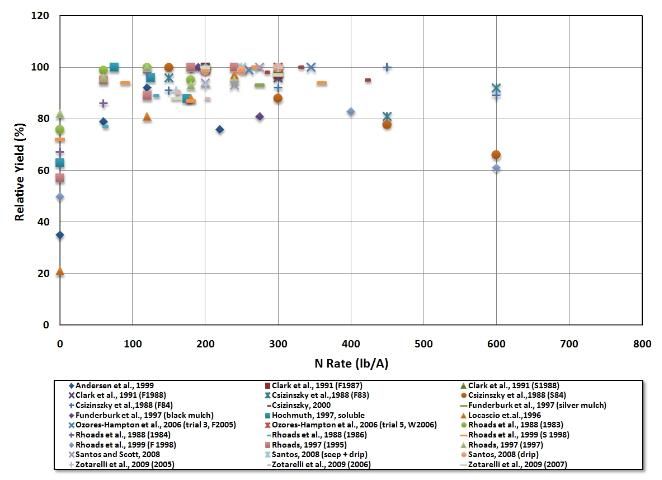
Research with Drip Irrigation in Central and Southern Florida on Spodosols
At the Gulf Coast Research and Education Center in Bradenton, yield responses to four N rates were tested during three growing seasons — fall 1983, spring 1984, and fall 1984 (Csizinszky et al. 1988). Nitrogen derived from NH4-N (30%) and NO3-N (70%) was applied, together with 30% preplant-incorporated K and 70% injected K throughout the fourteen-week growing season. Black polyethylene mulch was used in the spring season on beds spaced 4.5 feet on center, while white polyethylene was used in the fall season on beds with the same measurements. The fields were irrigated three times daily and received 68% of total water requirements in the early afternoon and 16% each in the early morning and late afternoon. Nutrient injection increased linearly through the fourteen-week season with heaviest fertilization in the last five weeks.
Marketable yields did not differ among N rates of 150, 300, 450, and 600 lb/acre in two fall seasons, 1983-1984. One hundred percent RY occurred with 300 and 450 lb/acre N (1,221 and 1,665 cartons/acre in 1983 and 1984, respectively). In the spring 1984 season, higher fertilizer rates reduced RY to 68% of the highest yield with 150 lb/acre N (1,050 cartons/acre) (Csizinszky et al. 1988). Leaf N concentrations measured at spring harvest in 1984 increased from 2.74% with 150 lb/acre N, which is an adequate level of added N, to 3.62% with 600 lb/acre N. Adequate leaf N concentrations for the harvest period were reported to be between 2.0% and 3.0% (Hochmuth et al. 1991b).
Research conducted during winter-spring 1998 (February-June) at the University of Florida Gulf Coast Research and Education Center in Bradenton measured the response of micro-irrigated 'Florida 47' to N rates and drip irrigation rates (Csizinszky and Scott 2000). The experimental design was a split-plot arranged in three randomized blocks. Irrigation was the main plot treatment with 0.7 and 1.0 times the pan evaporation. A third factor, calcium sprays, was included. Nitrogen application rates were 236, 282, 329, and 421 lb/acre N. N rate, irrigation rates, or calcium sprays did not have a significant effect on early tomato yields, total season yields, or fruit firmness or size. The lowest N rate employed in this study exceeded the recommended N rate of 200 lb/acre.
Two N rates and two tensiometer settings, -10 and -15 centibars, were evaluated on sandy, flatwood (Spodosols) soils during three seasons for effects on drip- and subsurface-irrigated tomato production at the Gulf Coast Research and Education Center in Bradenton (Clark et al. 1989). Total N rates of 200 or 300 lb/acre were applied to subsurface-irrigated fields. Nitrogen applied with drip irrigation was applied in graduated weekly amounts from a 4-0-8 (N-P2O5-K2O) solution, which resulted in differing cumulative N rates each year. The total drip-applied N rates were 210 and 311 lb/acre N in fall 1987, 202 and 289 lb/acre N in spring 1988, and 176 and 266 lb/acre N in fall 1988. Fruits were harvested three times each season, except the fall 1988 season, which was abbreviated to two harvests by a tropical storm. Beds were polyethylene mulched and spaced on 6-foot centers each season.
Tomato yields were not affected by fertilizer rate or irrigation method (-10 centibars drip or subsurface irrigation) in fall 1987 or spring 1988 trials, resulting in average yields of 1,724 and 2,557 cartons/acre each year. Lower yields (1,306 cartons/acre) occurred with the 300 lb/acre N, subsurface-irrigated treatment (fall 1988) compared to 1,585 cartons/acre with drip-applied 266 lb/acre N irrigated to -10 centibars of soil moisture. Average yields with 202 and 289 lb/acre N and the drier -15 centibars drip-irrigated treatment were lower, 2,360 cartons/acre, compared to 2,570 cartons/acre average yields with the wetter -10 centibars drip irrigation treatment (spring 1988). With the drier irrigation treatment this season, large-size fruit yields were reduced to 78% of large fruit yields obtained with the -10 centibars and subsurface-irrigated treatments averaged using both N rates. Soil moisture had no effect on marketable fruit or large-size fruit yields in either the fall of 1987 or 1988. Plants with all treatments had high concentrations of N in most recently matured whole leaves taken at first flower and early fruit set, and leaf-tissue N concentrations remained adequate thereafter. All tomato plants initially received 400,000 gallons/acre of subsurface-applied water to raise the water table and establish the transplants. For the remainder of the trial, drip or subsurface irrigation was applied as specified. The total drip-applied water used was one-third of the total subsurface-applied water.
Using whole-plant plus fruit analysis for N and K, drip-irrigated tomatoes were found more efficient in N utilization than the subsurface-irrigated tomato crop (spring 1988). At -15 centibars and 202 lb/acre N, 220 lb/acre N was removed from the soil. The subsurface-irrigated crop removed 163 lb/acre N with 200 lb/acre of applied N and removed 173 lb/acre N with 300 lb/acre of applied N (Clark et al. 1989). Drip irrigation proved to efficiently provide irrigation water and nutrients while maintaining high yields.
A study (Santos 2008) in west central Florida at the UF/IFAS Gulf Coast Research and Education Center evaluated N rates in combination with irrigation programs for effects on tomato (variety 'Florida 47') production during two seasons (fall 2006 and spring 2007). Three rates of N, 200, 250, and 300 lb/acre were combined with irrigation treatments of seepage at 14 acre-inches of water, seepage plus drip irrigation at 14 acre-inches each, and drip irrigation with 14 acre-inches. There was no interaction between treatment and season. Yields averaged 1,720 cartons/acre in this experiment across seasons. Yields were lowest with seepage irrigation only with 200 lb/acre N. Yields were maximized by adding another 14 acre-inches of water to the seepage irrigation treatment via drip irrigation and a total of 200 lb/acre N. These yields were the same as yields with drip irrigation with a total of 14 acre-inches and 200 lb/acre N. Results showed that more N is needed when seepage irrigation is used alone and that drip irrigation used alone or in combination with seepage irrigation allowed for the maximizing of N efficiency for tomato production.
Studies in the spring and fall of 2006 in southern Florida with seepage-irrigated tomato confirmed earlier work with controlled-release fertilizers (Ozores-Hampton et al. 2010). Yields were best when the controlled-release fertilizer was incorporated in the bed compared to when it was placed in the "hot bands" on the surface of the bed. Variable results were observed with controlled-release fertilizers across seasons. In one study, tomato yields were similar with 200 lb/acre N compared with the grower rate of 266 lb/acre N. Applying 50 lb/acre N from a controlled-release fertilizer plus 100 lb N from soluble N (total of 150 lb/acre N) resulted in similar yield to more total N all placed in the hot band. Use of controlled-release N sources appeared to provide an opportunity to reduce total N applications with seepage-irrigated crops.
Research on the Rockland Soils in Miami-Dade County
Limited research results on fertigated tomato grown on Rockdale soils prompted a Dade County fall/winter 1996 study (Carranza et al. 1996). Nitrogen and K were applied in a 4 x 4 factorial experiment. Nitrogen from NH4NO3 was applied 20% preplant and 80% injected, as recommended in the Vegetable Production Guide for Florida at that time (Hochmuth and Maynard 1996). N rates were calculated based on a 6-foot bed spacing. High yields resulted with 150 lb/acre N, 99% RY (1,901 cartons/acre). Yield nearly doubled with 75 lb/acre N (94% RY) compared to yield with the check (zero N) treatment. No significant yield advantage occurred with 225 lb/acre N (100% RY) compared to the yield with 150 lb/acre N. Incidences of graywall and blotchy ripening (BR) in tomato, considered to be K deficiency disorders (Hochmuth et al. 1994a), were also studied at this site. Increasing K from 0 to 150 lb/acre K2O had no effect on graywall or BER on this Dade County soil that tested medium high (AB-DPTA) in K. Nitrogen rate, however, had a positive effect on graywall incidence. Graywall incidence averaged using both tested cultivars increased with N rates from 0 to 225 lb/acre (7–34 cartons/acre). 'Agriset 761' had a 40% higher incidence of graywall than 'Sunny'.
A second study with N and K fertilization of tomato on rockland soils was conducted in the winter season of 1996-1997 (Carranza et al. 1997). The results of this study confirmed the results of the same study conducted in the previous winter season. Total season and extra-large fruit yields responded quadratically to N rate, maximizing with 150 lb/acre N (100% RY at 1,856 cartons/acre). Graywall incidence increased with N rate.
Research in North Central and Northern Florida on Sandy and Loamy Fine Sand Soils
Work with drip irrigation at the North Florida Research and Education Center, Quincy (Rhoads et al. 1988) was designed to test yield responses to N rates ranging from 0 to 200 lb/acre on the same field during the spring 1983, 1984, and 1986 planting seasons. Beds were spaced 6 feet apart, and soil moisture was monitored with tensiometers that were placed 6 inches from the plant and 6 inches deep. A soybean crop grown in 1982 left sufficient residual N to return a total tomato yield of 99% RY (2,640 cartons/acre) with 60 lb/acre N fertilizer for the first crop season. Total yields responded similarly to N rates from 60 to 180 lb/acre in 1984. Slight responses occurred with 120 lb/acre N this season, resulting in 98% RY (2,570 cartons/acre). Yields were similarly unaffected by changes in N source between NH4NO3 and Ca(NO3)2 or between preplant N application and split N applications — 40% preplant and 60% injected. In 1986, total tomato yields increased with N rates through 200 lb/acre (2,850 cartons/acre, 100% RY) and were also unaffected by preplant or injected fertilizer treatments.
Petiole sap nitrate concentrations were positively correlated with optimum tomato yields in the South Florida subsurface-irrigated trials summarized above, and optimum sap nitrate and K ranges were published (Hochmuth 1994a, b). Researchers at the North Florida Research and Education Center, Quincy, designed spring and fall 1995 experiments to test these petiole sap nitrate levels against optimum tomato yields on a drip-irrigated North Florida research site (Rhoads et al. 1996). In the spring, N was applied either 100% preplant-incorporated or 40% preplant-incorporated with 10% injected six times between 3 and 13 weeks from transplanting. All N was applied preplant in the fall. Five N rates (0, 60, 120, 180, and 240 lb/acre) were applied from NH4NO3 in both seasons. The beds were spaced 6 feet apart and mulched with black polyethylene in the spring and white in the fall. Tomato varieties were 'Solar Set' in the fall and 'Colonial' in the spring.
Battery-operated ion-specific meters provided instant sap nitrate analysis, and results were comparable to the South Florida standards derived with colorimetric procedures (Hochmuth 1994a, b). Tomato yields were highly correlated with petiole sap nitrate concentrations for the period of 4–10 weeks after planting (Rhoads et al. 1996). Researchers concluded that field ion meters were effective for mid-season monitoring of tomato N needs. Tomato yield responded to N more in the spring (higher yielding crop) than in the fall. Yields with preplant-applied fertilizers were not different with N at rates greater than 120 lb/acre N in spring and more than 60 lb/acre N in fall trials. Reduced fall yields (1,280 cartons/acre) pointed to other factors that limited tomato yield, such as poor fruit set due to high day/night temperatures. Authors noted that tomato yields would likely respond to N rates closer to the recommended rate at that time (175 lb/acre) during a cooler season. The highest yielding plants were grown with 180 lb/acre N applied 40% preplant and the remainder injected (100% RY; 2,268 cartons/acre). These yields were 7% higher than yields of plants with 100% preplant-applied fertilizer with the same N rate.
The Quincy, Florida, area produces both a spring and fall tomato crop. A lack of experiments with fall-grown tomato in north Florida prompted 1995 (variety 'Solar Set') and 1997 ('Equinox') experiments in Gadsden County (Rhoads 1997). Particular attention was given to petiole sap NO3-N concentrations measured with ion-specific meters. Nitrogen was applied 100% preplant from NH4NO3 at N rates of 0, 60, 120, 180, and 240 lb/acre. Yields were different between seasons with totals of 1,300 25 lb cartons in 1995 and 2,100 cartons in 1997. High temperatures in fall 1995 were suspected of limiting yield response to N applied that season (due to poor fruit set). Significant yield responses to N fertilization occurred in both seasons. In both seasons 95% and 96% RY (1,260 and 1,996 cartons/acre, respectively) occurred with the 60 lb/acre N rate. Yield increases to 100% RY with 180 lb/acre N (1,332 cartons/acre) in 1995 and 120 lb/acre N (2,082 cartons/acre) in 1997 were insignificant. Comparison of petiole sap NO3-N concentrations averaged using both seasons confirmed a range of N sufficiency concentrations (Hochmuth 1994a, b) in plants grown with 60 lb/acre N (low) to 120 lb/acre N (high). An adequate N rate for fall-grown tomato was given as 120 lb/acre. Researchers suggested that N fertilization efficiency may be improved by applying 40 lb/acre N at preplant and 15 lb/acre N injected weekly from weeks 5 through 8. The weekly injected N rate may be adjusted to meet petiole sap N sufficiency concentrations monitored through week ten.
A study was conducted with drip-irrigated tomato ('Colonial') to evaluate various plant petiole sap nitrogenous compounds for their value as a predictor of tomato yield (Andersen et al. 1999). Preplant applied N rates of 0, 60, 120, 180, and 240 lb/acre N were used in the study. Petiole sap was collected at 7 and 13 weeks after transplanting, and the concentrations of free nitrate-N, free amino acids, total amino acids, and total N (sum of free nitrate-N and amino acid N) were evaluated as a function of applied fertilizer N. These compounds were also used as predictors of fruit yield. At 7 weeks after planting, nitrate-N concentration and the concentrations of 15 of 18 of the free amino acids were correlated with fertilization rate, but concentrations of bound amino acids and total amino acid concentration were not. At 13 weeks after planting, most of the correlations observed at 7 weeks did not persist. Concentration of nitrate-N and total N in the sap and N fertilization rate were correlated with marketable fruit yield. Marketable fruit yield was maximized between 120 and 180 lb/acre N.
Rhoads et al. (1999) studied the impacts of N fertilization rates and various ground cover treatments on yield and nitrate movement below the crop on a loamy fine sand soil in Quincy, Florida. Ground cover treatments included polyethylene mulch for the tomato crop, fallow, and a cover crop (sorghum-sudan grass following the spring tomato crop and annual ryegrass following the fall tomato crop). Nitrogen rate varied from 0 to 300 lb/acre for the spring and fall crops of 1998, and the recommended rate was 175 lb/acre N at the time of this study.
