The purpose of this publication is to summarize the historical Florida research literature on nitrogen, phosphorus, and potassium fertilization of pepper. This publication documents the previous written literature, some of which now appears only in updated modern electronic format. All publications, including the older paper-format papers, will be placed in a PDF format on the website https://bmp.ifas.ufl.edu. Research publications have provided the scientific basis for the Florida Extension pepper fertilization recommendations that are the basis for Best Management Practices (BMPs) that address water quality protection as well as profitable pepper production.
This publication is an updated version of a previous research literature review by Hochmuth and Cordasco (1999; reviewed 2009) covering research through 1996. An older research review on pepper fertilization was made by Hochmuth and Hanlon (1989b). This new review includes research reports conducted since 1996 and expands on the role of irrigation in managing fertilizer for crop production efficiency and environmental protection. The audience for this publication includes educators, such as Extension specialists and agents, commercial vegetable producers, consultants, and governmental agencies involved in environmental regulations.
In 2007, the state of Florida ranked number one nationally for bell pepper production with 39.1% of the nation's overall bell pepper production. During the 2007–2008 production season, 18,800 acres were harvested in Florida resulting in 18,277,800 cartons (28 lb/carton) of bell peppers valued at $267,411,000. The largest volume of Florida pepper production came from the Palm Beach County area that has been responsible for 88 and 89% of the state's bell pepper production for the surveyed years of 2002 and 2007, respectively (Fla. Dept. of Agr. and Cons. Serv. 2009).
More than 60 years of pepper fertilization research has been conducted in Florida. During this time, many changes have occurred in pepper production practices, including changes in cultivars and the introduction of new cultural systems such as polyethylene mulch and drip irrigation. Pepper fertilizer management recommendations have changed throughout the years in keeping with new developments in research (Montelaro 1978; Hochmuth et al. 1988; Hochmuth 1996; Hochmuth and Hanlon 2000; Kidder et al. 1989; Olson et al. 2010; Simonne and Hochmuth 2010). The most current recommendations for nutrient management in pepper production are presented in the Commercial Vegetable Production Handbook for Florida (Olson and Simonne 2010). The early research focused largely on yield and fruit quality (size and shape) in response to fertilization. Since the early research was conducted, and since the first review of the literature in 1989, there have been increasingly strong interests in the incorporation of more environmental impact (water quality) studies into the fertilizer research. The state (Fla. Dept. of Agr. and Cons. Serv. 2005) has been formalizing BMPs and encouraging growers to implement BMPs. Part of the definition of a BMP includes consideration of both economics and environmental components and that a BMP embodies the best available science.
Fertilizer recommendations not only contain the recommended rate of fertilizer but also the management strategies for getting the most out of the fertilizer investment while protecting the environment. These principles for fertilization of vegetables are summarized by Hochmuth and Hanlon (2010a). Rate is only a part of the modern fertilizer recommendation. Sound fertilizer recommendations also consider fertilizer materials, placement, and timing, among other aspects (Hochmuth and Hanlon 2010b).
The fertilization recommendation addresses commercial yield and quality, the economics of crop production, and protection of the environment. Equally important is to have a mechanism available to the grower to adjust fertilization practices during the season due to leaching rains and extension of the growing season for additional harvests when market conditions are favorable. To address all of these concerns, UF/IFAS vegetable recommendations are given as a single target fertilizer rate that is projected to be sufficient for meeting the crop fertilizer needs for most growing seasons. This single target fertilizer rate is a recommended starting point and has been used historically in all vegetable fertilizer recommendations in Florida, as well as around the country. The target recommendation approach with footnotes was used by Montelaro (1978) and confirmed by Hochmuth and Hanlon (1995; 2000). The target value was derived from numerous fertilizer studies and represents a reasonable fertilizer rate that reflects the average maximum crop responses from all of the fertilizer research, not the extremes in responses. However the recommendation process recognizes some growing seasons are different due to more leaching rains or increases in crop value that can lead to prolonged harvest windows. Hence the target fertilizer amount is accompanied by a series of footnotes that explain the addition of supplemental fertilizer during the growing season, which includes addressing leaching rains and additional harvests near the end of the season. It is logical to select a single target rate based on research that avoids excessive fertilizer applications that often reduce crop nutrient efficiency and increase the potential for environmental degradation.
