Florida strawberry production in 2007-08 resulted in 14,950,000 flats (10.25 lb/flat) harvested from 6,900 acres (Fla. Agri. Stat. Bulletin 2009), produced largely in Hillsborough, Polk, and Manatee counties in central Florida (90% of total state strawberry production). The market value was $146,119,000 from a five-month harvest period beginning in November and ending in April. In 2010, there were 8,500 acres with a value of $300 million. The largest strawberry yields are in March each year (30% of total yields).
This document is not meant to present fertilization recommendations. The intent of this document is to review literature from all sources that pertain to commercial strawberry fertilization in Florida's growing conditions. This publication documents the previously written literature, some of which now appears only in updated electronic format. All publications, including the reviewed older paper-format papers, will be placed in PDF format on the website https://bmp.ifas.ufl.edu for future reference.
Strawberry fertilization recommendations should be based on actual measurements, and a compilation of the literature contained in this document will assist with making valid fertilizer recommendations that are both commercially viable and reduce the risk of environmental consequences in adjacent water bodies. As environmental regulation becomes more commonplace, well documented fertilization recommendations based on published research are essential to support the industry and the environment.
Fertilizer recommendations not only contain the recommended rate of fertilizer but also the management strategies for getting the most out of the fertilizer investment while protecting the environment. These principles for fertilization of vegetables are summarized by Hochmuth and Hanlon (2010a). Rate is only a part of the modern fertilizer recommendation. Sound fertilizer recommendations also consider fertilizer materials, placement, and timing of application, among other aspects (Hochmuth and Hanlon 2010b).
More than 50 years worth of strawberry fertilization research has been conducted in Florida. During this time, many changes have occurred in strawberry production practices including changes in cultivars and the introduction of new cultural systems, including polyethylene mulch and drip irrigation (Albregts and Howard 1984b). Strawberries are grown on raised beds with two rows of plants per bed. Research with 2 and 4-row beds with drip irrigation showed little benefit of 4 compared to 2 rows in 2 of 3 years of the research (Albregts and Howard 1979). The research reported on here covers strawberry production with polyethylene mulch. Plastic mulch replaced no mulch and organic mulch (straw, pine straw, etc.) in the 1960s (Locascio 1964; Thompson 1957; 1959). Strawberry crop and fertilizer management recommendations, such as plant and row spacing, have changed with time in keeping with new developments in research (Montelaro 1978; Hochmuth 1988; 1996a; 1996b; Hochmuth and Albregts 1995; 2003; Hochmuth and Hanlon 1989; 1995a; 1995b; 2000; Kidder et al. 1989; Olson et al. 2010; Peres et al. 2009; Simonne and Hochmuth 2010). Fertilizer is a significant cost input. The most current recommendations for nutrient management in strawberry production are presented in the Commercial Vegetable Production Handbook for Florida (Olson and Simonne 2010). IFAS recommends a target of 150 lb/acre each of N, P2O5, and K2O only when soil concentrations of phosphorus (P) or potassium (K) are very low, based on results of Mehlich-1 (M-1) soil testing (Hochmuth and Hanlon 1995a; 2000; Peres et al. 2009).
The early research focused largely on yield and fruit quality (size and shape) in response to fertilization. Since the early research was conducted and the first review of the literature in 1999, there have been increasingly strong interests in the incorporation of more environmental impacts (water quality) into the fertilizer research. The state (Fla. Dept. of Agr. and Cons. Serv. 2005) has been formalizing best management practices (BMPs) and encouraging growers to implement BMPs. Part of the definition of a BMP includes consideration of both economics and environmental components and that a BMP embody the best available science.
This literature review includes all available published documentation concerning strawberry yield and fertilizer use in Florida. We chose to present all the research without being selective because that would introduce bias to the presentation. Most of the older literature is captured in separate figures in the review document. Inclusion of all Florida literature shows the development of the commercial production system with respect to fertilizer use. Commercial yields have been increasing primarily due to the proper selection of new cultivars with traits that resist disease and pest pressures. Since the basic plant has not changed, nutrient requirements have also been slow to change. In fact, nutrient use efficiency has increased while fertilizer rates have not.
The purpose of this publication is to summarize strawberry fertilization research, present the current University of Florida recommendations for strawberry fertilization, and point out needs for more research. Since nutrient and water management are linked, fertilization research is summarized by irrigation method. No research results have been published on P fertilization of strawberry. Reviews such as this document may be used in the process of determining or revising recommendations.
The fertilization recommendation addresses commercial yield and quality, the economics of crop production, and protection of the environment. Equally important is to have a mechanism available to the grower to adjust fertilization practices during the season because of leaching rains and extension of the growing season for additional harvests when market conditions are favorable. To address all of these concerns, UF/IFAS vegetable recommendations are given as a single target fertilizer rate that is projected to be sufficient for meeting the crop fertilizer needs for most growing seasons. This single target fertilizer rate is a recommended starting point and has been used historically in all vegetable fertilizer recommendations in Florida, as well as around the country. The target recommendation approach with footnotes was used by Montelaro (1978) and confirmed by Hochmuth and Hanlon (1995; 2000). The target value was derived from numerous fertilizer studies and represents a reasonable fertilizer rate that reflects the average maximum crop responses from all of the fertilizer research, not the extremes in responses. However, the recommendation process recognizes some growing seasons are different because of more leaching rains or increases in crop value that can lead to prolonged harvest windows. Hence, the target fertilizer amount is accompanied by a series of footnotes that explain the addition of supplemental fertilizer during the growing season, which includes addressing leaching rains and additional harvests near the end of the season. It is logical to select a single target rate based on research that avoids excessive fertilizer applications that often reduce crop nutrient efficiency and increase the potential for environmental degradation.
This publication is an updated version of a previous research literature review by Hochmuth and Cordasco (1999, reviewed 2009), covering research through 1996. This new review document adds the research reports conducted since 1996 and expands on the role of irrigation in managing fertilizer for crop production efficiency and environmental protection. The audience for this publication includes educators, such as Extension specialists and agents, and the commercial strawberry producers, consultants, and governmental agencies.
Data Summary Method
Evaluation of strawberry yield responses to varying rates of applied fertilizer required a standardized method of summarizing statewide yields, which were expressed variably as kg/ha, lb/acre, Mg/ha, and flats/acre (10.25 lb/flat to 12 lb/flat). In addition, strawberry yields vary depending on season, cultivar, and location in the state. Relative yield (RY), a calculated percentage, was chosen as the unit to express strawberry yield responses to fertilization. Relative yield is an accepted scientific method for summarizing and presenting data across wide sources and reports (Brown 1987).
In his book, C. A. Black (1992) summarizes the advantages and disadvantages of the RY approach. There are valid statistical concerns about RY, but Black concludes that the RY approach can be useful in displaying general relationships when applied properly and cautiously. Black demonstrates examples in his book where RY yield was helpful and where it was not. We chose the RY approach because we wanted to display the historical data without making biased decisions about what to include in the presentation. Plotting absolute yield data in original units obviously would result in a scatter of data rendering any general interpretation impossible. Black (1992) points out that decisions can always be improved with further research, but the data on hand are the best we have at the time. The highest yield for each fertilizer experiment was assigned a 100% value, and other yields were expressed as a percentage of the highest yield. The actual yield corresponding to 100% RY was presented in 10.25 lb/flat units. The RYs were plotted against rates of nutrient to determine how strawberry yields responded to fertilizer in Florida. The RY presentation allowed data from a variety of experiments to be included in the graphical summary of yield responses. For most studies, RYs of 90 to 100% were not significantly different.
Sometimes the argument is made that growers have expectations of greater yields than those obtained in research projects. It should be pointed out that realistic, regularly obtainable yields might be different from expected yields or "yield goals." Research on this subject has documented that 20% of growers actually reached their yield goals and only 50% reached 80% of their yield goals (Schepers et al.1986). Fertilizer rates should not be set on yield goals but rather on realistic goals based on research. This practice of setting yield goals is not recommended in Florida, so the effect of over-fertilization has been avoided by grounding expectations in measurement of observed yields. In effect, the current Florida fertilizer recommendation includes a component that addresses yield expectation versus actual production capability concern expressed by growers. Scientists throughout the years have conducted many replicated demonstrations studies in growers' fields with true commercial production practices, and those studies are included in this review. Excessive fertilizer was justified in times past when fertilizer was viewed as inexpensive insurance against yield loss. Research with numerous crops has shown that nutrient use efficiency declines as nutrient rate, especially N, increases. Even given the best of production systems, N use efficiency rarely exceeds 70% of the applied N. Concepts and practices for managing nutrients in vegetable production were summarized by Hochmuth (1992; 2000). Further, it has been suggested that the yields in older studies were much lower than yields obtained today. However, there are older research reports from the 1950s and 1960s where yields were as high as yields achieved today. The information presented above should have implications for the process to make a fertilizer rate recommendation.
Commercial weight of a strawberry flat has varied from 10.25 lb to 12 lb. A 10.25 lb/flat was used with all data presented for the actual treatment corresponding to 100% RY. The RYs were plotted against rates of nutrient to determine how strawberry yields responded to fertilizer in Florida. The RY presentation allowed data from a variety of experiments to be included in the summary of yield responses to fertilization. For most studies, RYs of 95 to 100% were not significantly different from each other.
While fertilizer rate is reported in pounds per acre (lb/acre), the reader should keep in mind that the bed system used in each field may vary and require adjustments to the fertilizer rate. To address this issue, the linear bed foot concept can be used to convert among the various bed designs with and without spray rows or ditches. Refer to Hochmuth and Hanlon (2009), "Calculating Recommended Fertilizer Rates for Vegetables Grown in Raised-Bed, Mulched Cultural Systems," https://edis.ifas.ufl.edu/publication/ss516. Additional cultural practices for commercial strawberry production can be found in Chapter 2 of the Vegetable Production Guide (Simonne and Hochmuth 2010).
Keeping nutrients in the soil profile and available for strawberry plant uptake requires proper irrigation management. Because of the critical nature of irrigation and drainage management with respect to nutrient uptake by the plant and possible loss of nutrients to the environment, information is regularly updated (Simonne and Dukes 2009).
Another useful tool for measuring nutrients, which contributes to appropriate management of fertilizer, includes plant tissue and/or plant sap sampling. For interpretation of plant and sap values, see Hochmuth (1994) and Hochmuth et al. (2009).
Strawberry production on Florida sandy soils presents unique nutrient demands due to the protracted six-month growing season. While N demands progressively decrease in the root and crown portion of the plant, the growing fruit begin to accumulate more N, P, and K than the combined nutrient demands of all other plant organs (Albregts and Howard 1979b; Albregts and Howard 1980b; Albregts and Howard 1981b). For maximum fruit yields, nutrients must be present in the greatest quantities during the second half of the season (January through April) to sustain fruiting. Nutrient analyses of dried strawberry plants sampled through the season revealed consistent nutrient accumulation trends among three sampled cultivars at Dover (1977 and 1978). The average N, P, and K accumulation in the plant at midseason and end-of-season samplings were 24 and 24.4 lb/acre N, 3.4 and 3.4 lb/acre P, and 16.8 and 20.2 lb/acre K, respectively. While accumulation of these nutrients in the plant was nearly unchanged during this period, nutrient accumulation in the fruit significantly increased from 9.3 to 28 lb/acre N, 1.8 to 5.0 lb/acre P, and from 12.2 to 36.1 lb/acre K at mid- and end-of-season sampling, respectively. Accumulation of N, P, and K by the fruit represented 53, 60, and 64%, respectively, of the total plant plus fruit accumulation of these nutrients by the end of the season. Researchers noted, however, that only small nutrient quantities were needed late in the season to replace those nutrients removed with the fruit. Excessive nutrient application late in the season is often unused by the plant and prone to buildup or leaching from the soil.
In earlier research, Albregts and Howard (1978) estimated the nutrients removed by the fruit and calyx to be 27 to 35 lb/acre N, 5.2 to 6.5 lb/acre P, and 34.9 to 45.6 lb/acre K (Albregts and Howard 1978). These results were similar to those presented above as seasonal nutrient accumulation. Researchers noted that fruit nutrient composition varied depending on plant genotype, soil composition, fertilization, and cultural factors. These data represented the fruit and calyx nutrient contents of four strawberry cultivars grown on Scranton fine sand (Dover) fertilized with 200 lb/acre N, 100 lb/acre P2O5, and 200 lb/acre K2O and mulched with polyethylene (irrigation unspecified). The yields of all tested cultivars averaged 2655 flats/acre using both the 1975 and 1976 seasons, a yield much higher than the state average (1537 flats/acre) for these years (Bertelsen 1995).