Yields were 1,900–2,600 cartons/acre in the spring season and 1,300–2,700 cartons/acre in the fall crop. Yields did not respond to N rates greater than 180 lb/acre but residual soil nitrate-N did increase.
Findings in spring 1993 (Quincy) and spring 1994 (Gainesville) also indicated that petiole sap N concentrations at mid-season correlated best with yield (Locascio et al. 1997b). Beds spaced 6 feet apart were mulched with polyethylene and irrigated when soil water tension reached -10 centibars. The total applied N was 175 lb/acre at both sites. Yields were 16% higher when 60% of the N and K fertilizers were injected than when all of the fertilizers were applied preplant (1,328 cartons/acre) at Gainesville (sandy soils). Tomato yields in the Quincy trials (finer textured soils) were either unaffected by application method or had 16% higher yields with all preplant-applied fertilizer than with split-applied N (1,738 cartons/acre). Petiole sap nitrate concentrations decreased during the season (adequate) but the rate of decrease was less when some of the N was injected during the season compared to when all the N was applied preplant.
Yield responses to the time of N and K application, either preplant only or preplant with fertigation, depended on soil type. Less yield response resulted with fertigated N on coarse textured soils, such as Orangeburg loamy sands in Quincy, compared to the finer textured Arredondo fine sands in Gainesville. Earlier research supported this finding (Locascio et al. 1989). Yields averaged using 1984 and 1985 on Arredondo fine sands resulted in increased late-season extra-large and large fruit yields (489 and 360 cartons/acre, respectively) with 60% drip-applied N and K compared to yield response with all preplant-applied N and K (300 and 293 cartons/acre, respectively). Researchers noted that drip-applied nutrients extended the season of large fruit harvest by maintaining plant nutrient concentrations late in the season. Results of this experiment were repeated in 1988 at Gainesville (Locascio and Smajstrla 1989). When 60% of the applied N and K were fertigated, yields of extra-large fruits were 40% higher than extra-large fruit yields where N and K were applied 100% preplant. Yields of large and total marketable fruits were also 10% higher with the same fertigated treatment compared to 100% preplant-applied fertilizer. When percentages of fertigated N and K were exceeded 50%, 75%, and 100%, yields declined linearly (2473, 2459, and 2060 cartons/acre, respectively) in other Arredondo fine sand fertigation research (Dangler and Locascio 1990). The 1984 and 1985 seasons resulted in higher yields of medium-size early fruit, mid-season large fruit, and total season large fruit with 50% fertigated N and K (or N alone).
Experimentation with fertigation continued in Gainesville on Millhopper fine sand soil in the spring of 1996 (Locascio et al. 1996). Two-foot wide beds were prepared on six-foot centers and 40% of the total N was broadcast on the bed surface, tilled, and mulched with polyethylene. 'Agriset 761' tomato transplants were set March 14, and fertigation began April 3. Equal amounts of N were applied each week through ten weeks of fertigation. Total N (NH4NO3) rates were 120, 180, 240, or 300 lb/acre. Drip irrigation was applied to maintain soil moisture at -10 centibars by tensiometer with one overhead irrigation event for freeze protection. Marketable yields responded quadratically to increasing N rates leveling off at rates exceeding 180 lb/acre N with 240 and 300 lb/acre N. Respective RYs for these three N rates were 88%, 98%, and 100% RY (2,270 cartons/acre). Leaf tissue N concentrations were adequate (2.5%–4.0%) at rates more than 180 lb/acre N (> 2.7%).
In an N fertilization study performed by Andersen et al. (1999), a positive correlation resulted between N rates applied and NO3-N in the petiole sap. The 'Colonial' tomato variety was used with preplant N rates of 0, 60, 120, 180, and 240 lb/acre. The amount of NO3-N accounted for 37% of the total N in the 0 nitrogen treatment while NO3-N accounted for 83% of the total N in the 180 lb/acre. There was a high correlation (R2= 0.98) between the total N and the NO3-N for the hydrolyzed and nonhydrolyzed sap. This work also showed that the maximum yield was obtained with an N application rate (180 lb/acre) in agreement with the UF/IFAS recommended rate.
In addition to investigating the response of tomato fruit yield to N rates in the production field, Vavrina et al. (1998) investigated the impacts of tomato transplant fertilization on subsequent fruit yield in 1993-1995. Fall-grown transplants were larger than spring-grown plants. All transplant growth parameters, stem length, leaf area and number, and root to shoot ratio responded quadratically to increasing N concentration (from 0 to 75 ppm) in the nutrient solution applied to transplants in the greenhouse. In the spring, tomato fruit production and extra-large fruit production increased with increasing N concentration in the transplant house, but the opposite trend occurred in the fall. These results stressed the importance of season on N fertilization of transplants to gain the best potential yield in the field.
Most tomato research with fertilizer rates has been conducted with round-fruited tomatoes. Grape tomatoes have gained in popularity during the last 15 years in Florida. The grape tomato varieties have become popular with consumers because of potential health benefits, sweetness, flavor, and ease of consumption (Simonne et al. 2007). A study with 'Tami', a red grape tomato, was conducted with drip irrigation in Live Oak (Simonne et al. 2008). The N treatments included 0%, 33%, 66%, 100%, 133%, and 166% of the recommended N rate of 200 lb/acre. Grape tomatoes can produce fruit throughout a longer growing season than their more determinant round tomato variety counterparts. In this experiment, yield responses to N rate were quadratic in two seasons. Highest marketable yield of 36,000 lb/acre with 314 lb/acre N occurred in the first season, spring 2005, and 33,000 lb/acre with 280 lb/acre N in the second season, spring 2006. Because of the potential for a long growing season (indeterminate growth habit), authors suggested making N recommendations for grape tomatoes based on a daily N need rather than a total seasonal rate. Total-seasonal N requirements may be greater for grape tomatoes than for round tomatoes.
A study was conducted by Simonne et al. (2008), at the North Florida Research and Education Center, Suwannee Valley near Live Oak, FL, using 0%, 33%, 66%, 100%, 133%, and 166% of the current recommended N rate for round tomato (200 lb/acre) with three weekly injections of 20 lb/acre of N each in the 100% rate. The results from that study suggest that N fertilization for grape tomato grown in spring with plasticulture could be done by incorporating 50lb/acre N in the bed, followed by daily rates ranging from 0.4 to 3.0 lb/acre/day. Because the length of the growing season for grape tomato may vary, emphasis should be placed on daily N rates and irrigation management rather than on seasonal N rate.
Numerous drip-irrigation trials summarized above were treated with soluble N sources applied at preplant or applied at preplant and then fertigated throughout the crop season. Tomato yields generally maximized at or near the previous UF/IFAS recommended rate of 175 lb/acre N during that time (1990s). Researchers field-tested a polymer-coated fertilizer (Meister fertilizer; Helena Chem. Co., Memphis, TN), hoping to improve the efficiency of N fertilizer on tomato (Hochmuth 1997). Trials were conducted on Arredondo fine sands near Gainesville in spring 1997 on raised two-foot wide beds placed on four-foot centers (N rate calculations and expressions were based on six-foot bed centers). The polymer-coated, 19 N-5 P2O5-14 K2O, fertilizer was compared to soluble N and K sources (NH4NO3 and KCl). Fertilizers applied to the bed surface were broadcast and tilled or applied in a four-inch off-center band and pressed into the soil. All beds were mulched with black polyethylene, planted with 'Agriset 761' tomato, and drip-irrigated to -10 centibars soil moisture.
Interactions occurred in the first, third, and overall season harvests affecting marketable fruit yields, all fruit grades initially, and then only extra-large and large fruit yields. Yields of early fruit from all fruit grades increased when soluble fertilizers were broadcast applied and when polymer-coated fertilizer was placed in bands (yields averaged using all applied N rates). Total marketable yields and yields of large fruit also increased with the same fertilizer placement methods. Nitrogen rate increases with the polymer-coated material resulted in higher early yields; more extra-large, large, and total marketable fruit yield resulted with increasing N rates from 75 to 175 lb/acre N at the third harvest. With the soluble N source, yields decreased slightly with higher N rates. Overall, plants fertilized with the polymer-coated fertilizer produced 40%–50% more fruits than plants fertilized with soluble N (3,326 compared to 1,859 cartons/acre, respectively) and resulted in significantly higher yields, 80%, 86%, and 100% RY (3,326 cartons/acre) with N rates of 75, 125, and 175 lb/acre N. Yields exceeding the state average yields of 1,216 cartons/acre (1996) were likely due to increased plant density with plant rows spaced four feet instead of the standard six feet on center. Cull fruit yields were similar with both fertilizers and were not changed by fertilizer placement or rate.
Additional work was conducted in the fall 1997 with controlled-release fertilizers (Hochmuth 1998a) to evaluate rates and placement methods with 'Agriset 761' tomato variety. Total yield was best with 175 lb/acre N and also with broadcast/incorporation in the bed (highest yield for this fall crop was 1,550 cartons/acre). Early and total marketable yields were greater with controlled-release fertilizer compared with soluble fertilizer. Total amount of fertilizer could be reduced slightly with a controlled-release source compared to all-soluble fertilizer. Foliar controlled-release N did not affect tomato yields.
In the spring 1998 season, various fertility programs were compared, including combinations of controlled-release fertilizer (CRF) and soluble fertilizers (Hochmuth 1998b) for 'Agri Set 761'. Yields were high and averaged 2,990 cartons/acre. The use of the controlled-release fertilizer in various combinations (100%–20% CRF) with soluble fertilizer applied preplant resulted in the same yields as the standard program of fertigation. One application of fertilizer at preplant saved on the time involved with fertilizer injections during the season.
A greenhouse study found that leaching of N was greatest with a soluble fertilizer source compared with several controlled-release sources (Fan and Li 2009). Leaching was greatest with sandy soils compared to loam soil. Up to 33% of the applied N leached with the sandy soil, compared to 6% with the loam soil.
Tomato studies with CRF showed promise for the use of CRF in tomato production. There appears to be opportunity for reducing N rates from the UF/IFAS current recommended rate of 200 lb/acre N, or at least minimizing the need for extra N. Controlled-release fertilizers need to be incorporated in the soil for best efficiency. Controlled-release fertilizers seem to have their best application in seepage-irrigated tomato crops, but CRF sources also have promise for use in drip-irrigated crops if the management of injecting fertilizers is problematic for the grower. These products also have promise for reducing the potential for N losses due to leaching in the season.
Responses to N Rate over all Irrigation Methods
Tomato yield responses to N rates using all studies with all irrigation methods are presented in Figure 4. Results from more than 60 experiments show that 80% RY is achieved once N rate of more than 100 lb/acre is applied. Most of the treatment means are at least 90% RY in the range of 100–300 lb/acre N. There is an indication that N rates in excess of 300 lb/acre lead to reductions in yield.
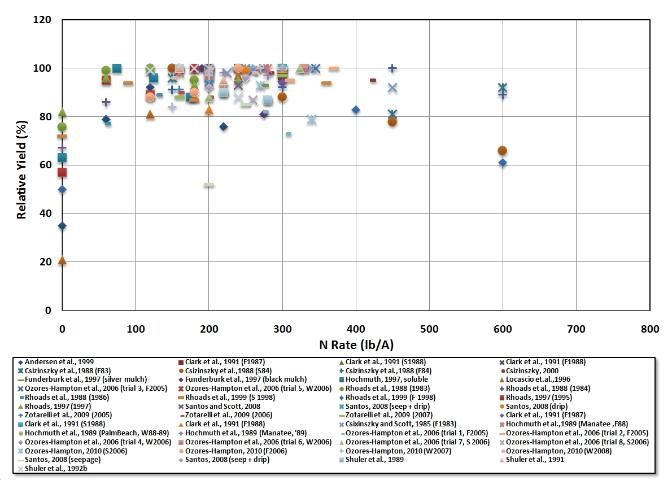
Research Investigating the Interaction of Fertilization and Pest Problems
Several additional aspects of plant growth and health have been studied in Florida in relation to N management. Studies have been conducted to evaluate fertilizer rate on disease and insect severity.
Csizinszky et al. (1988) applied various rates of N and K to tomatoes that had been inoculated with the bacterial spot organism in three seasons, fall 1983, fall 1984, and spring 1984. N and K rates of 150, 300, and 450 lb/acre N were combined with K2O rates of 300, 600, and 1,200 lb/acre. These researchers found that increasing N rate reduced the disease severity in one study out of three. The concentration of N in the leaf tissue was negatively correlated with disease severity, while calcium and magnesium concentrations were positively correlated with disease severity. N rates affected tomato yields in one out of three years, and best yields were obtained with 150 lb/acre N and 300 lb/acre K2O (100% RY: 1,021 cartons/acre). Fertilizer treatment had no effects on yield in the fall seasons. Yields averaged 1,128 (fall 1983) and 1,547 cartons/acre (fall 1984).
Over-fertilization with N has been measured in terms of reduced yield, but additional findings tie high rates of N application (>175 lb/acre) with increased populations of western flower thrips (Funderburk et al. 1997). Because western flower thrips is a vector of tomato spotted wilt virus, increased populations resulted in higher disease incidence in Quincy-grown tomatoes (1996 and 1997). Plants were fertilized with 190 or 275 lb/acre N, mulched with black or silver plastic, and drip-irrigated. Beds were spaced 6-feet apart. Pesticides were not applied to thrips or their natural predators. In 1997, tests were also conducted to see the effect of N rates (0, 60, 120, 180, and 240 lb/acre) on flower N concentration, amino acid quality, flower number, and plant size.
In 1996, adult thrips populations were generally lowest on silver-mulched beds fertilized with the recommended N rate. When measured in 1997, occurrence of tomato spotted wilt virus was lowest (23.6%) on silver-mulched beds fertilized with the recommended N rate (there was 30% disease occurrence with the higher N rate). Disease occurrence was highest (46.9%) where black plastic was applied to beds fertilized with the higher N rate (there was 40% disease occurrence with the recommended N rate). As a simple disease control measure, researchers recommended use of silver mulch with the recommended N rate. Analysis of flower tissue was not completed for the study, but analysis of plant sepal tissue revealed higher protein concentrations in the sepals of plants fertilized with excess N. Although thrips are commonly seen clustered on tomato plant sepals, researchers advised additional study to confirm an association. In general, increased N fertilization significantly increased plant size and flower number in both seasons without a subsequent yield increase.
Interactions of Fertilization and Fruit Chemical Quality
Simonne et al. (2007) conducted a study at the North Florida Research and Education Center, Suwannee Valley near Live Oak, Florida, on a Lakeland fine sand to investigate the effects of N rate on the chemical characteristics of grape tomatoes. This study used N rates of 0, 70, 140, 210, 280, and 350 lb/acre N. Tomatoes were harvested at full-ripe stage. Increasing N rate resulted in a decrease in vitamin C content from 44 to 35 mg per 200 grams, and a reduction in titratable acidity from 0.47% to 0.38% citric acid. Lutein, beta carotene, and color were not affected by N rate. Soluble solids decreased with N rate for the first harvest but increased with N rate for second harvest. Although there were slight treatment effects, the authors concluded that overall N rate had little impact on selected quality parameters.