Data Summary Method
Evaluation of pepper yield responses to variable rates of applied fertilizer required a standardized method of summarizing statewide yields that were expressed variably as bushels, cartons, boxes, or tons/acre. In addition, vegetable yields can vary depending on production season, cultivar, weather conditions, irrigation management level, and location in the state. Relative yield (RY), a calculated percentage, was chosen as the unit to express pepper yield responses to fertilization. Relative yield is an accepted scientific method for summarizing and presenting data across wide sources and reports (Brown 1987).The highest yield for each fertilizer experiment was designated as 100%, and other yields were expressed as a percentage of the highest yield. The actual yield in 28-lb cartons (one and one-ninth bushel per carton) was presented for the treatment corresponding to 100% RY. The RYs were plotted against rates of nutrient to visually determine how pepper yields responded to fertilizer in Florida. The RY presentation allowed data from a variety of experiments to be included in the summary of yield responses to fertilization. For most studies, RYs of 90 to 100% were not significantly different from each other.
Fertilizer rates are expressed on a per-acre basis (amount of fertilizer used on a crop growing in a 43,560 sq ft area). Changes in bed spacing lead to changes needed in applied fertilizer amounts per acre but not in rates in the actual bed. For example, to maintain the same amount of fertilizer in the bed of a crop on 6-foot-bed-spacing as in the bed of a 4-foot-bed-spacing crop requires an increase by a factor of 1.5 in the "per acre" rate of fertilizer for the crop growing in beds spaced 4 foot on center. The important aspect is to have the same amount of fertilizer per linear bed foot. This linear-bed-foot system is used by the University of Florida Extension Soil Testing Laboratory to express fertilizer rates. The concept was originally explained by Hanlon and Hochmuth (1989) and updated by Hochmuth and Hanlon (2009). Fertilizer-rate expressions used in this summary and its figures are those rates presented by the various authors in their research papers. Most authors express rates on a per-acre basis, irrespective of variations in bed spacings among reports or experiments. Authors of a few reports choose to use the linear-bed-foot system to standardize fertilizer-rate expressions across experiments and planting patterns. We will attempt to specify planting patterns and fertilizer rates for each experiment as far as we can determine from each report.
In his book, C. Black (1992) summarized the advantages and disadvantages of the RY approach. There are valid statistical concerns about RY, but he concluded that, when applied properly and cautiously, the RY approach can be useful in displaying general relationships. Black demonstrated examples in his book where RY yield was helpful and where it was not. We chose the RY approach because we wanted to display the historical data without making biased decisions about what to include and what not to include in the presentation. Plotting absolute yield data in original units obviously would result in a scattering of data rendering any general interpretation impossible. Black (1992) points out that decisions can always be improved with further research, but the current data are the best we have at the time.
Sometimes the argument is made that growers have expectations of greater yields than those obtained in research projects. However, realistic, regularly obtainable yields are often less when compared with expected yields or "yield goals." Research on this subject has documented that 20% of growers actually reached their yield goals, and only 50% reached 80% of their yield goals (Schepers et al. 1986). Therefore growers rarely achieved their stated yield goals, which means recommended fertilizer rates should not be set upon yield goals but rather on realistic yields documented by research. This practice of using yield goals to set fertilizer rates has not been recommended in Florida, so the effect of overfertilization has been avoided by grounding expectations in measurement of observed yields. In effect, the current Florida fertilizer recommendation includes a component that addresses concern expressed by growers about yield expectation versus actual production capability. Scientists have conducted many replicated demonstration studies in growers' fields with typical commercial production practices, and those studies are included in this review where possible. Excessive fertilizer was justified in times past when fertilizer was viewed as inexpensive insurance against yield loss. Research with numerous crops has shown that nutrient use efficiency declines as nutrient rate, especially N, increases. Even given the best of production systems, N use efficiency rarely exceeds 70% of the applied N. Concepts and practices for managing nutrients in vegetable production were summarized by Hochmuth (1992a; 1992b; 2000).
Further, it has been suggested that the yields in older studies were much lower than yields obtained today. However there are older research reports within the last two decades where yields were as high as yields achieved today. While fertilizer rate is reported in pounds per acre (lb/acre), the reader should keep in mind that the bed system and plant population used in each field may vary and require adjustments to the fertilizer rate. To address this issue, the linear bed foot concept can be used to convert among the various bed designs with and without spray rows or ditches (Hanlon and Hochmuth 1989; Hochmuth and Hanlon 2009). Additional cultural practices for commercial tomato production can be found in Chapter 2 of the Vegetable Production Guide (Simonne and Hochmuth 2010).