Mixed Fertilizer Trials
Overhead Irrigation
Mixed N-P-K fertilizers were often used in early strawberry experiments to establish crop nutrient requirements. Attributing crop response to a single nutrient, however, cannot be done with certainty from work with a blended N-P-K material. Yield responses in mixed studies were treated here as responses to N because N is the nutrient most often limited in sandy soils. Strawberry yields from mixed N-P-K fertilizer research were graphed separately (Figure 1).
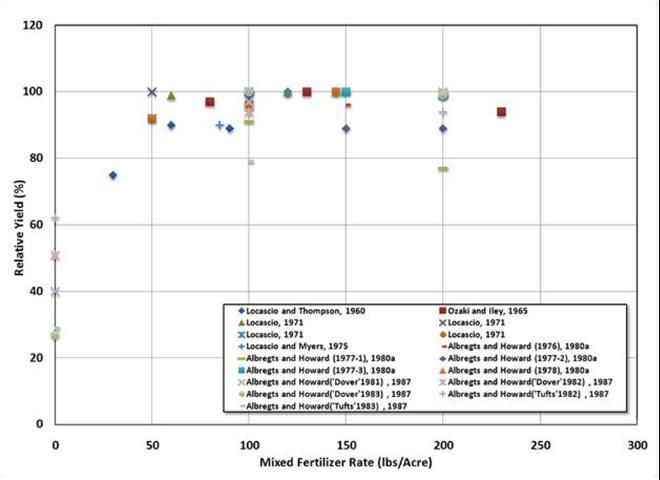
In one of the first nutrition studies with polyethylene-mulched strawberry in Florida, 'Florida Ninety' strawberry transplants were planted in Arredondo fine sand beds prepared with 6-8-6 (N-P2O5-K20) fertilizer applied in a narrow center band 6 inches beneath the bed surface (Locascio and Thompson 1960). Nitrogen rates were 30, 60, 90, and 120 lb/acre N. Beds were mulched with black polyethylene film. Yield response in this Gainesville experiment was linear through 120 lb/acre N, resulting in 812 flats/acre (100% RY). Significantly lower yields occurred with 30 lb/acre N: 608 flats/acre.
In Gainesville experiments, 1968-1969 and 1969-1970, sufficient rainfall was received (3.3 and 5.6 inches per month) each season so that supplemental irrigation was not required (Locascio 1971). The irrigation method in a 1970–1971 experiment was not specified. A 6-8-8 (N-P2O5-K2O) fertilizer was broadcast at increasing rates on plots that were then tilled, shaped into beds, and mulched with black polyethylene. 'Tioga' strawberries were planted each season. Yield variations among fertilizer rates were not different each year, resulting in 99 and 100% RY (12 lb, 562 flats/acre) with 60 and 120 lb/acre N (1969); 100% RY (778 flats/acre) and 98% RY with 50 and 100 lb/acre N (1970); and 92, 96, and 100% RY (1848 flats/acre) with 50, 100, and 145 lb/acre N (1971). Flats were described as 12-pint flats, no weight reference was indicated.
In 2005, red reflective mulch and black low-density polyethylene were tested at Gainesville, Hastings, Quincy, and Bradenton (Locascio et al. 2005). Fertilizer applications were similar among locations. While some early and total yield differences were observed between the two mulches at these locations, no consistent yield advantage for either mulch type was reported. The effect of fertilizer placement on nutrient retention in soil was studied in Dover on polyethylene-mulched, fine sand beds (Albregts and Howard 1973). In 1967–1968, a 4-8-8 (N-P2O5-K20) fertilizer derived from KCl and NH4NO3, was applied in 1- or 5-inch-deep bands (indicated as shallow or deep placement methods) at 120 lb/acre N. Strawberry yields were not affected by fertilizer placement. Based on soil fertility tests, researchers found that the inherent soil fertility was high and concluded that no response to fertilizer would be expected. Soil soluble-salt concentrations were measured late in the season (April 15) in samples taken 0 to 4 inches deep at bed center. Soluble-salt concentrations were nearly five times higher with the shallow compared to the deep placement method. In soil samples taken 4 to 8 inches deep, soluble-salt concentrations were two and a half times higher with the shallow fertilizer placement method. At this same April 15 sample date, K concentrations at the bed center, sampled 4 to 8 inches, were seven times higher, and NO3-N concentrations were 1.75 times higher with shallow fertilizer placement compared to deep placement. Soil soluble-salt concentrations measured in April were reduced by half from concentrations taken in January (samples taken at 0- to 4-inch depth at bed center) while soil concentrations of K and NO3 were not greatly depleted through the season in samples taken to the same depth at bed center. Nutrients present in the soil, below 12 inches deep, were considered inaccessible to strawberry plants.
A second experiment was conducted on lower fertility soils in the same area in 1971-1972. A 10-10-10 (N-P2O5-K20 [KNO3 and NH4NO3]) fertilizer was applied at rates of 0, 100, 200, 300, 400, and 1000 lb/acre N. Three placement methods were evaluated, including a fertilizer band placed 1 inch deep, fertilizer incorporated to 6 inches, or fertilizer half banded and half incorporated. Yields were similar with all placement methods, being optimal with 100 lb/acre N for an average yield of 478 grams/plant. Potassium and NO3-N concentrations sampled at midseason and harvest were similar with all placement methods with the lower N rates. Leaching losses, as measured by soil soluble salt, K, and NO3-N concentrations, occurred with the higher N rates with all fertilizer placement methods, with the heaviest losses occurring with broadcast/incorporated fertilizers.
Experimentation with 'Osmocote,' 16-5-16 (N-P2O5-K20), controlled release fertilizer, was conducted during three seasons in Dover; overhead irrigation was used in two of these experiments. The third experiment is summarized with drip-irrigated studies (Albregts and Howard 1980a). Nitrogen rates of 100, 150, or 200 lb/acre N were applied 1 inch below the bed surface at the bed center in 1976–1977 and 1977–1978. In the second year, two additional application methods were tested: band placement 4 inches below each row or 4 inches below and 2 inches inside the rows. High yields were expected from less fertilizer (lower N rates) thereby offsetting the cost of the resin-coated 'Osmocote' fertilizer. 'Florida Belle' strawberries were planted on Scranton fine sand beds, mulched with black polyethylene, and irrigated to keep the beds moist (use of tensiometers was not indicated). Yields were not affected by N rate in either season or by placement method in the second season. One hundred percent RY occurred each season with 100 lb/acre N (2429 and 1976 flats/acre) placed at bed center, 1 inch below the bed surface. These yields were 70 and 30% more than state average yields for each season, respectively.
A simultaneous series of experiments (1976 to 1979), also at Dover, were designed to test strawberry fruiting responses and potential N leaching losses from three consecutive annual applications of chicken manure (Albregts and Howard 1981a). In the fourth season, 50 lb/acre N (urea) was center-banded on all previously manured beds. Aged manure, 2.2% N, 6.6% P2O5, and 3.4% K2O by dry-weight analysis, was incorporated preplant into the top 6 inches of soil at 0, 4000, 8000, 16,000, and 32,000 lb/acre or 0, 90, 180, 350, or 700 lb/acre N. Beds were mulched with black polyethylene and planted with 'Florida Belle' strawberry each season. Yields increased quadratically at the 1% level (5% in 1978), with the highest yields at 180 to 700 lb/acre N. Foliage burn occurred in the first and third seasons, with 700 lb/acre N reducing yields. Relative yields with 350 lb/acre N were 100, 92, 100, and 89% or 2393, 2176, 2359, and 2568 flats/acre for each season, respectively. Although marketable fruit yields were high, 50 to 65% were then the state average annual yields; a large percentage of total yields were culled due to fruit rot with the 350 and 700 lb/acre N rates. Comparison of soil soluble-salt concentrations measured at 6, 12, 18, and 24 inch depths in the bed following the fruiting season in May, and before manure application in the fall, showed that N and K from the manure moved downward in the soil profile, while Ca and Mg accumulated in the top 24 inches of soil. Due to the high Ca concentrations in poultry manure and the potential to accumulate in the soil, researchers cautioned against frequent large applications of chicken manure. Downward movement of N and K was greatest during the summer season where soluble-salt concentrations from the top 6 inches of soil fell 2.5 to 4 times below concentrations measured in May.
Strawberry yielded more fruits with 200 lb/acre N compared to 100 lb/acre N from a mixed fertilizer (Albregts and Howard 1987). Response to soil-applied fertilizer was greater than responses to foliar-applied fertilizer.
Failure to hold nutrients in the root zone led researchers to seek other methods of replenishing leached fertilizers (Albregts et al. 1989). Application of late-season, side- and center-banded fertilizer, or foliar fertilizer application often resulted in soluble-salt injury when bands were placed improperly, or burned foliage at fertilizer rates necessary to satisfy crop needs. The fertilizer injection wheel (liquid fertilizer injected into the soil through the mulch) was the decided alternative method of adding late-season fertilizers without causing plant injury. Successive annual experiments, 1986-1987 and 1987-1988, were conducted in the same area at Dover on Scranton fine sand beds spaced 4 feet on center. Fertilizers were applied (1) at mulching; (2) by injection wheel (at one-month intervals) beginning November 17, 1986, at 10, 20, 30, and 40% N and K with each application and at 1.5 month intervals beginning December 2, 1987, at 30, 35, and 35% at each interval; (3) half at mulching/half by injection (applied at the later dates each season); or (4) at mulching with an additional 40 lb/acre N and K2O applied by injection in early March each season. Three N and K rates were used with each placement method for total N and K2O rates of 100, 150, and 200 lb/acre (1987) and 100, 175, and 250 lb/acre (1988). The fourth treatment was an additional 40 lb/acre N and K2O or 140, 190, and 240 (1987) and 140, 215, and 290 lb/acre total N and K2O. Yields, averaged using all N and K rates, were compared for the effects of placement method and time of application.
The lowest yields each season occurred when fertilizers were placed by injection wheel throughout the season: 1843 and 1829 flats/acre for 1987 and 1988 seasons, respectively. The highest yields each year were with fertilizers placed at mulching (2106 and 2358 flats/acre) or placed at mulching/injection with 40 lb/acre of additional injected N and K2O (2124 and 2344 flats/acre). Total marketable yields, percent marketable fruit, and average fruit weights were not improved by fertilizer injection in either season. Total marketable fruit yields were optimized with 192 lb/acre N and K2O, with the regression of yield vs. fertilizer rate being significant the first season but not the second. Since fertilizers injected as late as March had no yield effect, researchers concluded that higher percentages of fertilizer injected earlier in the season were preferable to late-season fertilizer injection.
Mixed Fertilizer with Subsurface Irrigation
Experiments through two seasons were conducted in Fort Lauderdale on soils 1.5 to 2 feet above the water table. Similar yields resulted in the first season on Delray fine sand with N and K rates of 80, 130, and 230 lb/acre N and K2O (NH4NO3, K2SO4) (Ozaki and Iley 1965). An initial 20 lb/acre N and K2O were placed in bands 3 inches to each side of plant rows before polyethylene mulch application. An additional 60 lb/acre N were applied half at four weeks before planting and half at two weeks before planting. One hundred percent RY resulted with 130 lb/acre N (1020 flats/acre). Relative yields with 80 and 230 lb/acre N were 97% and 94%, respectively. Ammoniated superphosphate and K2SO4 were broadcast, broadcast/rototilled, or banded in the second experiment on Pompano fine sand. Nitrogen and K rates were 60 or 120 lb/acre N and K2O. Placement methods interacted with fertilizer rates in this experiment. Yields with broadcast fertilizer increased with N rate resulting in optimum overall yields with 120 lb/acre N broadcast (1116 flats/acre). Yields with band-placed fertilizer decreased (likely due to soluble-salt injury with the higher N rate), and yields with broadcast/rototilled fertilizer were unchanged due to N rates. Other fertilizers were applied in separate treatments at only 120 lb/acre N broadcast or broadcast/rototilled. The highest yields, 1235 flats/acre, occurred with ureaformaldehyde (UFA) broadcast/rototilled.
Mixed Fertilizer with Drip Irrigation
Drip irrigation and fertigation systems increased nutrient management opportunities, including varying the proportion of preplant fertilizer (controlled-release [CR] or soluble fertilizer) to injected fertilizer and timing fertilizer application to meet plant growth. Experiments where both N and K rates were varied simultaneously are discussed here as yield responses to increasing N rates.