Studies on Fertilizer and Irrigation Management, Cover Crops, and Environmental Impacts
Irrigated area in Florida has increased (Smajstrla and Haman 1998). Many types of irrigation are practiced in the state (Dukes et al. 2010). High water uniformity is required for high fertilizer uniformity if fertilizer is to be injected through the drip irrigation system (fertigation) and certain management practices are needed for fertigation to prevent nutrient leaching (Dukes et al. 2010). Splitting the irrigation event into several short duration events is expected to result in decreased wetted depth and increased wetted width. Using a total of 4 hours of irrigation, Poh et al. (2009) evaluated the effect of variations in application alterations during the four hours of irrigation. The effect of the alterations was not significant for width and depth. The authors pointed out that these results did not contradict the recommendation to split irrigation events. The irrigation event was four hours in this test, and longer periods of irrigation would need to be evaluated.
Irrigation management and fertilizer management practices should be practiced in concert to keep water and nutrients in the root zone. This principle has been behind considerable research to find ways to better conserve water and fertilizer, particularly nitrogen (Hochmuth 1992a, b; Hochmuth 2000; Hochmuth and Hanlon 2010a, b). Recommendations for irrigation management for tomato and other vegetables have been written to reflect the science (Simonne et al. 2010).
In a set of three trials during 1992, 1993, and 1994, Stanley and Clark (2003) evaluated fertilizer programs for tomatoes, using the fully-enclosed seep system (FES). The FES was shown to save up to 30%–40% in irrigation applications (Stanley and Clark 1991). Three water table level treatments of 18, 24, and 30 inches below the top of the bed were used in combination with three fertilizer rates. The fertilizer programs were 192-100-221, 276-100-318, and 360-100-415 (N-P2O5-K2O). There were no interactions between irrigation and fertilizer treatments. There were no differences for fruit yields among fertilizer treatments. There was no advantage to maintaining a water table at shallower depths than 24 inches. The authors concluded that growers using seepage irrigation can employ more effective N management as follows:
- Use the FES to maintain a water table near 24 inches below the bed surface.
- Reduce N rates to the recommended rate of 200 lb/acre.
- Maintain a lower water table to encourage a more expansive root system that would better explore the root zone for N.
A fall 1997 experiment was conducted to evaluate rates (5, 10, or 15 tons/acre) and placement (narrow band or wide band on the pre-bed before final bed formation) of compost from Disney World with irrigation rates and N and K sources for 'Florida 47' tomato (Csizinszky and Stanley 1998). Total yield was best with 0.6 times pan evaporation irrigation. The advantage compared to the control treatment of adding 5 or 10 tons/acre of compost plus pre-plant dry fertilizer was observed only for total season yield. There were high residual concentrations of P, K, Ca, Mg, and Fe where compost was used. Authors pointed out the possible problems of nutrient buildup in soils treated with compost.
Nitrate-N levels in multilevel samples were assessed for vegetable fields in southwest Florida during 1990 and 1991 (McNeal et al. 1994; 1995). Nitrate-N concentrations below vegetable fields, including tomato, showed large peaks up to 130 ppm in the early part of the growing season. These spikes did not persist, and most of the samples from vegetable fields were less than 1 ppm. Off-site movement of water or lateral subsurface movement was not supported by the data. The authors postulated that gaseous losses of N from denitrification may remediate the periodic spikes in nitrate. The authors suggested that these results have implications for attempts to characterize nutrient loading from vegetable fields in southern Florida with high water tables. Nutrient loads cannot be calculated with certainty from infrequent sampling of water beneath the vegetable crop.
Nitrate-N concentrations were monitored in and around a vegetable field at the North Florida Research and Education Center near Live Oak, Florida, in 2001-2005 (Simonne et al. 2006). Treatments involved levels of drip irrigation water and N fertilizer ranging from 100% to 300% of the recommended practices. The objectives were to monitor trends in nitrate in groundwater 20 feet deep, identify seasonal peaks, and determine if rainfall affects nitrate concentrations. Various crops were grown on the site through the years, including watermelon, tomato, and pumpkin. Nitrate-N concentrations ranged from 0 to 45 ppm during the 5-year period. Maximum annual concentrations were greater in the down-gradient wells from the field. All well samples were less than 10 ppm nitrate-N in 2003 with the watermelon crop. High rainfall associated with Hurricane Jeanne and Frances in 2004 did not dilute the N concentrations as was expected.
Tomato growers in Homestead grow tomatoes on a gravelly soil and in the 1990s switched from sprinkler irrigation to drip irrigation. Many growers wanted to know how to manage drip irrigation. Tensiometers were evaluated as a method for monitoring soil moisture and scheduling irrigation events for tomatoes in Homestead (Li et al. 1998). Treatments included four irrigation rates and three fertilizer programs. Irrigation programs were -5, -10, -15, and -20 centibars, and the fertilizer treatments were three sources of fertilizer. Yields were low overall due to yellow leaf curl virus. Optimal irrigation was with the -10 centibar soil moisture level, and fertilizer source did not affect tomato yield. The usefulness of tensiometers for irrigation management on the gravelly soils in Homestead was demonstrated by Olczyk et al. (2000). Tomato growers used traditional approaches to irrigation management by stage of growth or by visual estimation of water needs. Tensiometers were demonstrated on a commercial field. Reductions in water use did not lead to reductions in yield. Marketable yield was 2,641–3,146 cartons/acre. There was a 27% reduction in water use with tensiometers.
A low volume/high frequency soil moisture-based drip irrigation system was compared with the grower method on a commercial tomato farm near Miami, Florida (Muñoz-Carpena et al. 2003; 2005). Tomato yields were the same as with the commercial grower program for several new control treatments. Water savings were observed when 100% of the crop water needs were applied in high frequency compared to the grower program of less frequent, longer run times. Increased savings were observed when high frequency low volume applications were made using feedback soil moisture controls. Switching tensiometers at -15 centibar performed the best, saving 74% water. Some maintenance was required. Granular matrix sensors behaved erratically.
Work done to evaluate the effectiveness of low volume/high frequency (LVHF) soil moisture-based drip irrigation systems was done in Miami, Florida, on a commercial tomato farm (Muñoz-Carpena 2005). The experiment was conducted using 'Florida 47' tomato plants on a 1.5 acre plot of land with Dade fine sand overlaying porous limestone. Pre-plant dry fertilizer (6N-2.6P-10K) was used at a rate of 1,431 lb/acre with dissolved fertilizer (4N-0P2O5-6.7K2O) applied weekly at a rate of 18 lb/acre for the final 5 weeks. Treatments were applied to 600-foot beds and consisted of six LVHF treatments and one control (conventional grower irrigation rate). The automation of the treatment irrigation systems was done with tensiometers and granular mix sensors (GMS). The LVHF systems were able to reduce water use by 39%–51% for the tensiometer-controlled systems and 2%–7% for the GMS. There was no yield difference for LVHF-irrigated tomatoes compared to the yields of the control (irrigated according to standard commercial schedule). The control used approximately 81% more water than the maximum crop water needs for the area.
Water and nutrient efficiency depends on the interaction between N-fertilizer rates and irrigation scheduling on yield, irrigation water use efficiency, and root distribution of tomato cultivated in plastic mulch/drip-irrigated production systems. Experiments were conducted near Citra, Florida, during the spring of 2005, 2006, and 2007 (Zotarelli et al. 2009a, b). Three different N-rates were applied at 160, 180, and 220 lb/acre N. Irrigation treatments applied were 1) surface drip irrigation (SUR), 2) subsurface drip irrigation (SDI), and 3) TIME with irrigation and fertigation lines placed as in SUR and irrigation being applied once a day. Except for the TIME treatment, remaining treatments were controlled by soil moisture sensor-based (SMS) irrigation. There was no significant difference in the tomato yield with irrigation method and N-rate treatments, but the irrigation rates had a significant effect. SUR treatment resulted in the application of 15%–51% less irrigation water whereas SDI treatment resulted in 7%–29% compared to TIME. Tomato yield increased by 11%–26% for SUR and SDI treatments compared to TIME (whereas N-rates exceeding 145 lb/acre did not affect yield). SDI- and SMS-based treatments consistently increased tomato yields while reducing irrigation water use and potential N leaching.
The conservation of soil and water nutrients can be accomplished simultaneously with increased tomato yields by implementing the correct planting and crop and soil management practices.
Soil microbial biomass plays an important role in the mineralization of soil organic matter, nutrient cycling, and retention. Though microbial biomass represents only a fraction of the total amount of soil carbon (C) and nitrogen (N), this biomass has relatively rapid turnover. Cover crops along with other kinds of organic materials like compost or manure applied to the soil may stimulate soil microbial activity and may change the amount of the soil microbial biomass. The experiment conducted at the Tropical Research and Educational Center, Homestead, Florida, with a split plot design and four irrigation rates showed that cover crops may play an important role in reducing nutrient leaching (Wang et al. 2007). Cover crops used in this experiment included sunn hemp, velvetbean, cowpea, sorghum sudangrass, and a fallow plot. Compared to fallow land, soil total C was higher using cowpea, and soil total N was higher with sunn hemp, velvetbean, and cowpea. Tomato yields were significantly higher in the treatments with sunn hemp, velvetbean, and sorghum sudangrass than with cowpea. The lignin concentration in cowpea was significantly higher in stems, leaves, and roots than in sunn hemp and sorghum sudangrass. Thus, with the cowpea treatment, the level of microbial biomass N was not significantly higher than in the sorghum sudangrass and fallow treatments and significantly lower than the level of biomass in the treatments with sunn hemp and velvetbean. This finding implied that after incorporation into the soil, sunn hemp and velvetbean may more quickly supply subsequent crops with plant-available N than the other cover crops, which played an important role in determining the growth and yield of the subsequent tomato crop.
A container experiment to evaluate leaching from various cover crop species was conducted in Homestead, Florida (Wang and Klassen 2005). Leachate was collected from the pots throughout a 6-week period. In the fallow pots, 21% of the N was lost to leaching, but only 1%–3% was leached with the cover crops. Losses of P were negligible with all treatments, but were half as much with cover crops compared with fallow. In 2002, a field experiment was conducted to evaluate cover crops on the fruit yield of the subsequent winter tomato crop (Wang et al. 2003). The largest cover crop biomass was produced by sunn hemp, followed in order by velvetbean, cowpea, and sorghum sudangrass. Sunn hemp as the cover crop resulted in an increase of tomato plant biomass and early fruit yield.
In a two-year study (2001 and 2002) in Homestead, Florida, Wang et al. (2001; 2002; 2005) found that growing summer cover crops such as sunn hemp and cowpea in combination with reduced irrigation improves soil fertility and tomato yields. The greatest N contribution was made by sunn hemp and the least by sorghum sudangrass. Tomato yields increased from 14% to 27% greater than the control (weed-free fallow) by growing cover crops. Maintaining soil moisture tension at -10, -20, or -30 centibars on a tensiometer improved yields compared to the control of -0.5 centibars, the commonly used grower practice. Maintaining a soil moisture tension of -30 centibars saved 85% water compared to the control. The authors concluded that growing N-fixing cover crops and reducing irrigation rates were two valuable approaches to conserving water and nutrients in a tropical production area. Studies were also supported with pot experiments (Wang et al. 2005) that showed legume cover crop improved tomato fruit production and also reduced leaching of N and P.
In another publication, Wang et al. (2009) recommended growing cover crops as green manures to increase soil N availability to tomatoes. This study showed that either the use of the cover crop sunn hemp or the addition of compost (at a rate of 22–33 tons/acre) as a source of organic matter increased the availability of soil N and also the total marketable yield of tomato crops subsequently grown. The authors mentioned that care must be taken to ensure that the C:N ratios in the shoots of leguminous cover crops are not high because this will affect soil N availability and tomato yields.
The effects of summer cover crops and compost on tomato production were evaluated in two crop cycles in 2003 to 2005 in Homestead, Florida (Wang et al. 2009). Cover crops were sunn hemp, velvetbean, cowpea, and sorghum sudangrass. Compost was applied at 11, 22, and 33 tons/acre. Sunn hemp accumulated up to 280 lb/acre N. Yields of extra-large tomato fruits increased with the use of sunn hemp cover crop and the higher rates of compost.
N recovery and N use efficiency were the topic of several studies involving irrigation and N fertilization. Fan and Li (2009) noted that high rates of N fertilizer application may reduce nitrogen use efficiencies (NUE) and contribute to groundwater pollution. Fan and Li recommended that fertilizer formulations should include slow-release fertilizers to reduce the leaching potential of NO3N in sandy soils.
In an early study on N recovery, Sweeney et al. (1987) studied the effects of irrigation method, N source, and mulch using 15N depleted ammonium nitrate. Neither tomato yield nor N uptake was affected by irrigation method (drip or sprinkler irrigation). Mulch use led to increases in tomato yield and N uptake. An average of 65% of the total 200 lb/acre N in the fruit and plant originated from the fertilizer. Highest N recovery was from plants grown with mulch and sprinkler irrigation. With this system, 53% of the N was in the plant and 42% was left in the soil. Only 5% was lost. Up to 46% of the N was lost with other treatments, especially those involving bare ground production.
Irrigation practices play an important role in obtaining good nitrogen use efficiency (NUE). Scholberg et al. (2000) conducted a study to evaluate N stress on tomato growth. The authors conducted growth analyses using data from several studies in Florida. With increasing N, average leaf area index increased but net radiation use efficiency increased at a lower rate, which was related to the decline in leaf N concentration. Greater NUEs can be obtained by increasing plant densities and regulating irrigation. N recovery as a portion of the fertilizer applied decreased as the N rate increased. Fertilizer N recoveries ranged from 36% to 74%. This study also found that less than 10% of N was taken up during the initial growth stages. It was therefore suggested that the apparent nitrogen recovery (ANR) for tomatoes on course-textured soils may be improved by reducing preplant N to as little as 20% of total N.
Excess nutrients are subject to leaching and runoff in the sub-irrigated tomato fields of southern Florida. A study was conducted in the spring and winter growing seasons of 2006 to describe the spatial and temporal nutrient distribution in tomato soil beds (Sato et al. 2009a). Ammonium-N was greatest in the first week after planting in the 0 to 4-inch soil depth below the fertilizer band and did not move vertically. Nitrate-N concentrations found in the 0 to 4-inch depth at 4 weeks after planting indicated about 3 weeks of nitrification in the spring. This time frame was shortened to 1–2 weeks in the winter growing season due to the planting during warm conditions in the late fall. There was both lateral and vertical movement of nitrate-N in the spring to 8–12 inches due to fluctuations in the water table. Distributions were not affected by N rate, but the higher N rate resulted in greater N concentrations in the soil throughout the season, which led to greater risk for N losses. Nutrient and moisture gradients can be maintained in the root zone, but fluctuating water tables must be avoided either from natural or man-made causes.
Water management is critical for preventing N leaching. For Extension programs, the challenge is to get growers to learn about the new technologies that help them manage nutrients and water. One way to demonstrate water management and its impact on nutrient movement is with the "dye test" (Simonne et al. 2003). Drip irrigation systems were operated for varying times, and depth of water movement was traced with blue food dye. On a fine sandy loam in Quincy the water front reached 10 inches in 2–4 hours with application of 30 gallons per 100 feet per hour. On a deep sandy soil in Live Oak, the wetted front passed the 12-inch depth after 3 hours. On a soil in southern Florida with a spodic horizon, the wetted front reached the spodic layer after 4 hours. Knowing the soil water holding capacity and the drip system specifications can help growers apply correct volumes of water without the potential for leaching N.
R. Hochmuth et al. (2005) described on-farm demonstrations for soil water movement with vegetables grown with plasticulture. Using the colored dye approach, these workers applied water with dye for varying irrigation run-times and then dug in the bed to show the depth and later movement of the dye. Movement of the dye was less on loamy sand soils compared with sandy soil. Educational efforts should focus on the water management program early in the season since this period is when the most potential for excessive irrigation occurs. Growers participating in this educational effort became more aware of the impact irrigation management can have on nutrient leaching. One grower reduced his in-bed fertilizer amount after seeing considerable fertilizer loss after his irrigation program had operated early in the season.