Keeping nutrients in the root zone and available for pepper plant uptake requires proper irrigation management. Due to the critical nature of irrigation and drainage management with respect to nutrient uptake by the plant and possible loss of nutrients to the environment, information is regularly updated (Simonne et al. 2010).
Another useful tool for measuring nutrients, and thus contributing to appropriate management of fertilizer, is plant tissue and/or plant sap sampling. For interpretation of plant and petiole sap values, see Hochmuth (1994a; 1994b) and Hochmuth et al. (1991b; 2009).
Research with Nitrogen Fertilizer
Many studies have been conducted with N fertilization of pepper. Some of the earlier studies evaluated rates of mixed N-P-K materials with subsurface irrigation (also called "seepage" irrigation) and are summarized in Figure 1. Pepper responses to rates of mixed fertilizers are considered here to be largely responses to N. In Northern Florida, early studies were conducted with sprinkler irrigation (Figure 2). Most of the studies with N rates for pepper in Southern Florida used subsurface irrigation (Figure 3). Drip irrigation (Figure 4) was used on most of the more recent studies in Northern Florida.
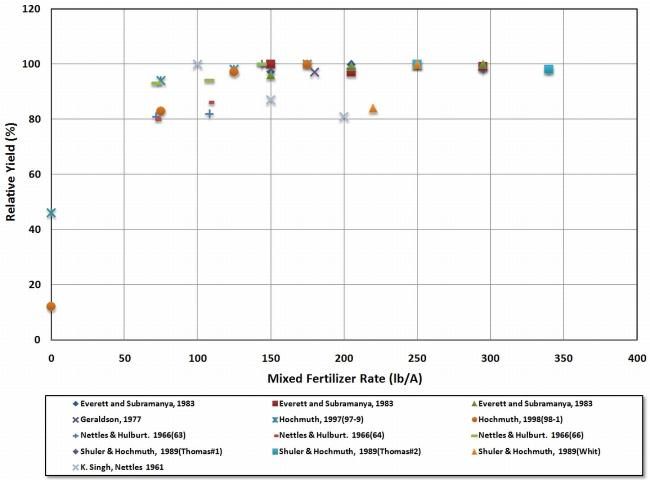
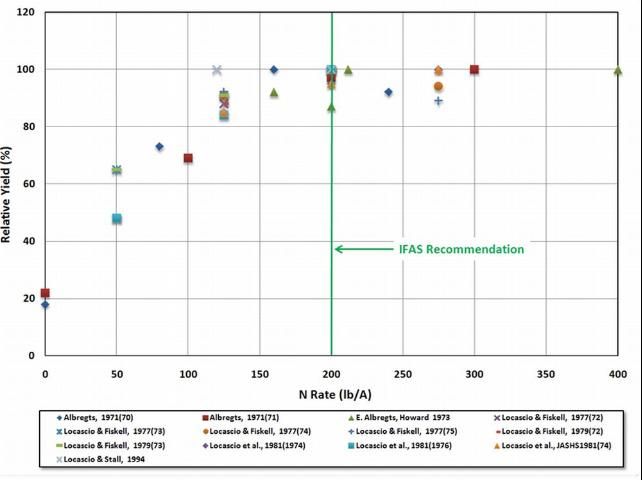
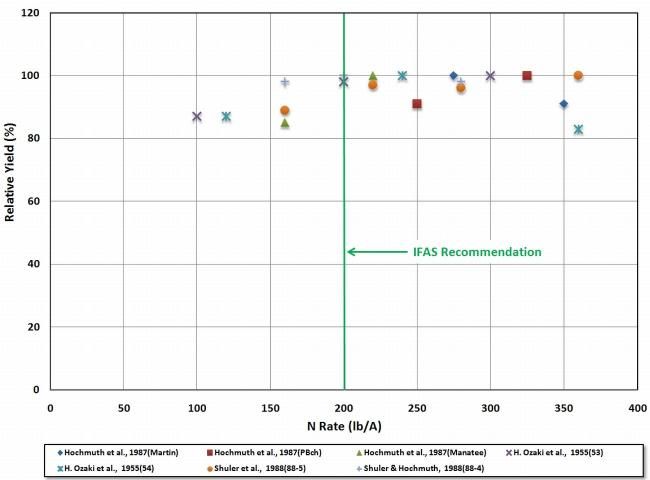
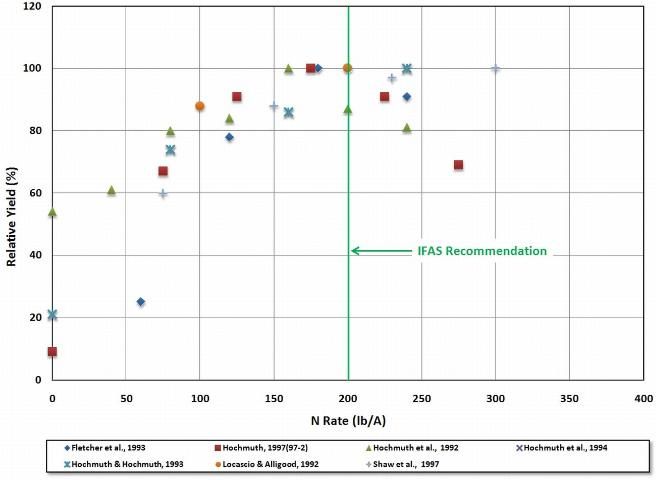
Nitrogen
Sprinkler Irrigation
The effects of increasing N rates with mixed fertilizers, defloration, and defruiting treatments on growth and yield of overhead-irrigated, unmulched pepper were evaluated in two experiments (Singh and Nettles 1961). In a 1960 experiment, 40-180-66 lb/acre N-P2O5-K2O was banded to each side of the row and beneath the soil at transplanting and again 30 days later. Supplements of sodium nitrate were side-dressed at 30-day intervals to achieve total N rates of 100, 150, or 200 lb/acre. In 1961, 40-180-66 lb/acre N-P2O5-K2O was applied as before, but at transplanting only. Side-dressings of sodium nitrate were applied for total N rates of 50, 100, or 150 lb/acre. Rows (one per bed) were spaced 34 inches apart.
Increasing N from 100 to 200 lb/acre decreased marketable yields in 1960 from 100% RY (300 cartons/acre) to 81% RY, respectively, with yields averaged using defloration and defruiting treatments. Researchers cited increased rainfall and N leaching losses as the basis for the 1960 yield decline. Yield was optimized with 150 lb/acre N (RY=100%, 314 cartons/acre) (Figure 1). Blossom-end rot increased with increased N. These yields are considerably lower than yields today due to better varieties and plastic mulch now used by growers.