Experiments were conducted on polyethylene-mulched, Kanapaha fine sand beds near Gainesville, 1973-1974 (Locascio and Myers 1975; Locascio et al. 1977). Nitrogen rates of 85 and 120 lb/acre from a 6-8-8 (N-P2O5-K20) blended fertilizer (NH4NO3, superphosphate, and K2SO4) were applied either all preplant (overhead and drip-irrigated experiments) or applied 50% preplant and 50% fertigated for 20 weeks (140 days) beginning December 24. The overhead irrigation requirement was estimated from the Modified Blaney-Criddle formula and drip-irrigation water rates were a percentage (35 and 50%) of this estimated rate. Significantly higher yields occurred with 120 lb/acre N with all irrigation methods (1475 flats/acre, 100% RY), compared to 90% RY with 85 lb/acre N. With both drip and overhead irrigation, yields were similar when all of the soluble fertilizer was applied preplant with 120 lb/acre N (1379 and 1371 flats/acre), respectively. Seventeen percent higher yields (1655 flats/acre) resulted when fertilizers were applied 50% preplant and 50% fertigated (120 lb/acre N), with the lowest drip-irrigation rate (35% of overhead water use), compared to overhead irrigation with the same preplant N rate. On average, drip irrigation resulted in 12% greater early fruit yields (424 flats/acre) than with overhead irrigation (378 flats/acre) and produced 5% larger fruit by weight (10.20 versus 9.68 gm, respectively). All yields exceeded the state average yield (1320 flats/acre) for 1974 (Bertelsen 1995).
The following season, 1974-1975, N at 120 or 240 lb/acre from a 6-8-8 (N-P2O5-K2O) blended fertilizer was applied all preplant, 50:50 preplant/injected, or all injected (Locascio et al. 1977). Injected fertilizers were applied once per week for 20 weeks or in daily increments for 140 days. All other experimental conditions were the same as the 1973-1974 study above. Researchers observed no differences in yields due to N rate. Yields were averaged using both N rates and evaluated for response to fertilizer application and irrigation method (drip or overhead). Lower yields (25% lower) resulted when all of the fertilizer was applied preplant, or when all of the fertilizer was injected (11% lower) compared to yields with the 50:50 preplant/injected-fertilizer treatment (1782 flats/acre). Drip irrigation resulted in 13% more early fruit (970 flats/acre) and 12% more total season fruits (1640 flats/acre) than with overhead irrigation. Frequency of fertigation, daily or once per week, had no differential effect on early or total yields. Soil soluble-salt concentrations were 1.5 to 2.5 times higher with overhead irrigation compared to drip irrigation with soil moisture concentrations standardized to 10%. Soil moisture concentrations between 3.5 and 5.5%, however, were common with overhead-irrigated, mulched soils, resulting in high soil soluble-salt concentrations. Strawberry yields were reduced 50% when soil soluble-salt concentrations were increased to 2650 to 2900 ppm. Leaf-tissue N concentrations were greater than 3.1% with the first sampling and greater than 3.5% with later samplings, with treatments having no effect on leaf-tissue N concentrations.
Although drip irrigation and polyethylene mulch greatly reduces water use, nutrient leaching losses were indicated in the above experiment when 25% lower yields resulted where fertilizers were broadcast preplant compared to yields where fertilizers were applied 50:50 preplant/fertigated (Locascio et al. 1977).
Experimentation to document nutrient movement under polyethylene-mulched beds with drip irrigation was conducted near Gainesville on Blichton fine sand (Mansell et al. 1977). All plants received 120-160-160 lb/acre N, P2O5, and K2O. Soil NO3-N concentrations from daily-irrigated beds were compared with those from beds that received preplant broadcast fertilizer and for beds where fertilizer was broadcast/fertigated. On day 10 (one day following fertilizer injection), soil NO3-N concentrations 3 inches below the bed surface were 6.4 ppm with 100% broadcast N compared to 150 ppm with the 50:50 treatment. At depths of 6, 12, and 24 inches, respective soil NO3 concentrations were 19, 41.2, and 180 ppm (100% broadcast), compared to NO3 concentrations of 160, 280, and 190 ppm (50:50 treatment). Leaching losses were minimized at this site due to a slowly permeable subsurface soil horizon that led to N losses due to denitrification. Greater leaching losses may be expected on well-drained soils, researchers concluded.
Researchers hoped to reduce nutrient leaching losses with controlled-release nitrogen (CR-N) resin-coated 'Osmocote' fertilizer, 16-5-16 (N-P2O5-K20), applied 4 inches beneath the plant row (Albregts and Howard 1980a). This 1978-1979 Dover experiment was conducted on Scranton fine sand. Beds were mulched with black polyethylene, and 'Florida Belle' strawberries were planted. Drip irrigation was applied to keep beds moist (tensiometer use was not indicated). Yields were not affected by N at 100, 150, or 200 lb/acre, though a slightly higher yield occurred with 100 lb/acre N (2463 flats/acre, 100% RY). Yields were 60% greater than the state average yield of 1571 flats/acre for this season. Researchers obtained similarly high yields with overhead-irrigated experiments conducted over two previous seasons (summarized in the section covering overhead irrigation). Soil soluble-salt concentrations were measured in 6-inch soil cores taken at the end of the season (one of five samples was taken through the fertilizer band). Samples were analyzed for electrical conductivity (mhos/cm x 103) at soil saturation. Soluble-salt concentrations were similar for both overhead and drip irrigation where Osmocote was placed 4 inches beneath the row. Soil soluble-salt concentrations were not influenced by N fertilization with either irrigation treatment. Researchers concluded that 100 lb/acre N with 'Osmocote,' seven- to eight-month-release fertilizers, were sufficient for optimum strawberry yields, regardless of irrigation method.
Work with five N and K sources, applied either 100% preplant or 40% preplant and 60% injected as NH4NO3 + K2SO4 or KNO3 + Ca(NO3)2, was conducted near Gainesville on St. John and Blichton fine sand soils in 1978-1979 and 1981-1982 seasons, respectively (Locascio and Martin 1985). Beds were mulched with polyethylene, and yield responses to N source and fertilizer application method were evaluated using 120-160-160 lb/acre N, P2O5, and K2O. High yields (averaged using both seasons) with 100% preplant fertilizer application resulted with the CR-N sources, sulfur-coated urea (SCU) (1562 flats/acre) or 150 butylidene diurea (IBDU) (1547 flats/acre). Lower yields, 1210 flats/acre, resulted with 100% preplant-applied soluble N sources, urea, NH4NO3, or KNO3 + Ca(NO3)2. Yields with CR-N sources were similar regardless of preplant or split application, but yields with soluble N sources improved with split application (interaction), approaching yields with CR-N sources. Researchers noted that high strawberry yields were a response to continuous nutrient supply, regardless of N source. Leaf-tissue and soil N concentrations were not consistently affected by N source and time of application. Yields were generally lower than average statewide yields for the 1978 and 1981 seasons (1730 flats/acre).
Application of preplant fertilizers increased strawberry yields when combined 50:50 with injected N and K fertilizers. Researchers in Dover (1988-1989) tested soluble preplant fertilizers from 0 to 25% of total applied N and K fertilizers to determine the most effective ratio of preplant to injected fertilizers (Albregts et al. 1991a; Albregts et al. 1991b). Nitrogen and K were banded 1.5 inches below the bed surface and 1.5 inches above the buried drip tube. Mulched Seffner fine sand soils were maintained at -5 centibars or wetter, 6 inches below the bed surface. Preplant N rates of 0, 15, 30, 45, and 60 lb/acre N were applied jointly with preplant K rates of 0, 16, 32, 48, and 65 lb/acre K2O. Additional N and K2O were injected twice weekly (1 lb/acre/day) beginning November 19, one month after transplanting 'FL 79-1126' (October 19), totaling 180 lb/acre of each nutrient. Yield increased linearly with preplant N rate through 45 lb/acre N (225 lb/acre total N). High yields with this rate, 2211 flats/acre (100% RY), were less than the state average of 2537 flats/acre. With 60 lb/acre preplant N (240 lb/acre total N) yields fell to 94% RY. Significant increases in average fruit weight occurred between 0 and 15 lb/acre preplant N. With higher preplant N rates, average fruit weights were not increased.
In a research project report (Santos 2008) "Influence of Nitrogen Rates on Strawberry Cultivar Growth and Yield," 9 treatments resulted from 3 strawberry cultivars and 3 N fertilization rates (0.75, 1.25, and 1.75 lb/acre/day). No preplant fertilizer was added to the soil and beginning at 2 weeks after transplanting each N rate was injected 3 times per week using a hydraulic injector. Other essential nutrients were within sufficiency levels. A customized non-N formula was used to supply deficient nutrients along with N fertilization during each injection. Rates of N fertilization had a significant effect on plant diameter at 8 weeks with the largest plants with 1.25 and 1.75 lb/acre/day. Both cultivars had the same plant diameters, regardless of N rates, and the interaction between N rates and cultivars was not significant. Early marketable yield was only affected by cultivars, with 'Strawberry Festival' having higher values than 'Winter Dawn.'
Nitrogen
Sprinkler Irrigation
A three-year factorial study was conducted on previously cropped Kanapaha fine sand (1965 and 1966) and on Ona fine sand (1967) near Gainesville (Locascio and Saxena 1967). One-half of the fertilizer was broadcast and incorporated on 8 x 25 ft plots; beds were 4-ft wide with 2-ft-wide guard rows on each side. The remaining fertilizer was band-applied at the bed center, watered, mulched with black polyethylene, and planted with 'Florida Ninety' strawberries 12 inches apart. The N source was not cited.
'Florida Ninety' strawberry yields were not affected by increasing N rates from 60, 120, or 180 lb/acre N in 1965 or from 50, 100, or 150 lb/acre N in 1966 and 1967 (Figure 2). Annual 100% RYs were 750 flats/acre (average yields), 1083 flats/acre (greater than the state average), and 638 flats/acre (less than the state average) with 120 lb/acre N (1965) and with 100 lb/acre N (1966 and 1967), respectively. Researchers cited soluble-salt injury for the lower third-season yields. Dry soils between overhead irrigations on mulched beds likely exaggerated plant response to soluble-salt concentrations of 1964 ppm with 150 lb/acre N (soluble-salt concentrations in the range of 2000 to 2500 ppm are considered high for strawberry). Although strawberry yields did not respond to increasing N rates, leaf-tissue N concentrations at first sampling increased quadratically. Peak leaf-tissue concentrations occurred with 120 and 100 lb/acre N, 3.57 and 3.90%, in 1965 and 1966, respectively. At the second sampling, leaf-tissue N concentrations increased linearly with increasing N rates (1965 and 1966). In 1967, leaf-tissue N concentrations were high at both samplings and unaffected by N rate.
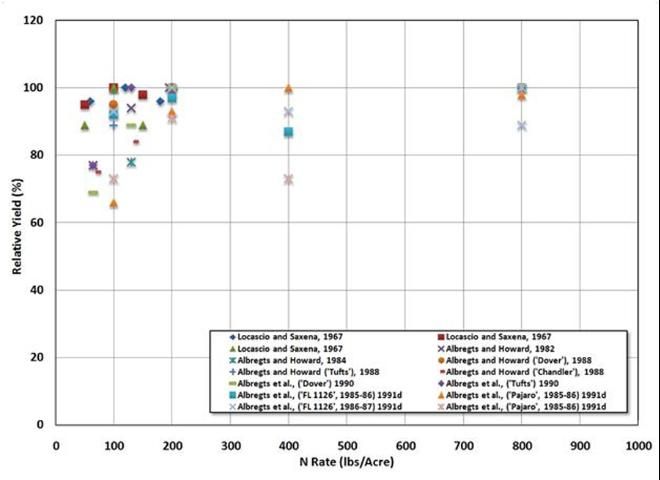
Total N content of fruits and the shear resistance increased with N rate throughout the range of 60 to 180 lb/acre N, but titratable acidity decreased (Saxena and Locascio 1968). Fruit K content and titratable acidity increased with K rate from 60 to 180 lb/acre K. Shear resistance was not affected by K rate. Source of K had no effect on fruit quality.
The effect of increasing N rates on the number of malformed strawberry fruit was studied in an experiment at Dover (Albregts and Howard 1982). Cultural practices were described as "standard to the area" (Albregts and Howard 1981a). Nitrogen rates of 65, 130, and 195 lb/acre N from isobutylidene diurea (IBDU) were applied with 60 lb/acre P2O5 and 195 lb/acre K2O. One-third of the P and K and 65 lb/acre N were placed 5 inches below the plant row, and the remainder banded 2 inches deep at bed center. Beds were mulched with black polyethylene and planted with 'Dover' and 'Tufts' strawberry transplants.