Further work by Wang and Klassen (2007) at the Tropical Research and Education Center in Homestead, Florida, used a split-plot design and four irrigation rates to show the importance of cover crops in reducing N leaching. The research suggested that cover crops along with other kinds of organic materials (i.e., compost or manure) applied to the soil may stimulate soil microbial activity and may increase the amount of the soil microbial biomass, which helps with nutrient recycling and retention. Cover crops used in this experiment were sunn hemp, velvetbean, cowpea, sorghum sudangrass, and a fallow plot. Compared to the fallow land, soil total C was higher with cowpea and soil total N was higher using sunn hemp, velvetbean, and cowpea. Tomato yields were significantly higher in the treatments with sunn hemp, velvetbean, and sorghum sudangrass than with cowpea. The lignin concentration in cowpea was significantly higher in stems, leaves, and roots than in sunn hemp and sorghum sudangrass. Thus, in the cowpea treatment the level of microbial biomass N was not significantly higher than in the sorghum sudangrass and fallow treatments and significantly lower than in the sunn hemp and velvetbean treatments. This finding implies that after incorporation into the soil, sunn hemp and velvetbean may more quickly supply subsequent crops with plant available N than the other cover crops, which played an important role in determining the growth and yield of the subsequent tomato crop.
In another study on irrigation with drip-irrigated tomato, Scholberg and Locascio (1999) evaluated the effects of salinity and irrigation method on tomato. Greenhouse studies were conducted with irrigation water with salinity ranging from 1 to 4 decisiemens per meter. Fruit production was greater with drip irrigation than with sub-irrigation and was related to the increased level of salinity in the soil. Tomato stem and leaf weights were greatest at intermediate salinity applied during flowering. Marketable fruit production of tomato decreased quadratically with increasing salinity.
Nitrogen Summary
Nitrogen was shown to be important in reaching maximum fruit yields in tomato. Studies on tomato yield responses to N rate showed the following results:
- Rare yield increases occurred with N rate exceeding 200 lb/acre.
- Often the grower N rate, typically more than 300 lb/acre N, resulted in lower yields compared with lower rates, often with lower first harvest yields and smaller sizes (the most valuable).
- When yields increased with rates greater than 200 lb/acre N, the increases were more often than not associated with increases in yields of later-season fruit production and smaller fruits.
- Recommended N rates were associated with increases in yields of large and extra-large fruits.
- Equal yields occurred with less N using fertigation compared with banded fertilizer with seepage irrigation.
- More soluble N is possibly needed with seepage (sub-irrigation) irrigated crops than with drip-irrigated crops due to reduced N efficiency with seepage irrigation.
- Controlled-release fertilizers led to possibilities for reducing N applications with seepage-irrigated tomato.
- Drip irrigation led to reduced irrigation water use; soil moisture tension of -10 centibars was often better for yield than drier soil conditions (-20 to 130 cbs).
- Drip irrigation with the recommended N rate led to greater N removal (greater N use efficiency) compared with subsurface irrigated crops.
- More graywall disorder occurred with higher N rates.
- Studies in northern Florida showed that tomato yield did not increase with more than the recommended N rate but that leached N amounts did increase.
There have been many studies on the relationship of N rate and tomato fruit yield. Although most research supports the current UF/IFAS recommended N rate of 200 lb/acre (this N rate is the BMP rate), some growers have not adopted this rate. Irrigation management has been clearly shown to play a role in NUE. Part of the reason growers do not adopt the recommended rate may be related to a lack of field demonstration of how N rate and irrigation management work together to provide maximum yields without leaching of fertilizer. Most N rate studies have not included a water quality monitoring component. In studies where this aspect was included, it was demonstrated that rates of N greater than the recommended rate led to more N leaching or more N remaining in the soil, potentially available for leaching. There is a need for more research into the leaching potential from various N and irrigation management strategies that could be used to refine the BMPs. In addition, potential loads of leached N should be measured. New, easy-to-install drainage lysimeters have been used with success in work with vegetables (Gazula et al. 2006). More work on N mass balance is needed for tomato to quantify the imports, exports, and recycling pools of N on a tomato farm.
Phosphorus and Potassium Soil Testing
Knowledge of soil nutrient levels, particularly P and K, before planting is the starting point to predicting tomato response to varying rates of applied nutrient. Using soil testing to determine preplant soil nutrient concentrations provides information so research results may be reviewed to offer some support for existing fertilization recommendations. The Mehlich-1 (double-acid) solution is the current extractant used by Florida and several other southeastern U.S. states for sandy soils.
Mehlich-1 extractant indices (expressed as ppm soil-extracted nutrient) are classified as very low, low, medium, high, and very high, and a crop specific fertilizer recommendation is made from that classification (Hochmuth and Hanlon 1995a; 2000). The M-1 solution became the accepted extractant standard in 1979 at the University of Florida. Previous to M-1, ammonium acetate and water extractants were used. Indices recorded from these methods cannot be directly equated with M-1 indices or fertilizer recommendation rates, but the review of research results from studies with these extractants presents a profile of tomato response to fertilizer in varying conditions. Water management practices, fertilizer sources, application methods, and the effect of mulch in the nutrient management system should also be considered in P and K fertilization programs.
Phosphorus
Tomato responses to rates of P in Florida are presented in Figure 5. Soil pH of Immokalee fine sand was 4.7, and hydrated lime was applied in October 1961 at rates of 500, 2000, and 5000 lb/acre (Hortenstine and Stall 1962). Subplots measuring 40 ft x 14 ft with 78 subplots/acre were treated with 100, 300, and 910 lb/acre P2O5 in November, and N, K2O, and Mg were applied through the growing season at respective rates of 220, 250, and 80 lb/acre. Irrigation method, mulch use, or research location were not specified.
Calcium present in hydrated lime increased individual tomato fruit weight, while P increased vegetation, flowering, and fruit set. Soils with high lime treatments and low P (100 lb/acre P2O5) resulted in plants with P deficiency symptoms. The reverse application of low lime (500 lb/acre) and high P2O5 (910 lb/acre) resulted in nutrient toxicity symptoms expressed as leaf roll. Higher P rates also simultaneously resulted in lower soil pH, reduced nitrification by microorganisms, and immobilization of Ca in the soil. Adequate rates of lime and P for this soil were 5,000 lb/acre lime and 300 lb/acre P2O5.
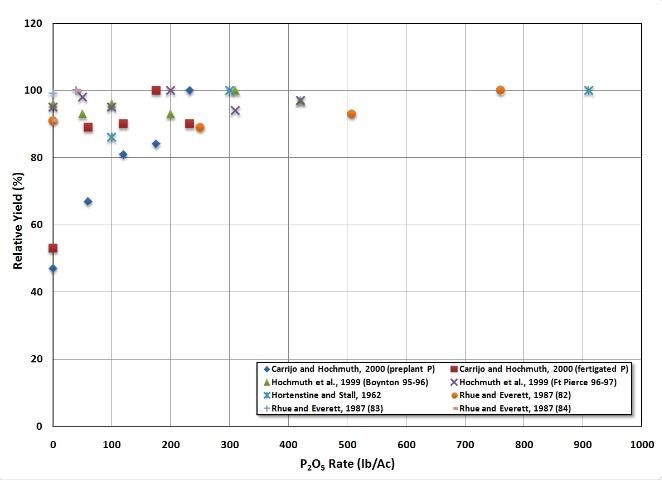
These combined rates brought soil pH to near 6.5, the recommended pH value for tomato, and soil samples taken in December and April had pH values of 6.2 and 6.3, respectively. Marketable yield with 300 lb/acre P2O5 was 88% RY (360 cartons/acre, based on 115 lb/subplot and 78 subplots/acre, bed spacing not indicated). An additional 600 lb/acre of P2O5 increased the RY to 100%, but was not cost effective.
Tomato response to added P on acidic, poorly drained Immokalee sand was studied for three winter seasons near Immokalee (Rhue and Everett 1987). Lime and P were needed to raise the pH from 5.0 to 6.5 and supplement the very low (9 ppm) M-1 soil-extracted P. Typical beds were arranged six feet on center, mulched with black polyethylene, and subsurface irrigated with the water table kept 16 inches below the bed surface. Dolomitic lime was applied annually in November at 0, 2000, and 4000 lb/acre (0, 1, and 2 times the amount required to reach 6.5 pH). Concentrated superphosphate (CSP) was broadcast and incorporated in January 1982 at 0, 250, 500, and 750 lb/acre P2O5 (150 lb/acre was recommended for this site) with starter applications of N and K2O of 25 and 40 lb/acre, respectively. Double bands of 180 lb/acre N and 250 lb/acre K2O were applied last to the bed shoulders. The same N and K fertilizer treatments were used in 1983 and 1984 except P application was reduced to 0 or 40 lb/acre P2O5 (CSP) broadcast.
Tomato yields on unlimed soils were not different with 0 or 250 lb/acre applied P2O5 but declined 35% with 500 lb/acre P2O5 (1,600 cartons/acre). Two probabilities were cited for reduced yields; the first was nutrient toxicity with excess P, and the second reason was related to decreasing leaf Mg concentrations with increases in P rate on unlimed soils. Limed soil had significantly more extractable P, but soils did not retain increased P concentrations into the next growing season. During the first season, 0, 250, 500, and 750 lb/acre P2O5 had been incorporated preplant, and the M-1 soil-extracted P measured at harvest was high. Phosphorus concentrations were low in samples taken before fertilization in 1983 and became very low in 1984. Leaching of P was likely on these very sandy, unlimed soils, as well as on sandy limed soils with successive annual P applications. Phosphorus in these conditions should be managed as a mobile nutrient, and researchers recommended seasonal P applications not to exceed crop uptake to prevent leaching losses. High yield occurred in the first trial year (1982) with 2,000 lb/acre lime and with 250 lb/acre P2O5, 100% RY (3,070 cartons/acre). An additional 40 lb/acre P2O5 applied in 1983 and 1984 increased yields 10% (1,830 and 2,000 cartons/acre respectively) compared to yields with 0 lb/acre P2O5.
Repeated annual applications of P on neutral pH EauGallie fine sand at the Gulf Coast Research Center in Bradenton increased soil P concentrations (Clark et al. 1989). Soil pH remained at or near 7.0 in this three-season study and represented typical production soils in west-central and southwest Florida. Concentrated superphosphate with micronutrients was broadcast uniformly at 375 lb/acre P2O5 each season in fall 1987, spring 1988, and fall 1988. Successive tomato crops failed to deplete soil P concentrations, rather soil P concentrations (M-1 extracted at preplant) increased from low to high concentrations during the three seasons. Yield responses to increased soil P concentrations were not evaluated. Previously cropped soil with near neutral pH often retains sufficient P so that P fertilization is not needed. UF/IFAS recommends withholding P fertilizer when soils test high for P. Application of 120 lb/acre P2O5 is recommended on soils that test low for P, and application of 100 lb/acre P2O5 is recommended for medium P soils.
Authors of research conducted in Boynton Beach during fall/winter 1994-1995 concluded that excess applied P did not enhance tomato yields (Shuler and Hochmuth 1995). Mehlich-1 soil indices of 222 ppm P for the soil at this site exceeded the 60 ppm needed for a very high soil P interpretation. Response to P was not predicted for this site. P was applied at 0, 50, 100, 150 lb/acre P2O5, and the grower rate of 200 lb/acre P2O5 to test yield response to P fertilization of a high-P soil. Phosphorus treatments combined with micronutrients, 39 lb/acre N, and 50 lb/acre K2O were broadcast in a 12–18 inch band and incorporated at bed formation. Grower N and K2O rates were applied in double-bands at 306 and 600 lb/acre, respectively. Single beds measuring 5 feet on center and 24 feet in length were mulched with black polyethylene, planted with 'Solimar' transplants, and subsurface irrigated.
Total marketable yield was not affected by added P through 200 lb/acre P2O5. The yield average using all P rates was 2,600 cartons/acre. High temperatures and tropical storm 'Gordon' claimed 10% of the plants in the first four weeks followed by satisfactory growing conditions for the remainder of the season. Phosphorus concentrations from whole leaf tissue samples were high at early fruit set and adequate through harvest with all P rates. Soil P concentrations had increased through 444 ppm with P fertilization to 200 lb/acre P2O5 at the early soil sampling. Soil P concentration remained unchanged from the prefertilization value through the season with the zero P treatment. Soil Zn concentrations decreased 40% with added P.
Further testing of tomato yield response in the presence of high soil P occurred on Millhopper fine sands near Gainesville (Locascio et al. 1996). Soil at this spring 1996 trial site tested very high (92 ppm) in M-1 soil-extracted P, and additional P was not recommended. Beds two-foot wide and six feet on center received broadcast applications of N, P (CSP), and K, which were incorporated, mulched in black polyethylene, and drip-irrigated to maintain soil moisture at -10 centibars by tensiometer. Phosphorus was applied at 0 or 160 lb/acre P2O5. Soil P concentrations were higher (1% level) through June 17 where P had been applied. Although marketable fruit yields were not affected by higher soil P concentrations, plant growth at the May 17 whole-plant sampling date had accelerated where P was applied. Larger plants at this sampling contained 13% more P (significant at 1%) in whole plant analysis than plants grown with zero added P.
Graywall is a fruit disorder of tomato fruit in the winter in Homestead. Trials were conducted in 1995-1997 on commercial farms to test the effects of reduced phosphorus fertilizer on tomato yield and graywall (Lamberts et al. 1997). Reduced P treatments were 37%, 50%, 63% reductions in P compared to the grower treatment (100%, which ranged from 190 to 230 lb/acre P2O5). In the first season, tomatoes were harvested at the pink stage five times with Grower 'A' and two times (mature-green stage) with Grower 'B.' In the second year, fruits were harvested twice at the mature green stage with Grower 'C.' Neither of the reduced P treatments had any impact on fruit yield or graywall incidence.
Two experiments were conducted in southeastern Florida on commercial tomato farms to test tomato response to P fertilization on soils testing high in Mehlich-1 soil P (Hochmuth et al. 1999). One study was conducted in 1995-1996 in Boynton Beach, Florida (M-1 P was 290 ppm), and the second study was conducted in Ft. Pierce (M-1 P was 63 ppm) in 1996-1997. Neither total marketable nor total seasonal production was affected by P fertilization throughout the range of 0–460 lb/acre P2O5. Cull (misshapen) fruits increased with P fertilization in the second season.
Further studies were conducted in Gainesville, Florida, on soils variable in Mehlich-1 P (Carrijo and Hochmuth 2000). Studies investigated drip-irrigated tomato responses to P rate and P application method (preplant application or fertigation). On a low-P soil (9 ppm M-1 P) fertigation was more efficient than pre-plant application. On this low-P soil, tomato yield response was maximized with 140 lb/acre P2O5. On a soil testing high in P (48 ppm M-1 P), yield was maximized with 50lb/acre P2O5. On a soil testing very high in P, tomato yield was maximized with 0 lb/acre P fertilizer. These responses to P substantiated the fertilizer predictions made from the Mehlich-1 soil test.
Stanley et al. (1995) investigated P levels in and around 10 vegetable fields in southwestern Florida in 1990-1991. Some movement of P from fields appeared likely but ortho-P levels were elevated in natural sites that had not received P fertilizer. Naturally occurring P deposits appeared to be contributing P to the ground waters. Regulatory agencies were advised to consider the natural sources of P in making regulatory proposals. Retention ponds excavated into the P-containing soils may end up as a P source.