Using 'Yolo Wonder' pepper, Nettles and Hulbert (1966) measured yield response to three rates of a mixed fertilizer (6-8-8 N-P2O5-K2O), resulting in nitrogen at 72, 108, or 144 lbs per acre. There was no difference in yield with the two lower rates of fertilizer, and yield with both was less than with 144 lb per acre N (100 % RY) in all three years of study (Figure 1). Yields (100% RY) ranged from 250 to 420 cartons per acre.
Sprinkler irrigation was used on a Dover, Florida, paper-mulched pepper field where varying rates of N fertilizer were applied in the 1970 and 1971 seasons (Albregts 1971). Initial soil nitrate-N concentrations were chemically determined from water extracts taken at field moisture capacity. The results were 337 and 101 parts per million (ppm) nitrate-N in 1970 and 1971, respectively. Soil was Scranton fine sand with 4% organic matter. One-third of the fertilizer was broadcast and the remainder banded at the bed center. Bed centers were spaced 56 inches apart. Nitrogen rates were 0, 80, 160, or 240 lb/acre and resulted in quadratic RY responses of 18, 73, 100 (908 cartons/acre) and 92% for each respective N rate. Second-year applications of 0, 100, 200, and 300 lb/acre N resulted in quadratic RY responses of 22, 69, 97, and 100% (725 cartons/acre) for each respective N rate. Yield was best with 160 lb/acre N in the first year on the soil with a high starting level of soil nitrate-N. In the second year, on a soil with a lower starting nitrate-N level, yield was slightly improved with 300 lb/acre N compared with the 200 lb/acre N treatment (Figure 2).
Research with biodegradable polyethylene-coated paper mulch and two different N rates in two years was conducted on okra and pepper crops in Dover (Albregts and Howard 1973). Bed spacing was not indicated in this study. In spring 1971, RY responses to 160 and 212 lb/acre N were 92 and 100% (556 28-lb cartons/acre), respectively. These treatment means were not significantly different. In spring 1972, RY responses to 200 and 400 lb/acre N were 87 and 100% (547 28-lb cartons/acre), respectively, and significant at the 5% level. High concentrations of residual NO3-N, 685 and 395 ppm, were measured from saturated water extracts from mulched beds before the last unmulched fertilization event each year. Average yields of mulched pepper were double the average yields of unmulched peppers (507 and 258 28-lb cartons/acre, respectively), and average fruit weight was 15% higher with mulched than with unmulched pepper.