Numerous field conditions contribute to malformed fruit, among them are events during flowering that result in altered fruit shape such as fungicide application at flowering, insufficient insect pollinators, flowers eaten by insects, poor pollen quality, and adherence of flower petals to the fruit. Other factors affecting fruit formation are frost damage and plant overcrowding. Higher numbers of malformed fruit observed in fertile fields led to research on the effects of N fertilization on fruit formation (Albregts and Howard 1982). Linear (5% probability) increases in malformed fruit occurred as N rate increased through 195 lb/acre N. Researchers noted cultivar-specific responses to increased N, with more 'Tufts' strawberries deformed than 'Dover.' Yields, expressed as total number of fruits per plant, resulted in 100% RY with 195 lb/acre N (39.25 fruits/plant averaged using both cultivars), 94% RY, and 76% RY with 130 and 65 lb/acre N, respectively. Leaf N concentrations increased linearly with N rates, but leaf N concentrations of 2.5 to 2.94% were less than the adequate range of 3.0 to 4.0% for samples taken in December.
Controlled-release (CR) N fertilizers were evaluated at Dover for the best N release rate for an eight-month strawberry crop (Albregts and Howard 1984a). Researchers ultimately searched for a CR-N source to avoid plant and nutrient losses typical of soluble N fertilizers, including early season plant loss due to soluble-salt injury, midseason N and K leaching losses with freeze-protection irrigations, and late-season nutrient inadequacy when the nutrient demands of fruiting were high. Four CR-N sources were tested: urea-formaldehyde (UFA), heat-dried biosolids, biosolids and UFA (1:1), and organiform (leather tankage bonded with methylene urea). Nitrogen from each source at 65 lb/acre N was applied 5 inches beneath the row. Additional N to 130 or 200 lb/acre total N was banded at the bed center with 200 lb/acre K2O (KCl) and 50 lb/acre P2O5 (concentrated superphosphate CSP). Beds were mulched with black polyethylene. Strawberry yields, averaged using all CR-N sources, were higher with the 200 lb/acre N rate (3500 flats/acre, 100% RY) than with the 130 lb/acre N rate (78% RY). Plants fertilized with biosolids and 1:1 biosolids/UFA treatments yielded 30 to 60% more strawberries (3825 flats/acre) and had significantly greater soil NO3-N and NH4-N concentrations in December compared to UFA- and organiform-fertilized subplots. Researchers cited December and January temperatures two and four degrees colder than average for reduced microbial activity and mineralization of UFA and organiform CR-N sources.
Heat-dried biosolids and a 1:1 combination of biosolids and NH4NO3 consistently produced strawberry yields greater than those with 'Osmocote' (16-8-12 [N-P2O5-K20]) treatments for the two seasons, 1974-1975 and 1975-1976, in earlier Dover research (Albregts and Howard 1979a). Fertilizers (200 lb/acre N and P2O5 and 210 lb/acre K2O) were banded at bed center 1 inch below the bed surface and the Scranton fine sands were mulched with black polyethylene. In both seasons 100% RYs resulted from plants with the sludge treatment (2655 and 2803 flats/acre) compared to 98 and 87% RY with the NH4NO3 /sludge treatment, and 96 and 85% RY with the 'Osmocote' treatment for each respective year. Lower yields with 'Osmocote' in the second season resulted from placement 5 inches below the bed surface compared to a 1-inch-deep placement the previous season. Leaching losses measured by residual soil soluble-salt concentrations in February and April were greatest with the soluble N sources and least with 'Osmocote.' Soluble-salt concentrations with the sludge treatments were generally half of those with 'Osmocote.'
Yields of three strawberry cultivars, grown on black-polyethylene-mulched Scranton fine sand, were nonresponsive to fertilizers placed all broadcast or 25% broadcast and 75% banded in Dover experiments in 1983-1984 and 1985-1986 seasons (Albregts and Howard 1988). At midseason 1983-1984, soil electrical conductivity was significantly lower where fertilizer was broadcast than where it was banded. In December, February, and April 1985-1986, however, strawberry yields were unaffected by these soluble-salt concentrations. Yields were likewise unaffected by N source NH4NO3 or a 1:1 mix of NH4NO3 and sulfur-coated urea (SCU). Significant yield variation occurred with increasing N rates for the California cultivars 'Tuft' and 'Chandler.' Yields of 'Tuft' strawberries (1983-1984) increased from 89% RY with 100 lb/acre N to 100% RY (2633 flats/acre) with 200 lb/acre N. The following year, 'Chandler' strawberry yields increased linearly from 75% RY with 100 lb/acre N to 100% RY with 200 lb/acre N (2280 flat/acre).
The importance of CR-N sources in long-season strawberry production was studied earlier by Albregts and Howard (1984a). Sludge and sludge-containing N sources were found to be the best organic sources for cool-temperature N release when applied at 200 lb/acre N. Further work with CR-N sources involved inorganic N sources: isobutylidene diurea (IBDU), sulfur-coated urea (SCU), and 'Osmocote' (16-5-16 [N-P2O5-K20]) (Albregts et al. 1990). One-third of the IBDU or SCU fertilizers and all of the 'Osmocote' were applied 4 inches below the plant row with the remaining IBDU and SCU applied at bed center. Fields were mulched with black polyethylene and watered to maintain soil moisture between 8 and 12%. The N source had no effect on yields or leaf-tissue N concentrations of 'Dover,' but 'Osmocote' resulted in increased strawberry yields and leaf-tissue N concentrations of California 'Tufts' strawberries (interaction). An interaction also occurred between the N source and the fertilizer rate. Yields of both cultivars increased linearly with 'Osmocote' and SCU-N sources through 195 lb/acre N, but with IBDU, yields were lower and response to N was quadratic. One-hundred percent RYs with SCU and 'Osmocote' were 3334 and 3403 flats/acre (195 lb/acre N), respectively. Yield results with IBDU peaked at 3012 flats/acre with 130 lb/acre N (100% RY). All yields were elevated compared to the state average of 1902 flats/acre for those years. Of the CR-N sources tested, SCU and 'Osmocote' resulted in similar yields, average fruit weights, and leaf-tissue N concentrations.
Clones of California and Florida strawberries were grown with four experimental N rates from 100 to 800 lb/acre N near Dover, FL (Albregts et al. 1991c). Nitrogen rates were 100, 200, 400, and 800 lb/acre N derived from NH4NO3 and sulfur-coated urea (SCU) mixed 1:1. Twenty-five percent of the N fertilizers including 50 lb/acre P2O5 and 200 lb/acre K2O were broadcast, and the remainder of the N was banded 1 inch deep at bed center. Beds were mulched in black polyethylene and overhead irrigated to maintain soil moisture at 8 to 11% dry weight.
Strawberry yields from 1985 and 1986 experiments did not respond to N rates greater than 200 lb/acre N for either cultivar 'FL 79-1126' or California 'Pajaro.' Yields of the Florida cultivar responded quadratically (1986) to increasing N rates up to 200 lb/acre N, 97%, to 87% and 100% RYs with 400 and 800 lb/acre N, respectively. Increased N did not affect yields of 'Pajaro' strawberries in 1986 or cultivars '79-1126' and 'Pajaro' in 1987. Respective 100% RYs occurred with 400 lb/acre N (2594 flats/acre) in 1986, with 200 lb/acre (2159 flats/acre) in 1987, and with 800 lb/acre (1189 flats/acre) in 1987 ('Pajaro' strawberries). Reduced yields of 'Pajaro' strawberries in 1987 were caused by heavy rains. Yield responses were approximated state average yields of 1806 and 2200 flats/acre for 1986 and 1987, respectively. Leaf-tissue N concentrations responded quadratically to increasing N rates for both cultivars, generally leveling off with 200 lb/acre N at near-adequate concentration ranges.
One study was conducted testing the effect of frequency of foliar fertilization on 'Tufts' and 'Dover' strawberries (Albregts and Howard 1986). Foliar fertilization did not improve fruit yield compared to yield with the basic soil-directed program alone, which was 200N-16P-166K (lb/acre). Some high rates of foliar fertilizer reduced yields.
Nitrogen with Drip Irrigation
Results for strawberry response to fertilizer research with sprinkler irrigation are presented in Figure 3. No yield differences resulted when four CR and soluble N sources were placed on the bed surface or 1.5 inches below the bed surface with either drip irrigation or overhead irrigation (1974-1975) (Albregts and Howard 1978). Drip irrigation rates of 0.20, 0.40, or 0.80 inches of water per week, applied from drip tubes placed 1.5 inches beneath the bed surface, also had no effect on yields. Strawberry plants grown with irrigation rates between 0.40 and 0.80 inches of water per week, however, produced higher quality fruit compared to yields from plants receiving 0.20 inches/week. Fertilizer rates were 200 lb/acre each of N-P2O5-K2O. Slightly higher yields occurred with NH4NO3 applied inside a paper barrier (a 2-inch-wide paper strip formed into a V- shape and buried 3 inches beneath the bed surface) and with an 'Osmocote' (18-6-12 [N-P2O5-K2O]) treatment producing 2385 and 2455 flats/acre (100% RY), respectively. When drip irrigation tubing was placed on the bed surface and the same fertilizers were placed 1.5 inches below the bed surface, 1975-1976 strawberry yields were unaffected by N sources NH4NO3 (with or without a paper barrier), 'Osmocote,' urea, or UFA. High yields were 1889, 1793, and 1793 flats/acre with NH4NO3 (with paper barrier), NH4NO3 (without paper barrier), and 'Osmocote,' respectively. Soils fertilized with 'Osmocote' or NH4NO3 and the paper barrier had higher residual fertilizer based on concentrations of soluble salts (K, NH4-N, and NO3-N) sampled across the bed to 24 inches below the bed surface in January and May of each season. Yields for both seasons exceeded the state average yields (Bertelsen 1995) of 1610 and 1463 flats/acre for each year.
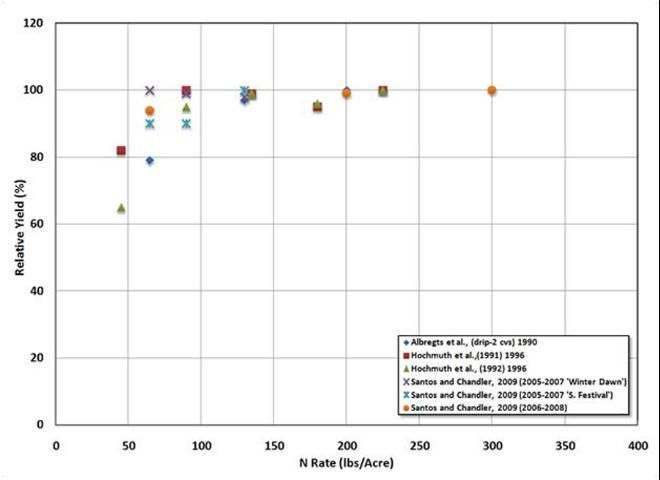
Additional research with overhead- and drip-irrigated strawberry concentrated on strawberry yield response to three CR-N sources in 1980-1981 and 1981-1982 seasons (Albregts et al. 1990). The overhead-irrigated portion of this research, presented with other overhead-irrigated experiments, resulted in interactions between N source and cultivar and between N source and N rate. No interactions occurred, however, when the same experiments were performed with drip irrigation. One-third of the IBDU and SCU fertilizers were applied 4 inches below the plant row and the remainder applied in the drip line. All of the 'Osmocote' (16-5-16 [N-P2O5-K2O]) was applied 4 inches below the plant row. Fields were mulched with black polyethylene and irrigated to maintain soil moisture between 8 and 12%. Total yields of both cultivars ('Tufts' and 'Dover') were averaged and 100% RY (3045 flats/acre) resulted with 200 lb/acre N. The significance of yield differences between N rates of 130 and 200 lb/acre N was not indicated, though 97% RY occurred with the 130 lb/acre N rate. Increasing N rates resulted in lower average fruit weights of drip-irrigated 'Dover' strawberries.
Later research with N sources (Santos 2010; Santos and Ramirez-Sanchez 2009) found that plants with all preplant N and S sources had improved early fruit yields compared with the control (no preplant fertilizer). Total fruit yield was best with ammonium sulfate, ammonium sulfate nitrate, polymer-coated ammonium sulfate, and elemental sulfate. The research showed the importance of S in the preplant fertilizer material and that preplant N mostly impacted early yield.
A rate of 90 lb/acre N was sufficient for high-yield strawberry production with drip-irrigated/fertigated, polyethylene-mulched strawberry in Dover (Hochmuth et al. 1996). Soils low in organic matter (0.1%) were known in previous studies to respond to applied N. Strawberry transplants were set into the bed in mid-October 1991 and 1992, and fertigation began October 21 and October 18 of each year and continued through April 30 each season (190 days). A solution of urea and NH4NO3 was injected once weekly for total season N rates of 45 through 225 lb/acre N. Injected K (KCl) totaled 170 lb/acre K2O for the season. Drip irrigation was used to maintain soil moisture between -5 and -15 kPa, measured by tensiometer.