In a study conducted by Sato et al. (2009b), P was applied to soils that met the Mehlich-1 pre-planting requirements for no P addition. Despite adding P contrary to the UF/IFAS recommendation (31–60 ppm in the high category and > 60 ppm as very high), they found that the majority of the soil labile P remained in the plant root zone. Despite this observation, the authors suggested that the risk for P leaching in the future should not be ruled out, and they recommended sticking to the UF/IFAS recommendations for P fertilization.
Soil tests showed very high Mehlich-1 P concentrations in several fields in the C-139 Basin area in southern Florida (Morgan et al. 2009). No fertilization with P was recommended based upon soil testing. In three years (spring 2005 to spring 2008), tomato plant biomass was not affected by P fertilization. The authors suggested that reduced P fertilization may have led to delayed fruit maturity because on one farm out of two, the yield of large fruits increased in the third of three harvests in the first year. However, fruit yields in the first and second picks were not affected by reduced P. On a second farm, there was no effect of reduced P on fruit yield. In the second year, one farm had no effect of reduced P on tomato yield. At a second farm, tomato early and late yields responded to P additions. At a third farm, yields were not affected by P reductions. Researchers theorized that withholding P led to a delay in maturity because of the increased large-size fruits with P additions.
Hochmuth et al. (1993) analyzed data from several studies with several crops, including tomato. These researchers concluded that the Mehlich-1 soil test extractant appeared to be correlated with yield and native soil P level. These studies were all conducted on soil with relatively neutral to slightly acidic reaction, unlike the studies reported above by Morgan et al. (2009). The M-1 extractant, which is a combination of dilute acids, may not be appropriate for soils with high pH because high concentrations of carbonates may neutralize the acids in the extractant.
Phosphorus Summary
Successive seasonal applications of P at rates exceeding M-1-based recommendations accumulate in most soils but provide no yield advantage. In the presence of high soil P, mid-season plant growth was accelerated compared to plants grown on high P soils without added P. Plants grown with high P fertilization also contained 13% more P by whole plant analysis but did not yield more fruit. On acidic soils, P did not accumulate and P leaching occurred. Application of P should not exceed crop uptake on coarse sandy soils to prevent possible interim leaching losses between crop seasons. Phosphorus application on acidic, unlimed soils caused nutrient toxicity symptoms in tomato as well as restricting Ca, Mg, and N uptake from the soil solution. On limed soils very low in M-1 P up to 250 lb/acre P2O5 was needed for maximum yield. More research is needed to better relate required P fertilization with the M-1 soil test index. P fertilization by M-1 extractant was shown to be accurate for slightly acidic sandy soils, and the amounts of P needed were accurately predicted. Soils testing high or very high in M-1 P do not need to be fertilized with P. Fertigation of P was an efficient method of applying P as long as considerations were made to prevent P precipitation in the drip lines.
Potassium
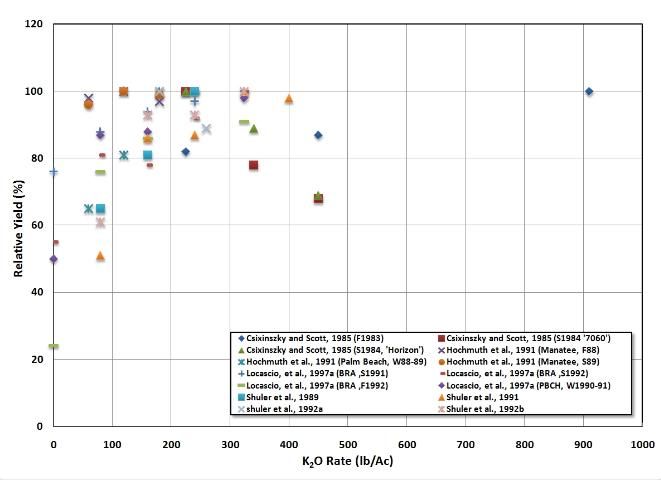
Potassium is a required element for optimum tomato production and has been implicated in various fruit quality aspects. Growers apply considerable amounts of K to tomato crops. When data on grower application rates were presented, the amounts were often given as more than 400 lb/acre K2O. Tomato responses to K fertilization are presented in Figure 6. A fall/winter 1988-1989 replicated trial conducted in Boynton Beach evaluated response of tomato to four K2O rates of 80, 160, 240, and 440 lb/acre and the grower rate of 672 lb/acre K2O (Shuler et al. 1989). Fertilizers were derived from KNO3, Ca(NO3)2, and NH4NO3. Soil tested very low (16 ppm) in extracted K using M-1 extractant, and the recommended rate for very low-K soils in 1989 was 160 lb/acre K2O (Hochmuth and Hanlon 1989; Kidder et al. 1989). A starter fertilizer with 60 lb/acre K2O was broadcast, and the remaining K was double banded to equal the total rates listed above. Single beds were 5.25 ft x 23.8 ft and contained 12–13 plants. Subsurface water levels were monitored with a water table recorder.
Total marketable yield increased linearly with increases in K2O through 240 lb/acre, 100% RY (2,160 cartons/acre). Yield declined 24% with grower N and K2O rates of 336 and 672 lb/acre. Leaf K concentrations at first flower and early fruit set were adequate with 240 lb/acre K2O but were deficient at first flower with 160 lb/acre K2O. Leaves with all experimental K rates were considered K deficient at first harvest with leaf K concentrations less than 2.0% (Hochmuth et al. 1991b). Soil had returned to very low M-1 potassium levels after harvest, and researchers noted that removal of mulch and plant material allowed residual soil K to leach. Band fertilization with 672 lb/acre K2O resulted in residual K (76 ppm) in the band at the end of the season. The yield response of subsurface-irrigated tomato to higher K rates in this study and others (Locascio et al. 1997a) led to a K rate recommendation revision for very low-K soils from the 1989 standard recommendation of 160 lb/acre K2O (Kidder et al. 1989) to 225 lb/acre K2O (Hochmuth and Hanlon 1995a).
Starter K2O was applied to commercial fields at rates of 40, 60, and 80 lb/acre in Manatee (fall), Palm Beach (winter), and Manatee (spring), respectively (Hochmuth et al. 1991a). Subsurface irrigation was managed by the grower on polyethylene-mulched fields. Experimental rates of K2O were applied in shoulder bands at varying rates depending on the specific experiment. Fertilizer sources were as follows: KNO3,Ca(NO3)2, NH4NO3, and K2SO4. 'Sunny' transplants were used for all trials.
Tomato yields were the same with all experimental K rates during the warm fall (1988) and spring (1989) seasons in Manatee County. High RYs were 98%, 100%, and 97% with 80, 160, and 240 lb/acre K2O (1988) and 96%, 100%, and 99% with 120, 200, and 280 lb/acre K2O (1989). High yields were 748 and 1,326 cartons/acre (13-foot row spacing, K rates calculated on 6-foot bed centers) with 160 and 200 lb/acre K2O applied each year. Acceptable yields occurred with the 1989 recommendations for very low and low-K soils, 160 lb/acre K2O (fall 1988) and 130 lb/acre K2O (spring 1989), respectively. Satisfactory leaf K concentrations, above the leaf sufficiency range, occurred with all K rates during these seasons. In the cooler winter season in Palm Beach County (1988-1989), tomato yields responded linearly to added K through the 240 lb/acre K2O rate, 100% RY (2,160 cartons/acre). Leaf K concentrations were deficient for lesser K rates, 80 and 160 lb/acre K2O, during this winter sampling. The 1989 recommendation of 160 lb/acre K2O for very low-K soils (16 ppm) was insufficient. Winter yields requiring 240 lb/acre K2O responded near the revised recommendation (Hochmuth and Hanlon 1995a) of 225 lb/acre K2O. Higher grower K rates used for all three seasons, 530, 625, and 393 lb/acre K2O, resulted in yields 15% lower, equal to, and slightly greater than yields with 240 lb/acre K2O for fall, winter, and spring trials, respectively.
A fall/winter 1991 experiment in Boynton Beach evaluated marketable yield response to three reduced N rates, four reduced K rates, and the grower rates of 652 lb/acre K2O and 346 lb/acre N (Shuler et al. 1992). Mehlich-1 soil-extracted K was low, 20 ppm, and 130 lb/acre K2O was recommended based on 1989 standards (Kidder et al. 1989). All treatments consisted of 40-200-40 lb/acre N-P2O5-K2O preplant broadcast fertilizer. The remaining K2O was double banded for total K2O rates of 80, 160, 240, and 320 lb/acre. Beds were spaced 5 feet on center with fertilizer calculations based on this spacing. Beds were mulched with white-on-black polyethylene, and 'Sunny' tomatoes were harvested three times.
The 100% RY value (1,770 cartons/acre) occurred with 240 lb/acre K2O with a 94% RY with 160 lb/acre K2O, in a quadratic response to applied K. This yield response exceeded the 130 lb/acre K2O 1989 recommendation and supported the higher 1995 recommendation of 150 lb/acre K2O for low-K soils. Yields did not increase with 320 lb/acre K2O, nor were yields improved with the commercial rate of 652 lb/acre K2O (1,907 cartons/acre). Extra-large fruit yields increased 45% with 160 lb/acre K2O compared to the 80 lb/acre K2O rate and leveled off thereafter. Adequate leaf K concentrations did not increase significantly with K rate at first flower, but increased linearly at early fruit set. Soil samples taken through the fertilizer band after harvest resulted in high concentrations of M-1 soil-extracted K, 490 ppm from the 612 lb/acre banded K2O and 221, 93, 37, and 19 ppm from the reduced K rates 280, 200, 120, and 40 lb/acre banded K2O, respectively. Increasing K rates from 80 to 320 lb/acre K2O did not significantly affect incidence of graywall and blotchy ripening (BR).
A winter 1991-1992 trial in Boca Raton tested reduced rates of soluble and controlled-release (CR) K sources against a commercial fertilizer treatment (Shuler 1992). Mehlich-1 soil testing revealed very low levels of soil K (4 ppm), and 160 lb/acre K2O was recommended in 1989. All treatments consisted of 40-20-60 lb/acre N, P2O5, K2O broadcast preplant with remaining treatments double banded for total K2O rates of 180 and 260 lb/acre (160 lb/acre N). The grower applied 300 lb/acre K2O, using broadcast and band placement, with 200 lb/acre N. Single-bed plots were 5.5 x 21.8 feet with eight 'Mountain Pride' tomato plants per bed. Beds were covered with silver-topped polyethylene mulch.
Following five harvests, marketable yield was not different with any K rate or K source. Yield response with soluble K sources was slightly higher with 180 lb/acre K2O, 100% RY (1,745 cartons/acre) and lower with 260 lb/acre K2O, 89% RY. When half of the band-applied K2O was from a controlled-release source, equally high yields (1,828 cartons/acre) were produced with 180 lb/acre K2O. Large fruit accounted for 48% of this yield while other treatments resulted in 43% large fruit (Shuler 1992). Tomato yield with the grower rate was 1,870 cartons/acre. Leaf-tissue K concentration was adequate with all treatments sampled at mature green fruit stage. Tissue K concentration was the same for plants receiving all soluble-K fertilizer and plants receiving half-soluble and half-CR forms of K. Soil samples taken through the fertilizer band after harvest had 409 ppm K with the commercial application of 300 lb/acre K2O (200 lb/acre banded). Lesser banded amounts of 120 and 160 lb/acre soluble K2O returned 55 and 89 ppm M-1 K in the fertilizer band.
Three Bradenton trials, including two in the spring and one in the fall, and one West Palm Beach winter trial evaluated the effects of K source and rate on subsurface-irrigated, polyethylene-mulched tomato (Locascio and Hochmuth 1994; Locascio et al. 1997a). Neither the 1989 recommendation of 130 lb/acre K2O nor the 1995 recommendation of 150 lb/acre K2O optimized yields for M-1 low-K (25 and 26 ppm) soils in Bradenton spring trials held in 1991 and 1992. Yields increased linearly in spring 1991 and increased quadratically in 1992, leveling off at more than 240 lb/acre K2O, 93% RY (2,535 cartons/acre). One hundred percent RY occurred at both trials with 325 lb/acre K2O. Researchers cited the inefficiency of banded-K fertilizers when post-harvest M-1 soil tests revealed large amounts of residual K2O in the band (all of the N and K2O were applied in two bands on the bed shoulder). Despite the higher yield demand for K, tomato leaf K concentrations measured in leaves taken at mid-season (spring 1992) were adequate with even the lowest K rate of 80 lb/acre K2O. In research with pepper (Hochmuth et al. 1994b), band placement was also found to be the least effective placement method with subsurface irrigation.
Plants grown in a M-1 very low K (15 ppm) soil in Bradenton in fall 1992, produced 100% RY (3,200 cartons/acre) with 240 lb/acre K2O. The maximum yield exceeded the 160 lb/acre K2O rate recommended in 1989 and was closer to the 225 lb/acre K2O rate recommended in 1995. Yields increased quadratically through 0, 80, and 160 lb/acre K2O, maximizing with 240 lb/acre K2O, and declining to 93% RY with 325 lb/acre K2O. Band placement of K2O was used in this trial. Mid-season leaf concentrations were adequate with all K rates, increasing linearly with K2O treatments from 80 to 320 lb/acre. Yields varied less than 3% with K sources, KCl, K2SO4, or KNO3 at two of the three Bradenton trials, but marketable fruit yield increased 19% with KNO3 in the spring of 1992.
In West Palm Beach, during winter 1990-1991, 80 lb/acre K2O was broadcast preplant and the remainder was banded on the bed shoulders for total K2O rates of 80, 160, 240, 320, and 400 lb/acre (Locascio et al. 1997a; Shuler et al. 1991). The bed spacing was not indicated in this experiment. Yields responded quadratically to increasing K rates leveling off with 320 lb/acre K2O, 100% RY (1,850 cartons/acre). Yields optimized above the 1989 recommended rate of 160 lb/acre K2O for this very low (12 ppm) K soil and above the 1995 recommended rate of 225 lb/acre K2O. Leaf K concentrations at mid-season were adequate with rates exceeding 240 lb/acre K2O, but deficient with 80 and 160 lb/acre. A dry period between October 15 through December 20 (2.0 inches of rain) was cited for the inefficient utilization of banded-K fertilizer, which resulted in the higher-than-expected fertilizer demand. Significant amounts of K remained in the fertilizer band after harvest, ranging from 34 to 674 ppm with treatments of 80–502 lb/acre K2O (post-harvest M-1 soil samples).
Graywall, a fruit disorder, has been considered to be related to K deficiency. However, studies in southern Florida to test this theory showed that increased N rate was more related to increased graywall than K fertilization (Carranza et al. 1996; 1997). Graywall and BR incidence decreased with higher K2O rates from 52 to 20 cartons/acre as K2O increased from 180 to 260 lb/acre K2O, consistent with the theory that these disorders are related to K deficiency. When K was reduced to 180 lb/acre K2O but half in CR form, fewer cartons of graywall and BR fruit were harvested, 17 cartons/acre (Shuler 1992). Reduced graywall and BR with CR fertilizer was probably due to more consistent availability of K. Similar leaf N and K concentrations indicated factors in addition to K were involved in these disorders.