In experiments encompassing four seasons at Gainesville, Florida, 1972–1975, yield responses to N rates were measured. Mulch treatments included black polyethylene mulch and bare ground; and fertilizer placement varied among broadcast, band, and multiple-band applications (Locascio and Fiskell 1977). Plots were 6 x 25 feet with two rows of plants on each bed. The largest yield response occurred between 50 and 125 lb/acre N in 1973. Quadratic yield responses resulted, with yields leveling off between 125 lb/acre N (91% RY) and 200 lb/acre N (100% RY, 534 cartons/acre). An interaction, significant at the 1% level, occurred between fertilizer placement and rate in 1972. Linear yield responses resulted with mulched/broadcast-fertilized pepper. Quadratic yield responses resulted with unmulched/broadcast-fertilized pepper. Linear yield responses occurred when unmulched pepper received N in a single, banded-fertilizer application, and yields were not affected when fertilizer was applied in three broadcast applications to unmulched pepper. Overall yields were progressively higher in 1974 and 1975 compared to 1973 yields. Yield responses were not different in 1974, producing an average 663 cartons/acre with N rates from 125 to 275 lb/acre. In 1975, yields responded quadratically, significant at the 10% level, reaching optimum 100% RY (890 cartons/acre) with 200 lb/acre N and declining to 89% RY with 275 lb/acre N. Results from three seasons suggested that the N fertilizer requirement of pepper was about 200 to 230 lb/acre. Leaf N concentrations of about 4% were associated with highest yields.
A factorial study of 12 N sources, three N rates, applied in banded or broadcast applications with three forms of mulch was conducted near Gainesville for two seasons, 1972 and 1973 (Locascio and Fiskell 1979). Plots were 6 x 25 feet, and fertilizer was applied in a five-foot area across the bed and incorporated. Responses to N rate were quadratic in both years. Maximum yields were obtained in most treatments with 200 lb/acre N. There was an interaction between N source and rate in 1972, but not in 1973. The authors cited lower yields from plants fertilized with 200 lb/acre N of soluble urea or sulfur-coated urea (SCU) when both were broadcast and unmulched. Yields with all other N sources increased linearly through the 200 lb/acre N rate. Considering both years, highest yields were produced with broadcast SCUs (faster dissolution rates: 30, 35, and 44%/week), urea broadcast in three applications, and urea broadcast and covered with mulch. Lowest yields were obtained with banded urea, urea with 10-inch-wide polyethylene strip mulch, and broadcast urea-formaldehyde. The authors cautioned against band application of urea due to soluble-salt injury with increased N rates.
Evaluation of soil N in this experiment indicated N retention was equal or better with polyethylene mulch covering broadcast or banded fertilizer compared to unmulched multiple fertilizer applications/season or controlled-release (CR) N forms. On unmulched soil, CR-SCU and isobutylidene diurea (IBDU) provided assurance of adequate soil N. Relative yield responses to N rates of 50, 125, and 200 lb/acre were 48, 88, and 100% (592 cartons/acre), respectively in 1972, and 65, 90, and 100% (534 cartons/acre), respectively in 1973. The results of additional Gainesville experiments conducted in 1974 and 1976 (Locascio et al. 1981) closely paralleled the previous study. Plots were 6 x 25 feet and were planted in double rows on the bed. A significant interaction (5% level) occurred between N rate, N source, and placement in 1974. Soluble-salt injury likely caused a sharp decline in marketable yields with broadcast NH4NO3 between N rates of 200 (1,000 28-lb cartons/acre) and 275 lb/acre (571 28-lb cartons/acre). Lower N rates, 125 to 200 lb/acre, reduced marketable yields with banded NH4NO3 (715 to 534 28-lb cartons/acre) and urea-formaldehyde (607 to 357 28-lb cartons/acre). In 1976, a significant interaction (5% level) occurred between N rate, N source, and mulch. Mulched plants with (NH4)2SO4, urea, and SCU-2 (26.8% dissolution in seven days) reached peak yields with 125 lb/acre N; and unmulched plants treated with (NH4)2SO4, urea, and NH4NO3 reached peak yields with 125 lb/acre N. In this season, fertilizers were broadcast—once at preplant with the mulched treatments and also in three equal applications with the unmulched treatments. The above research suggested that peppers with proper water management practices that minimized leaching were unlikely to respond to soluble N sources in one application as well as when grown with mulch at rates more than 125 lb/acre N. Higher soluble-N rates risked soluble-salt injury.