Strawberry yields in 1991-1992 were 2811 flats/acre with 90 lb/acre N (100% RY). Yields were near the state average yield of 2927 flats/acre for that year. Early yields averaged using all N rates were higher with the University of Florida cultivar 'Sweet Charlie' (678 flats/acre), compared to early yields of 478 flats/acre for the California cultivar, 'Oso Grande.' Yields of cull fruit were 35% of the total 'Sweet Charlie' yields, however, compared to 22 and 18% with 'Oso Grande' for both seasons. The amount of culled fruit increased with N rate during the first season but was not affected by N rate in the second season. Total marketable yields between cultivars were not different. Yields were higher overall in the second season (1992-1993) with California cultivars 'Oso Grande' and 'Seascape,' but were not affected by increasing N rates. Maximum yield was predicted with linear and quadratic plateau models at 85 lb/acre N, near the 90 lb/acre N rate, which yielded 3313 flats/acre (95% RY). State average yields were 2730 flats/acre for this season. Fruit firmness throughout the 1992-1993 season was not affected by N rate.
'Sweet Charlie' and 'Camerosa' cultivars were used during the 2000-2001 cropping season (Simmone et al. 2001). Both cultivars were combined with N rates of 66, 100, or 133 lb/acre, equivalent to 0.5, 0.75, and 1.0 lb/acre/day, and two irrigation rates. The number of irrigation drip tapes was varied to create the two irrigation rates. Marketable yields from both cultivars responded differently to N rate but not irrigation rate. The authors concluded that the 100 lb/acre N rate was appropriate for both cultivars and that it might be possible to lower irrigation rate by as much as 20%, saving water and reducing costs for the grower.
Strawberry cultivars 'Winter Dawn,' 'Strawberry Festival,' and 'Ventana' were grown in three seasons, 2003, 2004, and 2005 in Dover, Florida (Santos et al. 2006), to investigate N rates and irrigation amounts. N rates were 65, 75, and 90 lbs/acre in the first two seasons, and 60, 90, and 120 in the final season. In the first season, N rates did not affect fruit yield per month or total season. Yield increased with N rate for 'Strawberry Festival' but not for the other two varieties in the latter two seasons. Where N rate influenced fruit yield, the highest chosen treatment was lower than the recommended target seasonal N rate (Hochmuth and Albregts 2003; Perez et al. 2009).
Studies (Santos and Chandler 2009) were conducted near Balm, Florida, on the response of 'Strawberry Festival' and 'Winter Dawn' strawberries to different N rates. In the 2005–2006 and 2006–2007 growing seasons, N rates were 0.4, 0.6, and 0.8 lb/acre/day (65, 90, and 120 lb/acre/seasons). These seasonal rates were all less than the recommended projected seasonal amount (150 lbs/acre) of N (Peres et al. 2009). A second set of studies was conducted during the 2006–2007 and 2007–2008 growing seasons using N rates of 0.8, 1.25, and 1.8 lb/acre/day (65, 190, and 265 lb/acre/season) with the same cultivars. The recommended rate of N fell between the first two treatment levels in this experiment. In the first two years, N rate linearly increased plant canopy diameters (vegetation) of both cultivars. In the first two years (data combined using all years), there was a significant cultivar by N rate interaction for total marketable fruit weight. Increasing daily N rates from 0.4 to 0.8 lb/acre/day (increasing the total season N from 65 to 120 lb/acre) resulted in a linear increase in total marketable yields of 'Strawberry Festival,' but the yield of 'Winter Dawn' was not affected by N rate. N rate only influenced strawberry plant canopy diameters, but not early or total marketable fruit weight and number for the same cultivars in a second experiment. In the second two seasons, average fruit yield was greater than for the first two seasons. In the latter two seasons, 65 lb/acre of N was sufficient for strawberry yield. Taken together, these two studies demonstrate differences in variety requirements but, in any case, the seasonal N requirements were less than the currently recommended N rates. These later studies with different varieties confirm earlier research by Hochmuth et al. (1996), where 90 lb/acre N was found to be sufficient for high yields.
Strawberry production is highest in March when Florida-grown strawberries produce one-third of their seasonal yields (Hochmuth et al. 1996). Using the linear-plateau model, researchers have determined at least 0.70 lb/acre/day fertigated N provides optimum N for these high March yields. Based on this analysis, current fertigation recommendations are set at 0.3 lb/acre/day N in weeks one and two of the season, 0.6 lb/acre/day N in November, December, January, and April, and 0.75 lb/acre/day N during the high N demand period of February and March (Hochmuth and Smajstrla 1997). Evaluation of whole-leaf N concentrations, which were interpreted as less than adequate through the second season, indicate that current published adequate concentrations may be too high for fertigated strawberry and recalibration is needed. Petiole-sap nitrate concentrations were also lower than the published rates for November, 600-900 ppm compared to 800-900 ppm (Hochmuth 2009) and April results of 100–200 ppm compared to 200–500 ppm.
Nitrogen Summary
Overhead-irrigated experiments dominated N research with strawberry (1965–1991). High yields occurred in 84% of the experiments with mixed fertilizers or isolated N fertilizer with 200 lb/acre or less. Heat-dried sludge or sludge combined 1:1 with NH4NO3 or UFA resulted in higher yields than with CR inorganic N sources including 'Osmocote.' IBDU fertilization often resulted in lower strawberry yields than either SCU or 'Osmocote.' Strawberry plants, sensitive to soluble-salt concentrations as low as 2000 to 2500 ppm, were injured by increased soluble-salt concentrations when polyethylene-mulched soils, fertilized with soluble N sources, dried between overhead irrigations. Band or broadcast fertilizer placement had little effect on yields of overhead-irrigated strawberry, though leaching losses were greater with broadcast soluble fertilizers.
Drip-irrigated strawberries were found to respond to N fertilization similarly to overhead-irrigated strawberries when soluble fertilizers were applied all before planting. Generally, split applications of preplant and injected fertilizers resulted in 10% higher yields, 12% more early fruit, and 5% larger fruit, compared to yields with all preplant fertilizer and overhead irrigation. The potential for high yields with lower N rates with fertigation was realized with 90 lb/acre N when the entire N amount was injected as NH4NO3 one week from transplanting through the end of the season (180 days). Petiole-sap and leaf-tissue N concentrations of fertilized strawberries were also lower than the published ranges, and researchers recommended additional experimentation to set sap N-concentration ranges specific to fertigated strawberry. Yields were lower with mixed N-P-K fertilizer experiments where fertigation began two months after transplanting and continued for 140 days as opposed to the 180-day fertigation schedule described above. Successful fertilization practices involved maintaining a continuous nutrient supply throughout the six-month strawberry season by beginning fertigation earlier in the season and extending fertigation to 180 days and by using CR-N fertilizers to make up a portion of the preplant fertilizer. All broadcast soluble fertilizers were found to leach beneath polyethylene mulch despite lower water use with drip irrigation, resulting in yields 25% lower than 50:50 applied broadcast/injected soluble fertilizers or all CR fertilizers applied preplant.
Irrigation and Nutrient Management
Strawberries are grown on sandy soils with little water-holding capacity, thus soluble nutrients, such as N, can move with the water front. Leaching is possible from heavy rainfall or excessive irrigation. Irrigation is required for successful production of strawberries in Florida. Irrigation is used for plant growth, development, and fruiting. Sprinkler irrigation also is used for freeze protection. Depending on the freeze severity and wind speed, variable amounts of water are required (Locascio et al. 1967). In addition to plant growth and freeze protection, water is required for transplant establishment (Albregts and Howard 1985). Many studies have been conducted through the years to evaluate the water needs for plant establishment in the fruiting field. The traditional method has been, and continues to be, sprinkler irrigation. In a typical season, approximately one-third of the total season irrigation is applied to each of these components: plant establishment, crop growth, and freeze protection. Early studies in Dover, Florida, investigated the use of intermittent irrigation and antitranspirants as a means to reduce the water needs (Albregts and Howard 1976). Intermittent irrigation saved more than one-half of the amount of water used by the standard treatment (8 hours of continuous irrigation daily throughout a period of 10 to 14 days), and there were no differences among one-quarter or one-half hour periods as long as the mulch and leaves remained moist during the off-period. Antitranspriants did not have an effect on plant establishment or fruit yield.
Various intermittent irrigations from 3 minutes on and 17 minutes off to 15 minutes on and 15 minutes off were compared with continuous irrigation for plant establishment in 1978 and 1979 seasons in Dover, Florida (Albregts and Howard 1985). Water usage was greatly reduced (up to one-half) with intermittent irrigation. Treatments with short on periods, such as 3 in 17 minutes and 5 in 25 minutes resulted in negative impacts of plant survival and fruit yield. The number of old leaves remaining on the plant after the establishment period (14 days) was positively correlated with fruit yield.
Through the years there have been numerous studies of strawberry irrigation in Florida. Early strawberry culture was practiced with subsurface irrigation (Osaki and Iley 1965), and with sprinkler irrigation (Locascio et al. 1967; Myers and Locascio 1972). Drip irrigation came into research in the early 1970s (Myers and Locascio 1972). Sprinkler, furrow, and drip (trickle) irrigation methods were compared for effects on polyethylene-mulched 'Tioga' fruit yield in 1971–1972 on a Kanapaha fine sand soil near Gainesville, Florida (Myers and Locascio 1972). Total yield and average fruit size were not affected by irrigation method. Soil soluble salts accumulated in the upper and center part of the raised bed with sprinkler and furrow irrigation, but no soluble salt accumulation was measured with the drip irrigation. Drip irrigation maintained more uniform soil moisture compared to fluctuations with the furrow and sprinkler irrigation methods. Drip irrigation resulted in one-half of the water use compared with sprinkler and furrow irrigation.
Drip irrigation was applied at rates of 5, 10, and 22 mm per week (0.2, 0.4, and 0.8 inches per week) to fruiting strawberries in Dover, Florida (Albregts and Howard 1978). Irrigation rates had no effect on fruit yields or soil soluble salts, but fruit quality (fruit color) was poor with the lowest irrigation rate. The researchers concluded that between 0.4 and 0.8 inches per week irrigation were needed, but if the pan evaporation was greater than 1.0 inch per week then more irrigation may be required.
Distributions of nitrate and K ions in the soil were evaluated for a polyethylene mulched strawberry crop in Gainesville, Florida (Mansell et al. 1977). Nutrients moved deeper below the bed surface when all fertilizer was applied preplant with daily irrigation compared to a treatment where part of the fertilizer was applied with the irrigation during the season. Locascio et al. (1977) observed the greatest yields resulted when 50% of the N and K fertilizer was applied through the drip irrigation system (fertigation). Thus, increased yields and reduced nutrient leaching resulted from fertigation with strawberry on sandy soils.
Drip irrigation rate had no effect on strawberry yield in three trials in Dover, Florida, in 2003–2005 seasons (Santos et al. 2006). Irrigation treatments were 5.2, 6.9, 8.6, and 10.3 acre-inches per season (1 acre-inch = 27,100 gals). The current target irrigation management program would result in about 8.6 acre-inches. In another irrigation study, researchers determined that strawberries could be irrigated with less (7 acre-inches) than the recommended rates for the first 8 weeks of the season (Ramirez-Sanchez 2009). For one variety, 'FL-99-117' there was no influence of irrigation volume or frequency. For 'Strawberry Festival,' early yield was not affected by the irrigation program. Total yield improved with additional water volume when two irrigation cycles per day were used, but not with a single irrigation event. More irrigation applied more frequently was needed for highest total yields, but less water in single applications sufficed for early production.
Two recent papers addressed the issue of water use for plant establishment with a different approach than focusing on the irrigation water. Currently the strawberry crop is started with bare-root plants dug fresh from a nursery, typically in northern climates (e. g., North Carolina, Canada). These plants require irrigation to keep the leaves on the plants during the establishment phase while the plants develop roots and start to grow. Strawberry transplants also can be produced in a greenhouse in containers that produce a growing plant with an intact root system, a containerized or "plug" transplant. Plugs can be used to establish a strawberry crop without sprinkler irrigation, using only water applied with the transplant at planting (Hochmuth et al. 2006a). Early yield, the most valuable portion of the crops is enhanced with plugs compared to bare-root plants (Hochmuth et al. 2006b). Plug technology results in more costly plants, but may return more profit with increases in early yields. Plug technology greatly reduces the irrigation needs for plant establishment, but needs to be demonstrated on a large scale with the strawberry industry.
Early research on water requirements of drip-irrigated strawberry were summarized in early Extension documents by G. A. Clark (1991; 1993). These Extension fact sheets showed the importance of understanding evapotranspiration and irrigation management for scheduling irrigation. More current information is needed that couples irrigation and nutrient management to keep both nutrients and water in the root zone. Optimizing these two important inputs will maintain the profitability of strawberry production in Florida while protecting the environment from nutrient leaching losses.