Summary of Subsurface Irrigation and K Rates
In most of the subsurface-irrigated experiments, yield was maximized at or less than 240 lb/acre K2O. In some studies, responses to higher K rates were observed. Shoulder band applications of N and K2O were used in all experiments, and yields responded similarly to 0 through 80 lb/acre K2O broadcast preplant. Poor absorption of band-applied K by tomato was apparent in some instances when leaf K concentrations were deficient despite high amounts of residual K extracted through the fertilizer band post harvest. Low absorption of band-applied K was exaggerated by dry weather and fluctuations in soil water conditions. Higher grower K2O rates up to 672 lb/acre caused yields to drop 15%–25% or showed no increase compared to lower fertilization rates. Equivalent yields resulted with all K sources including KCl, K2SO4, KNO3, soluble sources, or half-soluble: half-CR sources. Graywall incidence was not affected with K2O increases up to 320 lb/acre.
Drip Irrigation
Responses of tomato to K fertilization with drip irrigation are presented in Figure 7. In studies in Quincy, Gainesville, and Live Oak, the effects of K rate and source on drip-irrigated 'Sunny' tomato were evaluated in spring seasons 1986, 1990, and 1991 (Locascio and Hochmuth 1994; Locascio et al. 1997a). Micronutrient mix was broadcast preplant at 40 lb/acre in 1986 with all of the K fertilizer (either 215 or 430 lb/acre K2O). Soil beds at all sites were mulched with black polyethylene (bed spacing was not indicated).
Medium M-1 K concentrations of 60 and 50 ppm were reported in the spring 1986 at Gainesville and Quincy, respectively, and 100 lb/acre K2O was recommended. Yield responses to 215 and 430 lb/acre K2O were similar at both sites. Relative yields with 215 lb/acre K2O were 99% (2,970 cartons/acre) at Quincy and 95% (2,070 cartons/acre) at Gainesville. Yields were also similar with both KCl and K2SO4 potassium sources. Leaf K concentration was adequate in the Gainesville study; data were not presented for Quincy.
Two additional Quincy studies were conducted in spring 1990 and 1991 where the soil M-1 potassium indices were medium (37 ppm) and low (34 ppm). All of the K fertilizer was broadcast preplant. Yield in 1990 was maximized within the medium recommendation of 100 lb/acre K2O with non-significant yield increases in excess of 80 lb/acre K2O (97% RY) (2,590 cartons/acre). Likewise in 1991, yield peaked with 160 lb/acre K2O, 100% RY (1,660 cartons/acre), near the recommended rate of 150 lb/acre K2O for low M-1 K soils. With K rates of 240 and 325 lb/acre K2O, yields declined 8% and 12%, respectively. There were no significant differences in yield responses to K supplied from KNO3, KCl, or K2SO4 at either location.
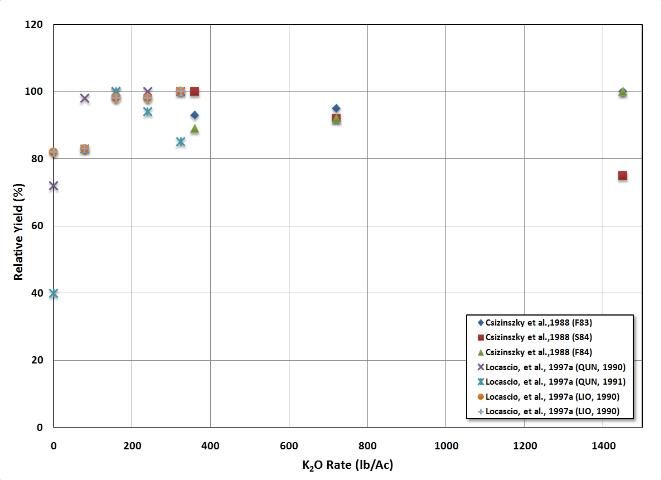
A fifth study at Live Oak in spring 1990 was conducted on a soil testing 54 ppm for K and 100 lb/acre K2O was recommended (Locascio and Hochmuth 1994; Locascio et al. 1997a). Yield increased through 160 lb/acre K2O (2,175 cartons/acre, 97% RY) and was unaffected by additional K2O at 240 or 325 lb/acre. The recommended K rate of 160 lb/acre at this site would have been insufficient for maximum tomato production. Yield response was not affected by K sources KNO3, KCl, or K2SO4.
Research in Dade County was aimed at determining N and K fertigation rates for the Rockdale soils and evaluating N and K effects on graywall incidence (Carranza et al. 1996). Growers often associate graywall and other ripening disorders with low-K fertilization. Graywall is a particular concern in commercial fields because graywall commonly causes yield losses of 10%–20% and occasionally losses greater than 50%. Graywall and BR incidence decreased with higher K2O rates from 52 to 20 cartons/acre as K2O increased from 180 to 260 lb/acre K2O, consistent with the theory that these disorders are related to K deficiency. When K was reduced to 180 lb/acre K2O but half in CR form, fewer cartons of graywall and BR fruit were harvested, 17 cartons/acre (Shuler 1992). Reduced graywall and BR with CR fertilizer was probably due to more consistent availability of K. Similar leaf N and K concentrations indicated factors in addition to K were involved in these disorders.
A factorial experiment was used with four N rates and four K rates from NH4NO3 and KCl in a fall/winter 1995-1996 season (Carranza et al. 1996). Twenty percent of the fertilizer was applied and incorporated preplant, and the remainder was injected through a drip irrigation line. Bed spacing was not indicated. Fertilizer injection graduated from 7 lb/acre/week N or K2O through 14 lbs/week after the fourth week in the season. Tensiometers were used to monitor soil moisture.
Soil samples sent to the UF/IFAS Analytical Research Laboratory (ARL) resulted in medium- to high-K interpretations using the AB-DTPA soil analysis method (Soltanpour and Schwab 1977). No yield response to applied K was anticipated and subsequent yield data confirmed no response to increased K (Carranza et al. 1996). The largest yield occurred with 50 lb/acre K2O, 100% RY (1,743 cartons/acre), and the yield was 83% RY with zero lb/acre K2O. Leaf tissue analysis results were not reported. Leaf-petiole sap K+concentrations averaged 3,300 ppm, just less than the 3,500–4,000 ppm recommended concentration (Hochmuth 1994) at first flower. Potassium concentrations were adequate for the remaining three sample stages but did not increase appreciably with K rates greater than the 50 lb/acre K2O rate throughout the sampling periods. Low-K fertilization was not a factor in graywall incidence on this medium- to high-K soil. Additional K2O at rates in excess of 50 lb/acre had no effect on total marketable yield or graywall incidence.
Tomato responses to K fertilization in all K studies with all irrigation methods are summarized in Figure 8. Most studies led to maximum yields with 200–250 lb/acre K2O.
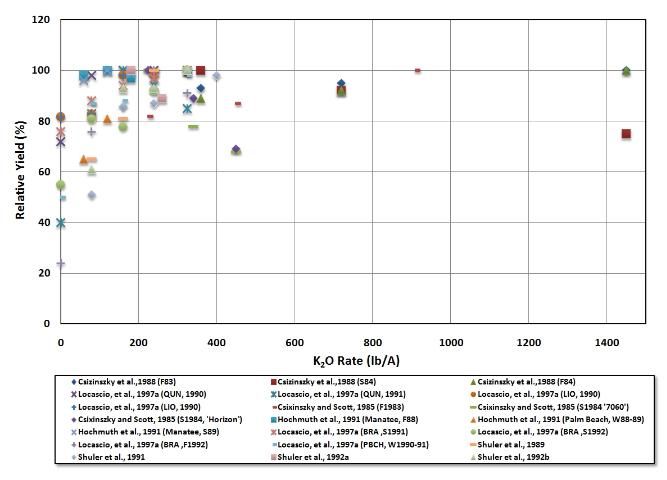
Potassium Summary
Responses to K rate were variable in relation to the M-1 predicted fertilizer amount. The M-1 recommended K rate for drip-irrigated tomato was supported by high yields in some studies. In a few studies yields maximized with more than the recommended rate. Increases in K greater than the recommended rate did not generally increase yields; in one season, yields fell 12% with 325 lb/acre K2O. Potassium absorption as measured by petiole sap testing indicated K concentrations did not increase with excess K application. Higher than recommended rates of K were not utilized by tomato for optimum growth or yield, especially with banded K in seepage-irrigated tomato.
Potassium rate recommendations from 1989 consistently failed to optimize yields in 15 of the 18 trials reported above. Tomato yields responded near the 1995 recommended K rate, based on M-1 soil test indices, in 70% of the trials documented above. Four subsurface-irrigated trials and one drip-irrigated trial accounted for the remaining 30% of trials that required greater K amounts for optimum yields. Winter conditions likely reduced absorption of applied K in two of the subsurface trials, but the large amounts of residual K found in the band post-harvest were of greater significance. Band application of K fertilizer has proven the least efficient K application method with other vegetables. Based on results from these K trials, the recommended maximum K rate was increased in 1995 from 160 to 225 lb/acre K2O. Revised recommendations were from 160 to 225 lb/acre K2O and from 130 to 150 lb/acre K2O, for respective very low and low M-1 tested K soils (Kidder 1989; Hochmuth and Hanlon 1995), based on some of the above summarized work. An additional factor was that the Mehlich-1 soil test failed to accurately predict K fertilizer needs. This aspect of the M-1 was shown for potato and other crops (Hochmuth et al. 1993). Potassium sources KCl, K2SO4, and KNO3 had no effect on yields of drip or subsurface-irrigated tomatoes in all trials except one where tomato yields increased 19% with the KNO3 treatment.
Summary for N, P, and K Fertilization of Tomato
Tomato is an important crop to the overall vegetable industry in Florida. During the 2007-2008 season, 31,500 acres were harvested with a value of $622 million (VanSickle et al. 2009a, b). Fertilizer is an important part of the tomato pre-harvest production budget inputs and amounts to $1,450 per acre (VanSickle et al. 2009a, b). This cost makes fertilizer account for about 9% of the total $16,250 per acre total production, harvesting, and marketing costs.
Mehlich-1 soil tests often result in low, medium, high, and very high indices of residual soil P and K; and UF/IFAS recommendations are further adjusted downward based on nutrient concentrations present in the soil. Assuming soils tested statewide were very low in P and K, fertilizers applied in 1994 were 25% and 60% greater than the recommended rate for these nutrients and 45% more than the recommendation for N fertilizer. Lower rates of applied fertilizer would reduce fertilizer costs without sacrificing yields. When no additional P and K are required on soils high and very high in these nutrients, fertilizer costs and environmental impact could be reduced even further.
Determining the most yield-responsive fertilizer rate for tomato, improving fertilizer-plant efficiency, and reducing nutrient leaching losses have dominated research. Use of polyethylene mulched beds, coated fertilizers (polymer, resin, and sulfur-coating), split fertilizer applications, and restricted water application have focused on fertilizer application timed to plant needs without the N losses associated with excessive water and fertilizer application. In these studies, most tomato crops responded to applied fertilizer N up to 200 lb/acre N, with slightly less nutrient required for tomatoes grown on loamy soils compared to sandy soils.
The objective of increasing fertilizer efficiency was found in these studies to be partially accomplished by avoiding inefficient fertilizer placement methods, particularly band placement of K fertilizer when using subsurface irrigation. Other means of increasing fertilizer efficiency include following calibrated soil test recommendations, managing irrigation in an optimal manner, and applying some of the N and K through the drip irrigation system.
Irrigation system and nutrient management were shown to be linked. Some studies with seepage (subsurface irrigation) showed responses to more N than the recommended rate. This number was a small minority of the total number of studies across irrigation systems and locations in the state. For some of the responsive studies, no explanation was provided for the possible response. In others, data were collected that revealed significant residual fertilizer in the fertilizer bands after the final tomato harvest.
Additional research is needed to improve the efficiency of the fertilizer-soil-plant system. Although drip irrigation has reduced water use by one-third to one-half of the water used by subsurface and overhead-irrigated fields, N and K remain of greatest concern as potentially leached fertilizer elements, even with this low water-use irrigation method. Research is needed with CRN and K sources, where a preplant-only fertilizer application could reduce N leaching losses, reduce management costs associated with split fertilizer application and fertigation, and provide a sustained nutrient source. As new CR fertilizer sources are developed, experimentation will be needed to determine their effectiveness with specific crops. Improvement remains possible with fertilizer sources that adjust to seasonal growing conditions — that is, spring, fall, and winter crop periods where temperatures, soil microbial activity, and plant growth rate change. Where soil N retention is poor, increasing organic matter content with cover cropping, for example, is known to increase N mineralization and decrease the need for applied fertilizer N. Studies also are needed on optimal timing of N application with drip irrigation systems and on the fate of N in the soil system as it relates to N and irrigation management.
Large-scale demonstrations on commercial farms of recommended nutrient and irrigation management programs are also needed. This requirement is particularly true for the seepage irrigation system. The question here is "Why can some farms grow tomato successfully with the recommended rate of N, while others cannot?" For subsurface irrigation management to remain relevant, research should be focused on determining the underlying differences in nutrient and water management relationships with seepage irrigation. Otherwise, micro-irrigation should be actively pursued, possibly with some form of cost-sharing and with proper field training of personnel.
Literature Cited
Andersen, P.C., F.M. Rhoads, S.M. Olson, and B.V. Brodbeck. 1999. "Relationships of Nitrogenous Compounds in Petiole Sap of Tomato to Nitrogen Fertilization and the Value of These Compounds as a Predictor of Yield." Hort. Science 254:254-8.
Black, C.A. 1992. Soil Fertility Evaluation and Control. Boca Raton, FL; Lewis Publishers.
Brown, J.R. 1987 Soil Testing: Sampling, Correlation, Calibration, and Interpretation. Soil Science Society of Amer. Special Publ. No. 21. Madison, Wisc.: Soil Science Society of America, Inc.
Carranza, J.F., S.K. O'Hair, H. Bryan, G. Hochmuth, and E.A. Hanlon. 1996. "Nitrogen and Potassium Fertigation and Cultivar Effects on Tomato Yield and Graywall in Dade County." Proc. Fla. Tomato Inst. Univ. Fla. Coop. Ext. Circ. Pro 108:35-9.
Carranza, J.F., S.K. O'Hair, H. Bryan, G. Hochmuth, and E.A. Hanlon. 1997. "Effects of Nitrogen and Potassium Fertigation on Tomato Yield and Graywall in Dade County." Proc. Fla. Tomato Inst. Univ. Fla. Coop. Ext.Circ. Pro 108: 53-5.
Carrijo, O.A., and G. Hochmuth. 2000. "Tomato Responses to Pre-plant Incorporated or Fertigated Phosphorus on Soils Varying in Mehlich-1 Extractable Phosphorus." HortScience 35:67-72.
Clark, G.A., G.J. Hochmuth, E.A. Hanlon, C.D. Stanley, D.N. Maynard, and D.Z. Haman. 1989. Water and Fertilizer Management of Micro-irrigated Tomato Production on Sandy Soils in Southwest Florida. Southwest Fla. Water Management Dist. Final Report.
Clark, G.A., G.J. Hochmuth, E.A. Hanlon, C.D. Stanley, D.N. Maynard, and D.Z. Haman. 1991. "Water and Fertilizer Management of Microirrigated Fresh Market Tomatoes." Am. Soc. of Agr. Engineers 34(2): 429-35.
Csizinszky, A.A., J.B. Jones, and C.D. Stanley. 1988. "Effect of N and K rates on Bacterial Spot in Trickle Irrigated Tomatoes ." Proc. Fla. State Hort. Soc. 101:353-6.