Peppers grown with controlled-release N sources responded to N rates up to 275 lb/acre supplied from IBDU, SCU (32% dissolution in seven days), and urea-formaldehyde, when broadcast, incorporated, and mulched but did not respond when N was banded and mulched (1974). Pepper yields, with and without mulch in spring 1976, were greatest with CR-N sources—IBDU and SCU-1 and -3 (37 and 32% dissolution in 7 days) with the 200 lb/acre N rate; the 275 lb/acre N rate was not tested. Of the soluble N sources, NH4NO3 alone resulted in peak yields with 200 lb/acre N when mulched. With CR sources, more efficient utilization of N may have resulted in yield increases with N rates above 125 lb/acre. A long six-harvest season likely resulted in the yield response to 275 lb/acre N CR-N.
Mulch use increased pepper yields 25% compared to yields from unmulched trials in 1976 (significant at 1% level). Yield results were averaged using all three N rates, 50, 125, or 200 lb/acre, and were greater with all N sources for mulched pepper compared to unmulched. Soil N retention with the mulched planting was 70% greater 3 and 15 weeks after fertilization due to reduced leaching of soluble nutrients with polyethylene mulch in overhead-irrigated fields.
In related studies, researchers showed that the 1:2 soil to water extract used for soil testing could be used for soil NO3, NH4, and K analyses (Fiskell et al. 1978). These analyses can be used to interpret soil nutrient status. Nitrogen source has a smaller effect on soil test values than N rate. The authors concluded that soil testing during the growing season could be used to track changes in soil nutrient status and guide fertilizer applications.
An overhead-irrigated and mulched pepper crop near Gainesville responded equally to N rates of 120 or 200 lb/acre N (Locascio and Stall 1994). The soil, previously uncultivated, had 1.7% organic matter, which was credited with equalizing the response to N. Nitrogen sources IBDU, 35% of total N, and NH4NO3, 65% of total N, were blended, broadcast, incorporated, and mulched with black polyethylene. Yield with 200 lb/acre N was 1.55 lb/plant (100% RY) and 1.46 lb/plant (94% RY).
Subsurface Irrigation
Three rates of N and three rates of K were evaluated in a factorial study on Davie fine sand near Fort Lauderdale (Ozaki et al. 1955). The study occurred during the 1953–1954 and 1954–1955 growing seasons on subsurface-irrigated fields with a constant two-foot (below-bed-surface) water table. Beds were planted in double rows, but the bed spacing was not indicated in this study. Pepper yields produced with N rates of 100, 200, and 300 lb/acre were 87, 98, and 100% RY (757 28-lb cartons/acre), respectively. Yields with between 200 and 300 lb/acre N treatments were not different. Frequent rainfall was cited for the 1953–1954 season, and leaching was suspected. In the second season, yield responses were also not different between 120 and 240 lb/acre N (87 and 100% RY [612 cartons/acre], respectively). Through use of regression analysis, researchers indicated that RY fell to 83% with 360 lb/acre N. Injured fruits, white tissue drying to brown, were present on plants with all treatments in that year. Numbers of injured fruits were greatest with the highest N and K rates. Additional research showed maximum pepper response to N was between 270 and 350 lb/ acre (Ozaki and Hamilton 1954; Ozaki et al. 1957). Increasing the biweekly applications of 10-10-10
(N-P2O5-K2O) fertilizer from 300 to 350 lb/acre did not significantly increase yield for variety ('World Beater'), but yield of 'Florida Giant' was greater with 350 lb/acre (Ozaki 1961). Associated increases in fruit injury with higher N rates were noted in earlier research by Singh and Nettles (1961), supporting 200 lb/acre N as the optimum N rate for overhead-irrigated and nonmulched pepper. In an early study on unmulched pepper in 1961, two levels of 10-10-10 fertilizer (300 and 350 lb/acre) were applied in biweekly side dressings. There was an interaction between fertilizer rate and variety in which increasing the fertilizer rate increased yields of 'Florida Giant' but decreased the yields of 'World Beater.'