Phosphorus and Potassium
Soil Testing
Mehlich-1 extractant indices (expressed as ppm soil-extracted nutrient) are classified as very low, low, medium, high, and very high, and a crop-specific fertilizer recommendation is made from that classification (Hochmuth and Hanlon 1995a; 2000; Mylavarapu 2009). The M-1 solution became the accepted extractant standard in 1979 at the University of Florida. Previous to M-1, ammonium acetate and water extractants were used. Indices recorded from these methods in early research cannot be directly equated with current M-1 indices, but review of these early studies presents a profile of strawberry response with varying fertilization conditions. This review also summarizes practices in water management, fertilizer application methods, fertilizer source, and the effect of mulch in the nutrient management system.
Phosphorus
There has been scant research on P with strawberry in Florida. Since a 1956 study (Dennison and Hall 1956), no P research has been conducted on Florida-grown strawberries. Results of this study indicated a P-soil interaction where strawberry yields responded to applied P when soil P concentrations were low but did not respond when soil P concentrations were high. This finding describes the basis of fertilizer recommendations where the nutrient contribution of the soil is considered before additional fertilizer is recommended.
Potassium
The response of strawberry to applied K fertilizer varied with cultivar (Albregts et al. 1991d). Strawberry genotypes such as 'Dover' and 'FL 79-1126' were more responsive to applied K fertilizer than California cultivars such as 'Chandler' and 'Tufts.' The authors cited differences in plant breeding conditions between the two locations as well as the ability of California cultivars to substitute Na for K during stress periods.
The success or failure of a K fertilization program, however, depends largely on whether sufficient K concentrations are present from flowering through fruiting, generally from December to February. In central Florida, the maximum plant growth period is in February. From first flowering to the last harvest, plant dry weight doubles and the developing fruits accumulate more N and K than all other plant organs combined (Albregts and Howard 1980b; Albregts and Howard 1981b). Effective strawberry fertilization programs must provide a continuous nutrient supply to carry this long-season crop through the demands of late-season fruiting.
Factorial combinations of N and K (NH4NO3 and KCl) were tested in 1965–1966 and 1970–1971 experiments (Albregts and Sutton 1971). Neither irrigation nor experimental location was specified in this study, although Dover and overhead irrigation were the likely site and irrigation used in these experiments. In 1965-1966, N and K were applied equally at rates of 90, 180, or 270 lb/acre N and K2O, to previously cropped Scranton fine sand (4% organic matter). All treatments received a single broadcast application of 20-40-40 lb/acre N, P2O5, and K2O and band placement of one-sixth of the total N and K rate at the bed center, 4 inches deep at mulch application (November 22). The remaining fertilizers were broadcast on September 21 (at fumigation) and October 18 (at transplanting). In 1970–1971, half of the fertilizer was incorporated in the bed and half was banded 2 inches below the bed surface at the bed center. Total N and K rates were 0, 100, 200, and 300 lb/acre N and K2O. Black polyethylene mulch was applied immediately afterwards. Yields were not affected by increasing K rates in either experiment. One-hundred percent RYs resulted in 287 gm/plant with 90 lb/acre K2O (1966) and 201 gm/plant with 0 lb/acre K2O (1971). High yields occurred with saturated-water-extracted K concentrations of 72 ppm (1966) and 70 ppm (1971) from soil samples taken in February each year. Researchers concluded that 70 ppm of saturated-water-extracted K was sufficient for high yields. Soil K concentrations in saturated-water extracts increased linearly through 252 and 275 ppm each year with increasing rates of applied K. Yields were unaffected by the increased soil K concentrations either year.
Overhead Irrigation
Researchers in a previously presented three-year N and K factorial study (Locascio and Saxena 1967) found that 'FL Ninety' strawberry yields did not respond to increasing N rates in any season (Figure 4). Likewise, increasing K rates had no effect on yields in 1964–1965 or in 1965–1966. Soil K concentrations in saturated-water extracts were tested with an unnamed soil test and results expressed as 73 to 89 lb/acre K (37 to 45 ppm). With this soil K concentration, 100% RY (760 and 1075 flats/acre, each year; flat weight not specified) resulted with the lowest K rates, 70 and 40 lb/acre K2O, respectively. In the 1966–1967 season, strawberry yields decreased linearly from 100% RY (656 flats/acre) with 0 lb/acre K2O to 90 and 80% RY with 80 to 160 lb/acre K2O, respectively. Lower overall yields in this third season were significantly correlated with foliar composition of N, K, and Mg. Researchers concluded that lower Mg concentrations in plant leaf tissue resulted from greater plant uptake of Ca and K. Best yields occurred in the previous season when both Mg and Ca concentrations in the leaf tissue were high. Researchers also cited the yield-reducing effects of high soil soluble-salt concentrations in the third season. Soluble-salt concentrations were 1800 ppm, measured at field capacity. Higher concentrations were likely on soils that dried between overhead irrigations, with concentrations in the range of 2000 to 2500 ppm proven detrimental to strawberry. Varying K rates had little effect on fruit quality, soluble-solids, acidity, or fruit firmness. Likewise, K sources, K2SO4, KNO3, and KCl, had no effect on fruit yields or leaf-tissue K concentrations each year.
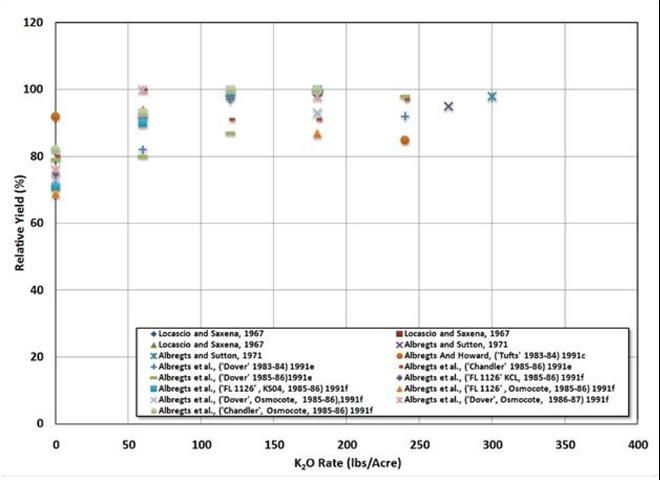
Responses to K fertilization were evaluated with 'Dover' and 'Tufts' in 1983 and with 'Dover' and 'Chandler' in 1984 season in Dover, Florida, with sprinkler irrigation (Albregts and Howard 1991c). The soil tested 23 (very low) and 30 ppm (low) Mehlich-1 in 1983 and 1984, respectively. K recommendations would have been 150 lb/acre K2O. 'Dover' fruit yield responded to K both seasons to about 180 lb/acre K2O, close to the recommended amount. 'Tufts' and 'Chandler' did not respond to K fertilization. These varieties apparently were more efficient in the utilization of K from the soil.
Variations in yield, average fruit weight, and leaf-tissue K concentration were evaluated for 'Dover' and 'Chandler' strawberry cultivars grown with K rates from 0 to 240 lb/acre K2O (Albregts et al. 1991d). The research was conducted at Dover during the 1983–1984 and 1985–1986 seasons on Seffner fine sand fields prepared in beds on 4-foot-wide centers. Potassium (KCl) was applied at 0, 60, 120, 180, and 240 lb/acre K2O with 200 lb/acre N and 40 lb/acre P2O5. One-fourth of the fertilizer was broadcast-incorporated preplant, and the remainder was banded 1.5 inches deep at bed center. Beds were mulched in black polyethylene and received overhead irrigation as needed. Yields of the Florida cultivar 'Dover' increased linearly with increasing K rates in both seasons, while yields of the California cultivar 'Chandler' were not affected by K fertilization in 1985–1986 (1985 yield data not presented). Soils (M-1) tested low for K, 23 and 30 ppm each year, and older K recommendations for irrigated mineral soils (Kidder et al. 1989; Montelaro 1978) were revised to 120 lb/acre K2O (Hochmuth and Hanlon 1995). 'Dover' strawberry yields were maximized with 180 lb/acre K2O (100% RY, 2698 and 2994 for each season), 40% more than the state average yields for these seasons. Leaf-tissue K concentrations were less than the sufficiency threshold (1%) with K rates less than 120 lb/acre K2O, resulting in K deficiency symptoms and RYs of 78 to 81% with 0 and 60 lb/acre K2O. Potassium deficiency symptoms did not appear with 'Chandler' strawberries. Average fruit weight of 'Dover' strawberries declined linearly with increasing K rate (1983) but was not affected by higher K rates in 1985–1986.
Studies involving CR-K fertilizers were conducted simultaneously with the 1985-1986 experiment summarized above (Albregts et al. 1991e). Location, bed preparation including black polyethylene mulch, N-P-K rates, and fertilizer placement methods were the same as above. Potassium sources were KCl, sulfur-coated K2SO4, and resin-coated K2SO4 ('Osmocote'). Although plants fertilized with 'Osmocote' were more responsive to increasing K rates compared to plants fertilized with other fertilizers, overall marketable yields were similar with all K sources. The optimum K rate with 'Osmocote,' applied 25% preplant and 75% at the bed center on low M-1 potassium soils (30 and 31 ppm each year), was between 110 and 170 lb/acre K2O. Similarly, high yields (yield data not given) with KCl and sulfur-coated K2SO4 occurred with 145 and 160 lb/acre K2O, respectively. These latter rates were slightly greater than the 128 lb/acre K2O recommended in 1978 (Montelaro 1978) and more than the updated 120 lb/acre K2O recommendation in 1995 for low M-1 K soils.
Subsurface Irrigation
A strawberry fertilization experiment was conducted near Fort Lauderdale on Delray fine sands with the water table at 1.5 to 2 feet (Ozaki and Iley 1965). Nitrogen and K were applied factorially at rates of 80, 130, or 230 lb/acre N and K2O (NH4NO3 and K2SO4). Initial fertilizer applications included 20-40-20 lb/acre N, P2O5, K2O applied in bands 3 inches to each side of the plant row and 60-0-60 lb/acre N, P2O5, K2O divided and applied, as the preceding fertilizer, at four and two weeks before planting. The remaining fertilizers were banded to achieve the rates given above, and beds were mulched with polyethylene. Yields were not greatly affected by increasing K rates, although slightly higher yields occurred with 130 lb/acre N and K2O (1134 flats/acre). Relative-yield responses to 80, 130, and 230 lb/acre K2O (averaged using all N rates) were 95, 100 (1067 flats/acre), and 96%, respectively. Potassium concentrations in leaf tissue taken in April were adequate and similar with both 80 and 130 lb/acre K2O. Researchers concluded that soil nutrient concentrations were high, based on the previous cultivation history, and the soil organic-matter content was high.
Drip Irrigation
Results from studies with potassium with drip irrigation are presented in Figure 5. The movement of nutrients in mulched, drip-irrigated soils was evaluated near Gainesville, Florida, in 1974-1975 (Mansell et al. 1977). Blichton fine sand soils were fertilized with 120-160-160 lb/acre N, P2O5, K2O (NH4NO3, superphosphate, and K2SO4), which were broadcast on unirrigated or daily-irrigated plots before bed preparation and three weeks before planting. In a third treatment, half of each of the N and K (and all of the P) was broadcast preplant, and the remaining N and K fertilizers were injected weekly. Estimates of crop water use on polyethylene-mulched beds were made using the Modified Blaney-Criddle equation. Daily irrigation applied to broadcast soluble fertilizers resulted in nutrient leaching from the root zone. Soil K+ concentrations were measured from the soil solution, extracted by porous ceramic cups placed in a partial air vacuum. These cups were placed 1.5 ft from tensiometers and extracted from 20 to 50 ml of soil water during a four-hour period. Soil K+ concentrations measured in March, April, and May ranged from 3.8 to 0.7 ppm, 3 inches below the bed surface, compared to 23.0 to 8.7 ppm, 24 inches below the bed surface. Soil K+ concentrations were generally higher where K was applied 50:50 preplant/injected. Soil K+ concentrations one day following weekly fertilizer injection were 48.7, 50, 81.7, and 31.5 ppm, compared to 3.3, 8, 16.7, and 20.7 ppm with broadcast preplant fertilizers (irrigated daily) at corresponding depths of 3, 6, 12, and 24 inches below the bed surface.