Csizinszky, A.A., and J.W. Scott. 1985. "Response of Tomato Breeding Line 7060 and 'Horizon' to Nitrogen and Potassium Rates." Proc. Fla. State Hort. Soc. 98:240-4.
Csizinszky, A.A., and C.D. Stanley. 1998. "Response of Tomatoes to Microirrigation Rates, Compost Placement and Rates, and N and K sources." Proc. Fla. State Hort. Soc. 111:73-7.
Csizinszky, A.A., and J.W. Scott. 2000. "Response of 'Florida 47' Tomato to Microirrigation, and N-rates and Ca-sprays." Proc. Fla. State Hort. Soc. 113:242-6.
Dangler, J.M., and S. Locascio. 1990. "Yield of Trickle-irrigated Tomatoes as Affected by Time of N and K Application." J. Amer. Soc. Hort. Sci. 115: 585-9.
Dukes, M.D., L. Zotarelli, and K.T. Morgan. 2010. "Use of Irrigation Technologies for Vegetable Crops in Florida." HortTechnology 20:133-42.
Everett, P.H. 1971. "Evaluation of Paper and Polyethylene-coated Paper Mulches and Fertilizer Rates for Tomatoes." Proc. Fla. State Hort. Soc .84: 124-8.
Everett, P.H. 1976." Effect of Nitrogen and Potassium Rates on Fruit Yield and Size of Mulch-grown Staked Tomatoes." Proc. Fla. State Hort. Soc. 89:159-62.
Everett, P.H. 1978. "Fertilizing Tomatoes or Cucumbers as Second Crops on Plastic Mulched Beds." Proc. Fla. State Hort. Soc. 91:317-9.
Fan, X.H., and Y.C. Li. 2009. "Effects of Slow Release Fertilizer on Tomato Growth and Nitrogen Leaching." Comm. In Soil Sci. and Plant Anal. 40: 3452-68.
Florida Dept. of Agriculture and Consumer Services. 2005. Water Quality/Quantity Best Management Practices for Florida Vegetable and Agronomic Crops. Accessed August 4, 2011. http://www.floridaagwaterpolicy.com/PDF/Bmps/Bmp_VeggieAgroCrops2005.pdf.
Florida Agriculture Statistical Bulletin. 2009. Season Vegetables Highlights. Accessed August 4, 2011. http://www.nass.usda.gov/Statistics_by_State/Florida/Publications/Annual_Statistical_Bulletin/fasb09p.htm.
Funderburk, J.E., P.C. Andersen, and S.M. Olson. 1997. "UV-reflective Mulch and Nitrogen Fertility Effects on Western Flower Thrips and Tomato Spotted Wilt Virus in Tomato." Fla. Agr. Expt. Sta. NFREC Research Report: 97-7.
Gazula, A., E. Simonne, M.D. Dukes, G.J. Hochmuth, R. Hochmuth, and D. Studstill. 2006. "Optimization of Drainage Lysimeters Design for Field Determination of Nutrient Loads." Proc. Fla. State Hort. Soc. 119:231-3.
Hanlon, E.A., and G.J. Hochmuth. 1989. Calculating Fertilizer Rates for Vegetable Crops Grown in Raised-bed Cultural Systems in Florida. Fla. Coop. Ext. Serv. Spec. Series SS-SOS-901. Gainesville: University of Florida Institute of Food and Agricultural Sciences.
Hanlon, E.A., and G.J. Hochmuth. 1992. "Recent Changes in Phosphorus and Potassium Fertilizer Recommendations for Tomato, Pepper, Muskmelon, Watermelon, and Snapbean in Florida." Commun. Soil Sci. Plant Anal. 23 (17-20): 2651-85.
Hartz, T.K., and G.J. Hochmuth. 1996. "Fertility Management of Drip-irrigated Vegetables." HortTechnology 6:168-72.
Hochmuth, G.J. (ed.). 1988a. Tomato Production Guide for Florida. Fla. Coop. Ext. Serv. Circ 98C. Gainesville: University of Florida Institute of Food and Agricultural Sciences.
Hochmuth, G.J. 1988b. Commercial Vegetable Fertilization Guide. Fla. Coop. Ext. Serv. Circ 225C. Gainesville: University of Florida Institute of Food and Agricultural Sciences.
Hochmuth, G.J. 1992a. "Fertilizer Management for Drip-irrigated Vegetables in Florida." HortTechnology 2 :27-32.
Hochmuth, G.J. 1992b. "Concepts and Practices for Improving Nitrogen Management for Vegetables." HortTechnology 2(1): 121-5.
Hochmuth, G.J. 1994a. Plant Petiole Sap-testing Guide for Vegetable Crops. Fla. Coop. Ext. Serv. Circ. 1144. Gainesville: University of Florida Institute of Food and Agricultural Sciences.
Hochmuth, G.J. 1994b. "Sufficiency Ranges for Nitrate-nitrogen and Potassium for Vegetable Petiole Sap Quick Tests." HortTechnology 4:218-22.
Hochmuth, G.J. 1996a. "Vegetable Fertilization" In Vegetable Production Guide for Florida, edited by G.J. Hochmuth and D. Maynard, 3-17. Fla. Coop. Ext. Serv. Circ. SP 170. Gainesville: University of Florida Institute of Food and Agricultural Sciences.
Hochmuth, G.J. 1996b. Commercial Vegetable Fertilization Guide. Fla. Coop. Ext. Serv. Circ. 225D. Gainesville: University of Florida Institute of Food and Agricultural Sciences.
Hochmuth, G.J. 1997. "Response of Mulched Tomato to Meister Controlled-release Fertilizer." Fla. Agr. Expt. Sta. Research Report, Suwannee Valley REC97-8. Gainesville: University of Florida Institute of Food and Agricultural Sciences.
Hochmuth, G.J. 1998a. "Response of Tomato to Fertilization with Meister Controlled-release Fertilizers." Research Report, Suwannee Valley REC 98-2. Gainesville: University of Florida Institute of Food and Agricultural Sciences.
Hochmuth, G.J. 1998b. "Evaluation of Pursell Controlled-release Polymer-coated Urea for Tomato and Pepper." Research Report, Suwannee Valley REC 98-7. Gainesville: University of Florida Institute of Food and Agricultural Sciences.
Hochmuth, G.J. 2000. "Management of Nutrients in Vegetable Production Systems in Florida." Soil and Crop Sci. Soc. Fla. Proc. 59:11-13.
Hochmuth, G.J., O. Carrijo, and K. Shuler. 1999. "Tomato Yield and Fruit Size Did Not Respond to P fertilization of a Sandy Soil Testing Very High in Mehlich-1 P." HortScience 34:653-6.
Hochmuth, G., and K. Cordasco. 2000 (reviewed in 2008). Summary of N, P, and K Research on Tomato in Florida. HS759. Gainesville: University of Florida Institute of Food and Agricultural Sciences. https://edis.ifas.ufl.edu/cv236.
Hochmuth, G. J., and E. A. Hanlon. 1989. Commercial Vegetable Crop Nutrient Requirements. Fla. Coop. Ext. Serv. Circular 806. Gainesville: University of Florida Institute of Food and Agricultural Sciences.
Hochmuth, G.J., and E. Hanlon. 1995a. IFAS Standardized Fertilization Recommendations for Vegetable Crops. Fla. Coop. Ext. Serv. Circ. 1152. Gainesville: University of Florida Institute of Food and Agricultural Sciences.
Hochmuth, G.J., and E.A. Hanlon. 1995b.Commercial Vegetable Crop Nutrient Requirements in Florida. Fla. Coop. Ext. Serv. SP177. Gainesville: University of Florida Institute of Food and Agricultural Sciences.
Hochmuth, G.J., and E.A. Hanlon. 2000. IFAS Standardized Fertilization Recommendations for Vegetable Crops. Fla. Coop. Ext. Serv. Circ. 1152. Gainesville: University of Florida Institute of Food and Agricultural Sciences.
Hochmuth, G.J., and E.A. Hanlon. 2009. Calculating Recommended Fertilizer Rates for Vegetable Crops Grown in Raised-bed Mulched Cultural Systems. SL303. Gainesville: University of Florida Institute of Food and Agricultural Sciences. https://edis.ifas.ufl.edu/ss516.
Hochmuth, G.J., and E.A. Hanlon. 2010a. Commercial Vegetable Fertilization Principles. SL319. Gainesville: University of Florida Institute of Food and Agricultural Sciences. https://edis.ifas.ufl.edu/cv009.
Hochmuth, G.J., and E.A. Hanlon. 2010b. Principles of Sound Fertilizer Recommendations. SL315. Gainesville: University of Florida Institute of Food and Agricultural Sciences.
Hochmuth, G.J., E. Hanlon, P. Gilreath, and K. Shuler. 1989. "Field Evaluations of Nitrogen Fertilization Programs for Subsurface-irrigated Tomatoes." Proc. Fla. State Hort. Soc. 102:351-4.
Hochmuth, G.J., E.A. Hanlon, P.R. Gilreath, and K.D. Shuler. 1991a. "Effects of K Rates on Yield of Tomato at Three Commercial Production Sites." Soil Crop Sci. Soc. Fla. Proc. 50:169-72.
Hochmuth, G.J., E. Hanlon, B. Hochmuth, G. Kidder, and D. Hensel. 1993. "Field Fertility Research with P and K for Vegetables — Interpretations and Recommendations." Soil Crop Sci. Soc. Fla. Proc. 52:95-101.
Hochmuth, G.J., and T.K. Hartz. 1996. "Fertility Management of Drip-irrigated Vegetables." HortTechnology 6:168-72.
Hochmuth, G.J., and D.N. Maynard. 1996. Vegetable Production Guide for Florida. SP170. Gainesville: University of Florida Institute of Food and Agricultural Sciences.
Hochmuth, G.J., D.N. Maynard, and M. Sherman. 1994a. Tomato Production Guide for Florida. Fla. Coop. Ext. Serv. Circ. 98C. Gainesville: University of Florida Institute of Food and Agricultural Sciences.
Hochmuth, G.J., D. Maynard, C. Vavrina, and E.A. Hanlon. 1991b. Plant Tissue Analysis and Interpretation for Vegetable Crops in Florida. Fla. Coop. Ext. Serv. Spec. Ser. SS-VEC-42. Gainesville: University of Florida Institute of Food and Agricultural Sciences.
Hochmuth, G.J., D. Maynard, C. Vavrina, E.A. Hanlon, and E.H. Simonne. 2009. Plant Tissue Analysis and Interpretation for Vegetable Crops in Florida. HS964. Gainesville: University of Florida Institute of Food and Agricultural Sciences. https://edis.ifas.ufl.edu/ep081.
Hochmuth, G.J., K.D. Shuler, E.A. Hanlon, and N. Roe. 1994b. "Pepper Response to Fertilization with Soluble and Controlled-release Potassium Fertilizers." Proc. Fla. State Hort. Soc. 107:132-9.
Hochmuth, G.J., and A.G. Smajstrla. 1997. Fertilizer Application and Management for Micro (drip)-irrigated Vegetables in Florida. Fla. Coop. Ext. Serv. Circ. 1181. Gainesville: University of Florida Institute of Food and Agricultural Sciences.
Hochmuth, R.C., E.H. Simonne, D. Studstill, J. Jones, C. Starling, J. Chandler, and A. Tyree. 2005. "On-farm Demonstrations of Soil Water Movement in Vegetables Grown with Plasticulture." Proc. Fla. State Hort. Soc. 118:184-6.
Hortenstine, C.C., and R.E. Stall. 1962. "The Effects of Ca and P Fertilization on Yield and Quality of 'Manapal' Tomatoes Grown on Virgin Immokalee Fine Soil." Soil Crop Sci. Soc. Fla. Proc. 22:125-31.
Kidder, G., E.A. Hanlon, G.J. Hochmuth. 1989. IFAS Standardized Fertilization Recommendations for Vegetable Crops. Fla. Coop. Ext. Serv. Spec. Ser. SS-SOS-907. Gainesville: University of Florida Institute of Food and Agricultural Sciences.
Lamberts, M., T. Olcyzk, Y.C. Li, H. Bryan, M. Codallo, and L. Ramos. 1997. "Field Demonstrations of Phosphorus Levels for Vine-ripe and Mature-green Tomatoes in Miami-Dade County." Proc. Fla. State Hort. Soc. 110:266-8.
Li, Y.C., R. Rao, H.H. Bryan, and T. Olcyzk. 1998. "Optimized Irrigation Schedule to Conserve Water and Reduce Nutrient Leaching for Tomatoes Grown on a Calcareous Gravelly Soil." Proc. Fla. State. Hort. Soc. 111:58-61.
Locascio, S.J., G.A. Clark, A.A. Csizinszky, C.D. Stanley, S.M. Olson, F. Rhoads, A.G. Smajstrla, G. Vellidis, R.J. Edling, H.Y. Hanna, M.R. Goyal, S. Crossman, and A.A. Navarro.1992. Water and Nutrient Requirements for Drip-irrigated Vegetables in Humid Regions. Southern Regional Research Project S-143 Report. Gainesville: University of Florida Institute of Food and Agricultural Sciences.
Locascio, S.J., J.G. Fiskell, and F.G. Martin. 1984. "Nitrogen Sources and Combinations for Polyethylene Mulched Tomatoes." Proc. Fla. State Hort. Soc. 97:148-50.
Locascio, S.J., and G.J. Hochmuth. 1994. "Potassium Source and Rate for Polyethylene-mulched Tomatoes." Proc. Tomato Inst. Fla. Coop. Ext. Serv. 105:103-9.
Locascio, S.J., G.J. Hochmuth, S.M. Olson, R.C. Hochmuth, A.A. Csizinszky, and K.D. Shuler. 1997a. "Potassium Source and Rate for Polyethylene-mulched Tomatoes." HortScience 32:1204-7.
Locascio, S.J., G. Hochmuth, F.M. Rhoads, S.M. Olson, A.G. Smajstrla, and E.A. Hanlon. 1997b. "Nitrogen and Potassium Application Scheduling Effects on Drip-irrigated Tomato Yield and Leaf Tissue Analysis." HortScience 32:230-5.
Locascio, S.J., S.M. Olson, and F.M. Rhoads. 1989. "Water Quantity and Time of N and K Application for Trickle-irrigated Tomatoes." J. Amer. Soc. Hort. Sci. 114:265-8.
Locascio, S.J., and A.G. Smajstrla. 1989. "Drip Irrigated Tomato as Affected by Water Quantity and N and K Application Timing." Proc. Fla. State Hort. Soc. 102:307-9.
Locascio, S.J., A.G. Smajstrla, and M.R. Alligood. 1996. "Nitrogen Requirements of Drip-irrigated Tomato." Proc. Fla. State Hort. Soc. 109:146-9.
McNeal, B.L., C.D. Stanley, L.A. Espinosa, and L.A. Schipper. 1994. "Nitrogen Management for Vegetables and Citrus: Some Environmental Considerations." Soil and Crop Sci. Soc. Fla. Procs. 53:45-51.
McNeal, B.L., C.D. Stanley, W.D. Graham, P.R. Gilreath, D. Downey, and J.F. Creighton. 1995. "Nutrient-loss Trends for Vegetable and Citrus Fields in West-central Florida: Nitrate." J. Env. Qual. 24:95-100.
Montelaro, J. 1978. Commercial Vegetable Fertilization Guide. Fla. Coop. Ext. Serv. Circ. 225-B. Gainesville: University of Florida Institute of Food and Agricultural Sciences.