Typical commercial pepper yields in the 1970s were reported to be approximately 550 bu/acre (Geraldson 1977). Some growers could obtain greater yields with more intensive production practices, such as mulch, new varieties, attention to nutrition, etc. Two rates of mixed fertilizer were compared in studies involving other factors like fertilizer band placement and variety (Geraldson 1977). Yield with 1,000 lb/acre of 18-0-25 N-P2O5-K2O fertilizer resulted in 1,244 cartons/acre (97% RY) compared with 1,276 cartons/acre (100% RY) with 2,000 lb/acre of the same fertilizer banded on the bed surface.
A subsurface-irrigated, mulched study in Immokalee established CR fertilizer as a useful starter fertilizer to prevent seedling burn and to hold nutrients during wet seasons (Everett 1977). Starter fertilizer sources alone were tested in fall 1976, including Osmocote (15-5-21 [N-P2O5-K2O]) and a 50% Osmocote blend (10-12-11 [N-P2O5-K2O]). CR-N sources were banded two inches below the row, two inches below and to the side of the row, or in a four-inch band in the plant row. Nitrogen rates were 0, 30, 60, or 90 lb/acre. A soluble fertilizer, 18-0-25 (N-P2O5-K2O), broadcast two feet across the row at 36 lb/acre N, was also tested. At one and two weeks after transplanting, soluble salts from soluble fertilizer sources had leached from the upper two inches of soil to the same soluble-salt concentrations as unfertilized soil. Five weeks after transplanting, salt concentrations in the soil treated with the soluble fertilizer source were 1.7 times higher than the Osmocote-treated soils. Both the straight Osmocote and the blended form resulted in less leaching and reduced surface-salt accumulation compared to the soluble N source (KNO3 and NH4NO3).
In the spring 1977 season, the above starter fertilizers (Osmocote, Osmocote blend, and the soluble source) were tested at rates of 0, 30, or 60 lb/acre N. Additional soluble fertilizer at 180 lb/acre N was banded on both sides of the starter-fertilized beds. A higher N rate was applied on the zero-starter-fertilizer treatment. Relative yield responses with 0, 30, or 60 lb/acre (starter) N were 83, 100 (1,147 cartons/acre), and 97%, respectively. Controlled-release starter fertilizer at 30 lb/acre N proved a worthwhile starter N source at this early stage of pepper growth.
Pepper fruit yield was not affected by simultaneous changes in both N and K rates (mixed N/K fertilizers) during three Immokalee trial seasons: spring 1982, fall 1982, and fall 1983 (Everett and Subramanya 1983). Relative yields ranged from 96 to 100% (1,459; 1,311; and 1,300 cartons/acre, in the three seasons, respectively) resulted from 150 lb/acre N and 210 lb/acre K2O. Yield responses with 205 lb/acre N (290 lb/acre K2O) and 295 lb N (415 lb/acre K2O) did not vary from yields with the lower N and K rate in this mixed N and K study. These fruit yields are similar to yields obtained in studies during the 1990s, showing that older production practices produced yields similar to those expected today. Spring plantings were mulched with black polyethylene and fall plantings with white. Nitrogen at 25 lb/acre was applied preplant to a low bed then covered with four inches of soil, with additional N from KNO3 and NH4NO3 (70% NO3-N, 30% NH4-N) applied in two bed-surface shoulder bands. Despite a long harvest season and high rainfall in the three trial periods, N and K programs supplying 150 lb/acre N and 210 lb/acre K2O proved sufficient for optimum yields.
Researchers at subsurface-irrigated sites in Martin, Manatee, and Palm Beach counties (Hochmuth et al. 1987b) tested two N rates each, using SCU at Martin County and mixed fertilizer sources at the remaining counties. A fourth site in Hillsborough County was used, but yields were very low due to bacterial leaf spot and pepper weevil damage. Beds were spaced 6 feet on center. At Martin County, 120 lb/acre N (SCU) was broadcasted and incorporated preplant, and additional N was banded outside of the plant rows for a total of 275 or 350 lb/acre N, the grower rate. The higher yield, 100% RY (1,263 cartons/acre), occurred with 275 lb/acre N (significant difference). Yield dropped 10% with the 350 lb/acre rate.