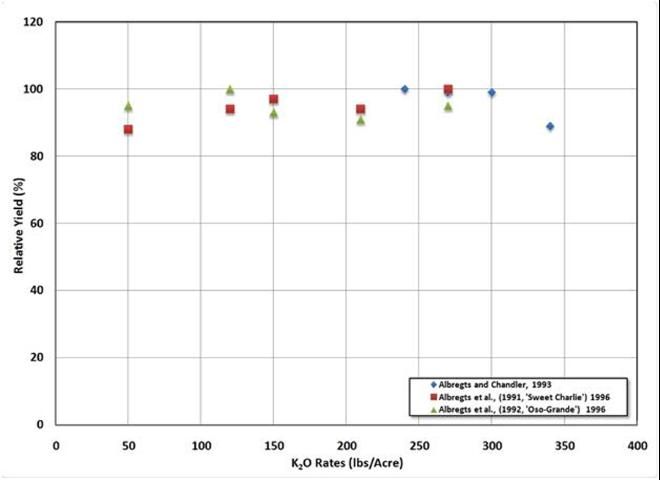
With drip irrigation, proportional combinations of CR preplant fertilizer and soluble injected fertilizer were compared for their effects on yield of polyethylene-mulched strawberry in Dover, 1991–1992 (Albregts and Chandler 1993). A controlled-release N-P-K fertilizer (16-5-16 [N-P2O5-K2O]) was applied preplant at N and K rates of 0, 50, 100, and 150 lb/acre N and K2O. The total N rate was limited to 200 lb/acre by reducing the amount of injected N on beds with higher preplant N rates. Total preplant plus injected K rates were 340, 300, 270, and 240 lb/acre K2O. Where no preplant N and K were applied, fertilizer injection began 30 days earlier and continued for 193 days. Injected K totaled 340 lb/acre K2O. Yields were significantly (5%) lower (2918 flats/acre) with this treatment compared to yields averaged using K rates from 240 to 300 lb/acre K2O (3250 flats/acre) where 20 to 60% were applied preplant from CR-K sources. Researchers suggested that the higher injected K rate (340 lb/acre K2O) may have reduced yields on this M-1 low K soil (27 ppm) where recommendations specified 120 lb/acre K2O (1995). With a fifth treatment, yields were the same with 20% soluble preplant K as with 20% CR preplant K (300 total lb/acre K2O): 3274 and 3267 flats/acre, respectively. All yields were greater than the state average of 2927 for this season. Leaf-tissue K concentrations were adequate with all treatments.
Work with fertigated strawberry led to additional Dover studies in 1991–1992 and 1992–1993 (Albregts et al. 1996). Nitrogen and K were applied only through the drip lines for total K rates of 54, 110, 160, 215, or 270 lb/acre K2O and a total N rate of 130 lb/acre. Fertigation, begun one week from transplanting, occurred once weekly and continued for 175 days. Beds were mulched with black polyethylene and drip-irrigated to maintain soil moisture between -5 and -15 kPa as measured by tensiometer. Strawberry marketable yields, averaged from cultivars 'Oso Grande' and 'Seascape,' did not respond to applied K in either season, despite M-1 recommendations of 120 and 100 lb/acre K2O for soils testing low (27 ppm) and medium (46 ppm) in K each season, respectively. Yields with K rates of 54, 110, 160, 215, and 270 lb/acre K2O were 2301, 2470, 2536, 2460, and (100% RY) 2624 flats/acre (1992) and 3127, (100% RY) 3287, 3059, 2991, and 3114 flats/acre (1993) with respective K rates. Leaf K concentrations from petiole sap taken late each season were deficient through 110 lb/acre K2O in the first season and both leaf-tissue K and petiole-sap K concentrations were deficient with 54 lb/acre K2O late in the second season. These late deficiency results did not affect fruit quality or fruit yields, which exceeded the state average each season (2927 and 2731 flats/acre). Researchers felt strawberry plants efficiently utilized fertigated K in these experiments but recommended additional research before reducing current K rates.
Potassium Summary
Half of the above summarized experiments occurred before the use of M-1 soil testing (1979). High yield responses in these experiments resulted from K applications in the range of 0 to 130 lb/acre K2O, near the current maximum recommended rate of 150 lb/acre K2O for soils M-1 very low in extractable K (M-1 soil test). Higher K rates reduced yields linearly in one experiment and had no yield effect in other experiments. Where M-1 soil testing was implemented, yields in 70% of experiments were unaffected by increasing K rates, and in 30% of experiments required 1.5 times the recommended amount of K.
Fertilizer injection begun shortly after transplanting and continued throughout the season resulted in highly positive strawberry yield responses to N at rates well below the recommended rate. Yield responses to similarly injected K fertilizers, however, were unaffected by K rates from 110 to 270 lb/acre K2O. Injection of K at more than twice the recommended rate, however, resulted in 11% lower yields compared to the combination preplant/injected K. Potassium leached readily with drip irrigation when all of the K was applied broadcast preplant. Soil K concentrations within the root zone were ten times higher when K was applied 50:50 preplant/weekly injection compared to all-broadcast preplant K and drip irrigated daily. UF/IFAS preplant recommendations with overhead irrigation are for 25% broadcast K and 10 to 20% broadcast K with subsurface-irrigated strawberry. The remaining K fertilizer should be banded in the bed center and mulched with polyethylene to reduce K leaching losses for this long-season crop.
Overall Summary
State harvested strawberry acreage has increased nearly three times from 1700 to 4700 acres, averaged using two ten-year periods from 1970 to 1980 and 1980 to 1990, respectively. On average, total state strawberry yields have increased four times, and per-acre production efficiency has increased from 1380 to 2035 flats/acre during these same periods (Bertelsen 1995). Based on the above summarized research, optimum strawberry yields can be maintained with current IFAS recommended fertilizer rates. Nearly 80% of all N and K research conducted in the past forty years have occurred with overhead irrigation, but with increased usage of drip irrigation and fertigation, higher yields are possible with lower N rates and lower water use. With overhead irrigation, yields were most often maximized with 150 to 200 lb/acre N; however, with fertigation, N requirements were shown to be less than 150 lb/acre N. With further research it may be possible to adjust N recommendations downward for fertigated strawberry. Studies are needed also on optimal timing of N application with drip irrigation systems and on the fate of N in the soil system as it relates to N and irrigation management. Recommended nutrient management programs also need to be demonstrated on commercial farms. As improved CR-N and K fertilizers become commercially available, their effectiveness throughout the long strawberry season will require evaluation. Current K recommendations appear to support high strawberry yields, but researchers recommended band application of most of the applied K with subsurface- and overhead-irrigated strawberry, and split preplant/injected K application with fertigated strawberry. Potassium was proven to leach from beneath polyethylene mulch when broadcast entirely at preplant. Nearly all strawberries are now grown with drip irrigation and fertigation. Current N and K recommendations for soils testing low in K are for 150 lb/acre each of N and K2O. Fertilization of strawberries with these amounts of N and K appears to be justified by the results of more than forty years of research. More research is needed with P to determine if P is still needed on Florida strawberry soils that have been fertilized with P for many years.
Some best management practices supported in the research literature are as follows:
- Use soil testing to determine P and K fertilizer requirements.
- Fertigation for N and K should be standard practice for applying the majority of N and K during the season. Injection rate should approximate the growth pattern of the crop—less early in the season when fruit load is low and more in February and March with heavy fruit production.
- Current UF/IFAS N recommendations (Perez et al. 2009) for fertigation target 150 lb/acre N for the typical strawberry season in central Florida from late September into April. More N may be needed if the harvest season is to be extended.
- Preplant N benefitted early yields but not total season; impact of sulfur was greater than N for fruit yield.
- Drip irrigation water requirements increase in the season. Lower amounts are needed for the first eight weeks than for the remainder of the season. With high water needs the frequency should be two applications instead of once per day.
Literature Cited
Albregts, E. E., and C. K. Chandler. 1993. Slow release fertilizer rates for strawberry fruit production. Proc. Fla. State Hort. Soc. 106:187–189.
Albregts, E. E., G. A. Clark, C. D. Stanley, F. S. Zazueta, and A. G. Smajstrla. 1991a. Preplant fertilizer for microirrigated strawberry. pp. 158–159. In: The Strawberry into the 21st Century. Proc. of 3rd North American Strawberry Conference. Adam Dale and James Lube. Timber Press, Portland, Oregon.
Albregts, E. E., G. A. Clark, C. D. Stanley, F. S. Zazueta, and A.G. Smajstrla. 1991b. Preplant fertilization of fruiting microirrigated strawberry. HortScience 26(9):1176–1177.
Albregts, E. E., G. J. Hochmuth, C. K. Chandler, J. Cornell, and J. Harrison. 1996. Potassium fertigation requirements of drip-irrigated strawberry. J. Amer. Soc. Hort. Sci. 121(1):164–168.
Albregts, E. E., and C. M. Howard. 1973. Influence of fertilizer placement and rates on strawberry production and soil fertility. Soil Crop Sci. Soc. Fla. Proc. 32:89–92.
Albregts, E. E., and C. M. Howard. 1976. Effect of intermittent irrigation and antitranspirants on establishing strawberry transplants on mulch. Proc. Fla. State Hort. Soc. 89:85–86.
Albregts, E. E., and C. M. Howard. 1977. Influence of fertilizer sources and drip irrigation on strawberries. Soil Crop Sci. Soc. Fla. Proc. 37:159–162.
Albregts, E. E., and C. M. Howard. 1978. Elemental composition of fresh strawberry fruit. J. Amer. Soc. Hort. Sci. 103(3):293–296.
Albregts, E. E., and C. M. Howard. 1979. Effect of two and four row beds with drip or sprinkler irrigation on strawberry fruiting response. Proc. Fla. State Hort. Soc. 91:73–74.
Albregts, E. E., and C. M. Howard. 1979a. Effect of bed height and N fertilizer sources on fruiting strawberries. Soil Crop Sci. Soc. Fla. Proc. 38:76–78.
Albregts, E. E., and C. M. Howard. 1979b. Nutrient accumulation by strawberry plants. Dover Agric. Res. Center Res. Rep. SV-1979-1.
Albregts, E. E., and C. M. Howard. 1980a. Fruit yield of Florida Belle strawberries as affected by rates of a resin-coated fertilizer. Soil Crop Sci. Soc. Fla. Proc. 39:14–16.
Albregts, E. E., and C. M. Howard. 1980b. Accumulation of nutrients by strawberry plants and fruit grown in annual hill culture. J. Amer. Soc. Hort. Sci. 105(3):386–388.
Albregts, E. E., and C. M. Howard. 1981a. Effect of poultry manure on strawberry fruiting response, soil nutrient changes, and leaching. J. Amer. Soc. Hort. Sci. 106(3): 295–298.
Albregts, E. E., and C. M. Howard. 1981b. N, P, K composition of and accumulation by strawberry plant organs from transplanting through fruit harvest. Soil Crop Sci. Soc. Fla. Proc. 40:30–3.
Albregts, E. E., and C. M. Howard. 1982. Effect of fertilizer rate on number of malformed strawberry fruit. Proc. Fla. State Hort. Soc. 95:323–324.
Albregts, E. E., and C. M. Howard. 1984a. Effect of three slow-release fertilizers on fruiting strawberries. Soil Crop Sci. Soc. Fla. Proc. 43:10–11.
Albregts, E. E., and C. M. Howard. 1984b. Strawberry production in Florida. Fla. Coop. Ext. Serv. Bull. 841.
Albregts, E. E., and C. M. Howard. 1985. Effect of intermittent sprinkler irrigation on establishment of strawberry transplants. Soil and Crop Sci. Soc. Fla. 44:197–199.
Albregts, E. E., and C. M. Howard. 1986. Supplemental foliar fertilization of fruiting strawberries. Proc. Fla. State Hort. Soc. 99:329–331.
Albregts, E. E., and C. M. Howard. 1987. Fertilizer rate and method of application on fruiting strawberry. Proc. Fla. State Hort. Soc. 100:198–200.
Albregts, E. E., and C. M. Howard. 1988. Effect of fertilizer placement, source, and rate on strawberry fruiting response. Soil Crop Sci. Soc. Fla. Proc. 47:146–149.
Albregts, E. E., and C M. Howard. 1991c. Potash fertilization for overhead sprinkler irrigated strawberry in the fruiting field. University of Florida, IFAS, Dover AREC Research Report DOV-1991-5.
Albregts, E. E., C. M. Howard, and C. K. Chandler. 1989. Fertilizer injection into strawberry fruiting beds. Soil Crop Sci. Soc. Fla. Proc. 48:108–111.
Albregts, E. E., C. M. Howard, and C. K. Chandler. 1991d. Effect of high N rates on fruiting strawberry. Soil Crop Sci. Soc. Fla. Proc. 50:134–136.
Albregts, E. E., C. M. Howard, and C. K. Chandler. 1991e. Strawberry responses to K rate on a fine sand soil. HortScience 26(2):135–138.