Morgan, K.T., S. Sato, and E. McAvoy. 2009. "Preliminary Data on Phosphorus Soil Test Index Validation in Southwest Florida." Proc. Fla. State Hort. Soc. 122:233-9.
Muñoz-Carpena, R., H.H. Bryan, W. Klassen, and M.D. Dukes. 2003. "Automatic Soil Moisture-based Drip Irrigation for Improving Tomato Production." Proc. Fla. State Hort. Soc. 116:80-5.
Muñoz-Carpena, R., M.D. Dukes, Y.C. Li and W. Klassen. 2005. "Field Comparison of Tensiometer and Granular Matrix Sensor Automatic Drip Irrigation on Tomato." HortTech 15 (3):1-7.
Mylavarapu, R.S. 2009. UF/IFAS Extension Soil Testing Laboratory (ESTL) Analytical Procedures and Training Manual. Circular 1248. Gainesville: University of Florida Institute of Food and Agricultural Sciences. https://edis.ifas.ufl.edu/ss312.
Olczyk, T., R. Regalado, Y.C. Li, and R. Jordan. 2000. "Usefulness of Tensiometers for Scheduling Irrigation for Tomatoes Grown on Rocky, Calcareous Soils in Southern Florida." Proc. Fla. State Hort. Soc. 113 (2000):239-42.
Olson, S.M., W.M. Stall, G.E. Vallad, S.E. Webb, S.A. Smith, E. Simonne, E. J. McAvoy, and B. M. Santos. 2010. "Tomato Production in Florida." In Vegetable Production Handbook for Florida 2010-2011, edited by S.M. Olson and B. Santos. Gainesville: University of Florida Institute of Food and Agricultural Sciences.
Olson, S.M., and B. Santos. 2010. Vegetable Production Handbook for Florida. Gainesville: University of Florida Institute of Food and Agricultural Sciences. https://edis.ifas.ufl.edu/topic_vph.
Ozores-Hampton, M., E.H. Simonne, E. McAvoy, F. Roka, P. Roberts, P. Stansly, S. Shukla, T.A. Obreza, K. Cushman, P. Gilreath, J. Gilreath, and A. Whidden. 2005. "BMP Fertilizer Trials in Central and Southwest Fla." Proc. Tomato Inst.: 8-16.
Ozores-Hampton, M., E.H. Simonne, E. McAvoy, F. Roka, P. Roberts, P. Stansly, S. Shukla, K. Cushman, K. Morgan, T. Obreza, P. Gilreath, and D. Parmenter. 2006a. "Nitrogen BMP Efforts with Tomato Production in Florida in the 2005-2006 Season." Proc. Tomato Inst.: 16-23.
Ozores-Hampton, M., E.H. Simonne, G. McAvoy, F. Roka, P. Stansly, S. Shukla, P. Roberts, K. Morgan, K. Cushman, T. Obreza, P. Gilreath, and D. Parmenter. 2006b. "Nitrogen Best Management Practice with Tomato Production in Florida in the 2005-2006 Season." Proc. Fla. State Hort. Soc. 119:284-8.
Ozores-Hampton, M., E.H. Simonne, P. Gilreath, S. Sargent, D. McClure, T. Wilkes, E. McAvoy, P. Stansly, S. Shukla, P. Roberts, F. Roka, T. Obreza, K. Cushman, and D. Parmenter. 2007. "Effect of Nitrogen Rate on Yield of Tomato Grown with Seepage Irrigation and Reclaimed Water." Proc. Fla. State Hort. Soc. 120:184-8.
Ozores-Hampton, M., E.H. Simonne, K. Morgan, K. Cushman, S. Sata, C. Albright, E. Waldo, and A. Polak. 2010. "Can We Use Controlled-release Fertilizers in Tomato Production?" The Tomato Magazine: 8-15.
Poh, B.L., E.H. Simonne, R.C. Hochmuth, and D. Studstill. 2009. "Effect of Splitting Drip Irrigation on the Depth and Width of the Wetted Zone in a Sandy Soil." Proc. Fla. State Hort. Soc. 122:221-3.
Rhoads, F.M. 1997. Sap-test Nitrate-N of Fall Fresh Market Tomatoes. Fla. Agr. Expt. Sta. NFREC Research Report 97-7. Gainesville: University of Florida Institute of Food and Agricultural Sciences.
Rhoads, F.M., C.S. Gardner, O.S. Mbuya, G.L. Queeley, and H.M. Edwards. 1999. "Tomato Fertilization, Ground Cover, and Soil Nitrate Nitrogen Movement." Proc. Fla. State Hort. Soc. 112:315-19.
Rhoads, F.M., S.M. Olson, G.J. Hochmuth, and E.A. Hanlon. 1996. "Yield and Petiole-sap Nitrate Levels of Tomato with N Rates Applied Preplant or Fertigated." Soil Crop Sci. Soc. Fla. Proc. 55:9-12.
Rhoads, F.M., S.M. Olson, and A. Manning. 1988. "Nitrogen Fertilization of Staked Tomatoes in North Florida." Soil Crop Sci. Soc. Fla. Proc. 47:42-4.
Rhue, R.D., and P.H. Everett. 1987. "Response of Tomatoes to Lime and Phosphorus on a Sandy Soil." Agron. J. 79:71-7.
Santos, B.M., and J.W. Scott. 2008. "Response of 'Fla. 8153' Tomato to Nitrogen Fertilization Programs and In-row Distances." Hortscience 53:175-7.
Santos, B.M. 2008. "Evaluation of Nitrogen Rates and Irrigation Programs for Tomato Production in West Central Florida." Proc. Fla. State Hort. Soc. 121:164-6.
Sato, S., K. Morgan, M. Ozores-Hampton, and E.H. Simonne. 2009a ."Spatial and Temporal Distributions in Sandy Soils with Seepage Irrigation: I. Ammonium and Nitrate." Soil Science Society of America Journal 73 (3):1044-52.
Sato, S., K.T. Morgan, M. Ozores-Hampton, E.H. Simonne. 2009b. "Spatial and Temporal Distributions in Sandy Soils with Seepage Irrigation: II. Phosphorus and Potassium." Soil Science Society of America Journal 73(3):1053-60.
Schepers, J.S., K.D. Frank, and C. Bourg. 1986. "Effect of Yield Goal and Residual Nitrogen Considerations on Nitrogen Fertilizer Recommendations for Irrigated Maize in Nebraska." J. Fertilizer Issues 3:133-9.
Scholberg, J.M., and S.J. Locascio. 1999. "Growth Response of Snap Bean and Tomato as Affected by Salinity and Irrigation Method." HortScience 34:259-64.
Scholberg, J., B.L. McNeal, K.J. Boote, J.W. Jones, S.J. Locascio, and S.M. Olson. 2000. "Nitrogen Stress Effects on Growth and Nitrogen Accumulation by Field-grown Tomato." HortScience: 159-67.
Shuler, K. D. 1992. Tomato Yield Response to Reduced Rates of N and K Fertilizer and to a Slow Release Source of N and K, Capella Farms, Boca Raton, FL, Fall-Spring 1991-1992. Fla. Agr. Expt. Sta. Research: Palm Beach County Ext. Report. Gainesville: University of Florida Institute of Food and Agricultural Sciences.
Shuler, K.D., and G.J. Hochmuth. 1995. "Field Tests of Phosphorus Fertilization of Tomato Growing in High-P Soils in Palm Beach County, Florida." Proc. Fla. State Hort. Soc. 108:227-32.
Shuler, K.D., G.J. Hochmuth, and E.A. Hanlon. 1989. Effects of Reduced Nitrogen and Potassium Fertilization on Tomatoes in Boynton Beach, Florida, Fall/Winter 1988-89. Fla. Agr. Expt. Sta. Research Report: Palm Beach County Ext. Report. Gainesville: University of Florida Institute of Food and Agricultural Sciences.
Shuler, K.D., G.J. Hochmuth, and E.A. Hanlon. 1991. Tomato Yield Response to Reduced Rates of Nitrogen and Potassium Fertilizer, Thomas Produce, Boca Raton, FL, Fall-Winter 1990-91. Fla. Agr. Expt. Sta. Research Report: Palm Beach County Ext. Report. Gainesville: University of Florida Institute of Food and Agricultural Sciences.
Shuler, K.D., G.J. Hochmuth, E. A. Hanlon, and N. Roe. 1992. Tomato Yield Response to Reduced Rates of Nitrogen and Potassium Fertilizer, Neillco Farm, Boynton Beach, FL, Fall-Winter, 1991-92. Fla. Agr. Expt. Sta. Research Report: Palm Beach County Ext. Report. Gainesville: University of Florida Institute of Food and Agricultural Sciences.
Simonne, A.H., J.M. Fuzere, E. Simonne, R.C. Hochmuth, and M.R. Marshall. 2007. "Effects of Nitrogen Rates on Chemical Composition of Yellow Grape Tomato Grown in a Subtropical Climate." Jour. Plant Nutrition 30(6):927-35.
Simonne, E.H., M.D. Dukes, G.J. Hochmuth, B. Hochmuth, D. Studstill, and A. Gazula. 2006. "Monitoring Nitrate Concentration in Shallow Wells Below a Vegetable Field." Proc. Fla. State Hort. Soc. 119:226-30.
Simonne, E.H., M.D. Dukes, and L. Zotarelli. 2010. Principles and Practices for Irrigation Management for Vegetables. AE260. Gainesville: University of Florida Institute of Food and Agricultural Sciences. https://edis.ifas.ufl.edu/cv107.
Simonne, E.H., and G.J. Hochmuth. 2008. "Fertilizer and Nutrient Management for Tomato."Tomato Institute Proceedings: 55-7.
Simonne, E.H., and G.J. Hochmuth. 2010. "Chapter 2. Soil and Fertilizer Management for Vegetable Production in Florida." In Vegetable Production Handbook. HS711. Gainesville: University of Florida Institute of Food and Agricultural Sciences. https://edis.ifas.ufl.edu/cv101.
Simonne, E., R. Hochmuth, G. Hochmuth, and D.W. Studstill. 2008. "Development of a Nitrogen Fertigation Program for Grape Tomato." Journal of Plant Nutrition 31(12): 2145-54.
Simonne, E.H. 2009."Fertilizer and Nutrient Management for Tomato." Proc. Tomato Inst.: 41-4.
Simonne, E.H., D.W. Studstill, R.C. Hochmuth, G. McAvoy, M.D. Dukes, and S. Olson. 2003. "Visualization of Water Movement in Mulched Beds with Injections of Dye with Drip Irrigation." Proc. Fla. State Hort. Soc. 116:88-91.
Smajstrla, A., and D. Haman. 1998. "Irrigated Acreage in Florida:A Summary through 1998." Fla. Coop. Ext Serv. Cir 1220. Gainesville: University of Florida Institute of Food and Agricultural Sciences. https://edis.ifas.ufl.edu/ae150.
Smith, S.A., and T.G. Taylor. 1996. 1995-96 Production Cost for Selected Vegetables in Florida. Fla. Coop. Ext. Serv. Circ. 1176. Gainesville: University of Florida Institute of Food and Agricultural Sciences.
Soltanpour, P.N., and A.P. Schwab. 1977. "A New Soil Test for Simultaneous Extraction of Macro- and Micro-nutrients in Alkaline Soils." Commun. Soil Sci. Plant Anal. 8 (3): 195-207.
Stanley, C.D., and G.A. Clark. 1991. "Water Table Management Using Microirrigation Tubing." Soil and Crop Sci. Soc. Fla. Proc. 50:6-8.
Stanley, C.D., and G.A. Clark. 2003. "Effect of Reduced Water Table and Fertility Levels on Subirrigated Tomato Production in Southwest Florida." Fla. Coop. Ext. Serv. SL210. Gainesville: University of Florida Institute of Food and Agricultural Sciences.
Stanley, C.D., B.L. McNeal, P.R. Gilreath, J.F. Creighton, W.D. Graham, and G. Alveio. 1995. "Nutrient-loss Trends for Vegetable and Citrus Fields in West-central Florida: II Phosphate." Jour. Env. Quality 24:101-6.
Sweeney, D.W., D.A. Graetz, A.B. Bottcher, S.J. Locascio, and K.L. Campbell. 1987. "Tomato Yield and Nitrogen Recovery as Influenced by Irrigation Method, Nitrogen Source, and Mulch." Hortscience 22:27-9.
Taylor, M.D., S.J. Locascio, and M.R. Alligood. 2002. "Incidence of Blossom-end Rot and Fruit Firmness of Tomato Affected by Irrigation Quantity and Calcium Source." Proc. Fla. State Hort. Soc. 115:211-4.
VanSickle, J., S. Smith, and E. McAvoy. 2009. Production Budget for Tomatoes in the Manatee-Ruskin Area of Florida. Fla. Coop. Ext. Serv. FE817.Gainesville: University of Florida Institute of Food and Agricultural Sciences. https://ufdc.ufl.edu//IR00003785/00001.
VanSickle, J., S. Smith, and E. McAvoy. 2009. Production Budget for Tomatoes in Southwest Florida. Fla. Coop. Ext. Serv. FE818. Gainesville: University of Florida Institute of Food and Agricultural Sciences. https://ufdc.ufl.edu//IR00003786/00001.
Vavrina, C.S., G.J. Hochmuth, J.A. Cornell, and S.M. Olson. 1998. "Nitrogen Fertilization of Florida-grown Tomato Transplants: Seasonal Variation in Greenhouse and Field Performance." HortScience 33:251-54.
Wang, Q., H.H. Bryan, W. Klassen, Y.C. Li, M. Codallo, and A. Abdul-Baki. 2002. "Improved Tomato Production with Summer Cover Crops and Reduced Irrigation Rates." Proc. Fla. State Hort. Soc. 115:202-7.
Wang, Q., and W. Klassen. 2005. "Influence of Summer Cover Crops on Conservation of Soil Water and Nutrients in a Subtropical Area." J. Soil and Water Conservation 60:59-63.
Wang, Q., W. Klassen, H. Bryan, Y.C. Li, and A. Abdul-Baki. 2001. "Influence of Summer Cover Crops on Growth and Yield of a Subsequent Tomato Crop in South Florida." Proc. Fla. State Hort. Soc. 116:140-3.
Wang, Q.R., Y.C. Li, and W. Klassen. 2007. "Changes of Soil Microbial Biomass Carbon and Nitrogen with Cover Crops and Irrigation in a Tomato Field." Journal of Plant Nutrition 30(4): 623-39.
Wang, Q., W. Klassen, Y. Li ,and M. Codallo. 2009. "Cover Crops and Organic Mulch to Improve Tomato Yields and Soil Fertility." Agronomy Journal: 345-51.
Wang, Q., W. Klassen, Y. Li, M. Codallo, and A. Abdul-Baki. 2005. "Influence of Cover Crops and Irrigation Rates on Tomato Yields and Quality in a Subtropical Region." HortScience 40:2125-31.
Zotarelli, L., J. Scholberg, M.D. Dukes, R. Muñoz-Carpena, and J. Icerman. 2009a. "Tomato Yield, Biomass Accumulation, Root Distribution, and Irrigation Water Use Efficiency on a Sandy Soil, as Affected by Nitrogen Rate and Irrigation Scheduling." Agr. Water Management 96:23-34.
Zotarelli, L., M.D. Dukes, J.M.S. Scholberg, R.Muñoz-Carpena, and J. Icerman. 2009b. "Tomato Nitrogen Accumulation and Fertilizer Use Efficiency on a Sandy Soil, as Affected by Nitrogen Rate and Irrigation Scheduling." Agr. Water Management 96(8): 1247-58.