Albregts, E. E., C. M. Howard, and C. K. Chandler. 1991f. Effect of potassium sources and rates on fruiting response of three strawberry clones. Advances in Strawberry Production. 10: 37–39.
Albregts, E. E., C. M. Howard, and C. K. Chandler. 1991g. Slow release fertilizers for strawberry production. Proc. Fla. State Hort. Soc. 104:244–245.
Albregts, E. E., C.M. Howard, C. K. Chandler, and F. G. Martin. 1990. Fruiting response of strawberry as affected by rates and sources of controlled-release N fertilizer, and irrigation method. Soil Crop Sci. Soc. Fla. Proc. 49:46–49.
Albregts, E. E., and Paul Sutton. 1971. Response of strawberry to N and K fertilization on a sandy soil. Soil and Crop Sci. Soc. of Fla. Proc. 31:114–116.
Bertelsen, Diane. 1995. The U.S. Strawberry Industry. U.S.D.A. Stat. Bul. 914.
Black, C. A. 1992. Soil fertility evaluation and control. Lewis Publishers, Boca Raton, Fla. 746 pp.
Brown, J. R. 1987. Soil testing: sampling, correlation, calibration, and interpretation. Soil Science Society of Amer. Special Publ. No. 21. Soil Science Society of America, Inc. Madison, Wisc.
Clark, G. A. 1991. Water management for drip-irrigated strawberries. Univ. Fla. Gulf Coast Res. and Educ. Center Bradenton Research Report BRA-1991-9.
Clark, G. A. 1993. Water requirements for drip-irrigated strawberries in south central Florida. Univ. Fla. Gulf Coast Res. and Educ. Center. Bradenton Research Report BRA-1993-2; Drip Tips No. 9301.
Dennison, R. A., and C. B. Hall. 1956. Influence of nitrogen, phosphorus, potash and lime on the growth and yield of strawberries. Proc. Fla. State Hort. Soc. 69:220–8.
Florida Dept. of Agriculture and Consumer Services. 2005. Water quality/quantity best management practices for Florida vegetable and agronomic crops. http://www.floridaagwaterpolicy.com/PDF/Bmps/Bmp_VeggieAgroCrops2005.pdf.
Florida Dept. of Agriculture and Consumer Services. 2009. Florida Agricultural Statistics. http://www.florida-agriculture.com/fass_activities.htm.
Hochmuth, G. J. 1988. Commercial Vegetable Fertilization guide. Fla. Coop. Ext. Serv. CIR 225C.
Hochmuth, G. 1992. Concepts and practices for improving nitrogen management for vegetables. HortTechnology. 2 (1):121–125.
Hochmuth, G. J. 1994 (reviewed in 2009). Plant petiole sap-testing for vegetable crops. Fla. Coop. Ext. Serv. Circ. 1144. https://edis.ifas.ufl.edu/pdffiles/cv/cv00400.pdf.
Hochmuth, G. 1996a. Vegetable fertilization pp. 3-17. In: G. Hochmuth and D. Maynard (eds.). Vegetable production guide for Florida. Fla. Coop. Ext. Serv. Circ. SP 170.
Hochmuth, G. J. 1996b. Commercial Vegetable Fertilization guide. Fla. Coop. Ext. Serv. CIR 225D.
Hochmuth, G. 2000. Management of nutrients in vegetable production systems in Florida. Soil and Crop Sci. Soc. Fla. Proc. 59:11–13.
Hochmuth, G. 2009. Plant petiole sap-testing guide for vegetable crops. Fla. Coop. Ext. Serv. Circ. 1144.
Hochmuth, G. J., and E. E. Albregts. 1995. Fertilization of strawberries in Florida. Citrus and Vegetable Grower Magazine, March 1995. pp.20–24.
Hochmuth, G. J., and E. E. Albregts. 1995. Fertilization of strawberries in Florida. Fla. Coop. Ext. Serv. Circ. 1141.
Hochmuth, G. J., and E. E. Albregts. 2003 (reviewed). Fertilization of strawberries in Florida. Fla. Coop. Ext. Serv. CIR1141.
Hochmuth, G. J., E. E. Albregts, C. C. Chandler, J. Cornell, and J. Harrison. 1996. Nitrogen fertigation requirements of drip-irrigated strawberries. J. Amer. Soc. Hort. Sci. 121(4):660–665.
Hochmuth, G., D. Cantliffe, C. Chandler, C. Stanley, E. Bish, E. Waldo, D. Legard, and J. Duval. 2006a. Containerized strawberry transplants reduce establishment-period water use and enhance early growth and flowering compared with bare-root plants. HortTechnology 16:46–54.
Hochmuth, G., D. Cantliffe, C. Chandler, C. Stanley, E. Bish, E. Waldo, D. Legard, and J. Duval. 2006b. Fruiting responses and economics of containerized and bare-root strawberry transplants established with different irrigation methods. HortTechnology 16:205–210.
Hochmuth, G., and K. Cordasco. 1999 (reviewed in 2009). Summary of N, P, and K research with strawberry in Florida. Fla. Coop. Ext. Serv. Fact Sheet HS 752.29.
Hochmuth, G. J., and E. A. Hanlon. 1989. Commercial vegetable crop nutrient requirements. Fla. Coop. Ext. Serv. Circ. 806.
Hochmuth, G. J., and E. A. Hanlon. 1995a. IFAS standardized fertilization recommendations for vegetable crops. Fla. Coop. Ext. Serv. Circ. 1152.
Hochmuth, G. J., and E. A. Hanlon. 1995b. Commercial vegetable crop nutrient requirements in Florida. Fla. Coop. Ext. Serv. SP 177.
Hochmuth. G. J. and E. A. Hanlon. 2000. IFAS standardized fertilization recommendations for vegetable crops. Circular 1152. University of Florida, Institute of Food and Agricultural Sciences, Gainesville, FL.
Hochmuth, G. J., and E. A. Hanlon. 2009. Calculating recommended fertilizer rates for vegetable crops grown in raised-bed mulched cultural systems. Fla. Coop. Extension Serv. Circ. SL 303. https://edis.ifas.ufl.edu/pdffiles/ss/ss51600.pdf.
Hochmuth, G. J., and E. A. Hanlon. 2010a. Commercial vegetable fertilization principles. Fla. Coop. Extension Serv. Cir. SL 319. https://edis.ifas.ufl.edu/pdffiles/cv/cv00900.pdf.
Hochmuth, G. J., and E. A. Hanlon. 2010b. Principles of sound fertilizer recommendations. Fla. Coop. Extension Serv. Cir. SL 315.
Hochmuth, G. J., D. Maynard, C. Vavrina, E. A. Hanlon, and E. Simonne. 2009. Plant Tissue Analysis and Interpretation for Vegetable Crops in Florida. 55 p. https://edis.ifas.ufl.edu/publication/ep081.
Hochmuth, G. J., and A. G. Smajstrla. 1997. Fertilizer application and management for micro (drip)-irrigated vegetables in Florida. Fla. Coop. Ext. Serv. Circ. 1181.
Kidder, G., E. A. Hanlon, and G. J. Hochmuth. 1989. IFAS standardized fertilization recommendations for vegetable crops. Fla. Coop. Ext. Serv. Spec. Ser. SS-SOS-907.
Locascio, S. J. 1964. Effects of fertilizer placement, organic nitrogen and time of mulching on strawberry yield. Proc. Fla. State Hort. Soc. 77:194–198.
Locascio, S. J. 1971. Strawberry yield and soil nutrient levels as influenced by plant population, fertilizer rate and bed shape. Proc. Fla. State Hort. Soc. 84:160–162.
Locascio, S. J., J. P. Gilreath, S. Olson, C. M. Hutchinson, and C. A. Chase. 2005. Red and black mulch color affects production of Florida strawberries. HortScience 40(1):69–71.
Locascio, S. J., D. S. Harrison, and V. F. Nettles. 1967. Sprinkler irrigation of strawberries for freeze protection.
Locascio, S. J., and F. G. Martin. 1985. Nitrogen source and application timing for trickle irrigated strawberries. J. Amer. Soc. Hort. Sci. 110(6): 820–823.
Locascio, S. J., and J. Mostella Myers. 1975. Trickle irrigation and fertilization method for strawberries. Proc. Fla. State Hort. Soc. 88:186–189.
Locascio, S. J., J. M. Myers, and F. G. Martin. 1977. Frequency and rate of fertilization with trickle irrigation for strawberries. J. Amer. Soc. Hort. Sci. 102(4):456–458.
Locascio, S. J., and G. K. Saxena. 1967. Effects of potassium source and rate and nitrogen rate on strawberry tissue composition and fruit yield. Proc. Fla. State Hort. Soc. 80:173–176.
Locascio, S. J., and B. D. Thompson. 1960. Strawberry yield and the soil nutrient levels as affected by fertilizer rate, type of mulch and time of application. Proc. Fla. State Hort. Soc. 73:172–179.
Mansell, R. S., S. J. Locascio, H. M. Selim, L. C. Hammond, and J. M. Myers. 1977. Nutrient and water distributions in sandy soil during growth of trickle-irrigated strawberries. Soil Crop Sci. Soc. Fla. Proc. 36:106–113.
Montelaro, James. 1978. Commercial vegetable fertilization guide. Fla. Coop. Ext. Serv. Circ. 225-B.
Myers, J. M., and S. J. Locascio. 1972. Efficiency of irrigation methods for strawberries. Proc. Fla. State Hort. Soc. 85:114–117.
Mylavarapu, R. S. 2009. UF/IFAS Extension Soil Testing Laboratory (ESTL) Analytical Procedures and Training Manual Circular 1248, 19 p. https://doi.org/10.32473/edis-ss312-2009.
Olson, S. M., and E. Simonne (eds.). 2010. The Vegetable Production Handbook. Fla. Coop. Extension Serv. https://edis.ifas.ufl.edu/entity/topic/vph.
Ozaki, H. Y., and J. R. Iley. 1965. Strawberry fertilizer trials on sandy soils of the Lower East Coast. Soil Crop Sci. Soc. Fla. Proc. 25:344–351.
Peres, N. A., J. F. Price, W. M. Stall, C. K. Chandler, S. M. Olson, T. G. Taylor, S. A. Smith, E. H. Simonne, and B. M. Santos. 2009. Chapter 20. Strawberry Production in Florida. In: Vegetable Production Handbook. pp. 259–277.
Ramirez-Sanchez, M., B. M. Santos, C. K. Stanley, and S. A. Sargent. 2009. Influence of irrigation programs on strawberry cultivars. Proc. Fla. State Hort. Soc. 122:206–208.
Santos, B. M. 2010. Effects of preplant nitrogen and sulfur fertilizer sources on strawberry. HortTechnology 20:193–196.
Santos, B. M., and C. K. Chandler. 2009. Influence of nitrogen fertilization rates on the performance of strawberry cultivars. International Journal of Fruit Science 9:126–135.
Santos, B. M., J. R. Duval, E. A. Golden, E. H. Simonne, and A. J. Whidden. 2006. Influence of drip irrigation and nitrogen rates on strawberry cultivars. Proc. Fla. State Hort. Soc. 119:221–223.
Santos, B. M., and M. Ramirez-Sanchez. 2009. Effects of preplant nitrogen fertilizer sources on strawberry. Proc. Fla. State Hort. Soc. 122:240–242.
Saxena, G. K., and S. J. Locascio. 1968. Fruit Quality of Fresh Strawberries as Influenced by Nitrogen and Potassium Nutrition. Proc. Amer. Soc. Hort. Sci. 92:354–362.
Schepers, J. S., K. D. Frank, and C. Bourg. 1986. Effect of Yield Goal and Residual Nitrogen Considerations on Nitrogen Fertilizer Recommendations for Irrigated Maize in Nebraska. J. Fertilizer Issues 3:133–139.
Simonne, E. H., J. R. Duval, and E. Golden. 2001. Interaction between Nitrogen Rates and Cultivar on the Yield of Strawberry. Proc. Fla. State Hort. Soc. 114:315–317.
Simonne, E. H., and G. J. Hochmuth. 2010. Chapter 2. Soil and Fertilizer Management for Vegetable Production in Florida. Vegetable Production Handbook. HS711: 3–15. https://edis.ifas.ufl.edu/publication/cv101.
Simonne, E. H., and M. D. Dukes. 2009. Chapter 3: Principles and Practices of Irrigation Management for Vegetables. EDIS: AE260, Florida Vegetable Handbook: 17–23. https://edis.ifas.ufl.edu/publication/cv107.
Thompson, B. D. 1957. Strawberry production. Fla. Agr. Exp. Sta. Ann. Report 1957:166.
Thompson, B. D. 1959. Response of strawberries to mulching with plastic. Proc. Fla. State Hort. Soc. 72:179–185.