At Manatee and Palm Beach counties, 24 and 25 lb/acre N, respectively, of mixed fertilizer were incorporated in the bed before planting. The remaining fertilizer was banded in grooves on the bed surface for total N rates of 160 and 220 lb/acre at Manatee County and 250 and 325 lb/acre N at Palm Beach County. Relative yields at Manatee County were 85 and 100% (1,355 cartons/acre) with each N rate (difference was not significant). Overall, yields in the Palm Beach County study were 40% lower than yields achieved at either Martin or Manatee counties, resulting in 100% RY (810 cartons/acre) with 325 lb/acre N (difference not significant). Researchers cited a drastic lowering of the water table at the Palm Beach trial during weeks five through eight. Heightened end-of-season soluble-salt levels in the soil of 1,300–1,700 ppm (with 250 and 325 lb/acre N, respectively) were attributed to this dry period leading to subsequent soluble-salt injury and reduction in yield. Soil at all sites had comparable prefertilization soil soluble-salt levels of 100 to 200 ppm.
Total N rates of 160, 220, 280, or 360 lb/acre were compared for effects on pepper production at a Jupiter spring demonstration planting (Shuler and Hochmuth 1988b). Experimental plots were 6 x 20 feet and planted with two rows per bed. Sulfur-coated urea was broadcast at 120 lb/acre N, with the remainder of each rate applied in a band on the bed surface or two inches below the surface. Relative yields ranged from 89, 97, 96, and 100% (1,380 cartons/acre) with each N rate noted above. In a winter demonstration study on a commercial pepper farm in Boynton Beach (Shuler and Hochmuth 1988a), peppers planted on 6 x 20-foot plots did not respond to increased N rates greater than 160 lb/acre (98% RY), the recommended N rate in 1989 (Hochmuth and Hanlon 1989a). In this study, all fertilizer treatments were applied in two side bands and one center band. Additional N to 220 lb/acre increased RY 2% (1,535 cartons/acre, 100% RY). Nitrogen applications at twice the recommended amount (360 lb/acre applied to the grower's field) provided no yield benefit (97% RY) compared to the 160 or 220 lb/acre rates.
A subirrigated system (Fully Enclosed Subirrigation [FES]) pepper study in Bradenton (Stanley and Clark 1993) was designed to determine how much N leached out of the root zone, how much N was utilized by the crop, and how much water was utilized by the crop in a four-month growth cycle. The FES system uses buried microirrigation tubing to convey water to the field to maintain a targeted water table. FES has been shown to result in a savings of 30 to 40% compared to open-ditch conveyance for subsurface (seepage) irrigation. Units were constructed of four separate plastic barrels linked by valves to one sump. A float switch controlled the irrigation and drainage to maintain water levels at 18 to 20 inches for two plants per subunit (eight per lysimeter). Soil within the containers was leached to minimal N concentrations, and KNO3 + NH4NO3 were applied at 300 lb/acre N. Fertilizer was placed eight inches from the plants in 12-inch bands, 1.5 inches deep, and the soil surface was covered with black polyethylene. The total N content was determined from harvested fruit and from vegetative tissue taken at the end of the season. Plant tissue, residual soil, and unaccounted-for N were itemized as follows: 51.9% N (156 lb/acre), 25% (75 lb/acre), and 23% (70 lb/acre), respectively. Plant N uptake confirmed N recommendations at that time of 160–175 lb/acre for pepper (Hochmuth et al. 1988; Hochmuth and Hanlon 1995). The sustained high water table prevented N loss from leaching, but denitrification or loss of N to the atmosphere was suspected for the 23% lost N. N/K mixtures were applied in this study. The study confirmed that a lower water table depth (75 cm) and a lower-than-commercially-practiced rate for N/K fertilizer (200/250 lb/acre) caused no detrimental effect on pepper production when using the FES system.
The advantage of using this system is that it allowed for the integration of water conservation into the planting process. The automated controlling and monitoring of water table levels in the pepper beds eliminated the need for having to constantly flood the beds to ensure adequate water for the crop. The FES system is also potentially beneficial to the environment. Owing to the reduction in the use of water, there is potentially a decrease in the nutrient loading of fertilizers discharged in the environment.
Drip Irrigation
A spring study at the Suwannee Valley Research and Education Center (SVREC, now North Florida Research and Education Center–Suwannee Valley), in Live Oak, Florida, tested NH4NO3 applications of 0, 40, 80, 120, 160, 200, and 240 lb/acre on mulched beds (Hochmuth et al. 1992a). Fertilizer was hand applied and incorporated with a rolling cultivator on beds spaced on 5-foot centers. Total marketable yields increased quadratically in response to increasing N rate. Yield increased from 54% RY with 0 N to 100% RY with 160 lb/acre N (100% RY, 700 cartons/acre), then decreased to 81% RY with 240 lb/acre N.
A 2 x 10 factorial experiment at the UF Horticulture Unit near Gainesville (Locascio and Alligood 1992) evaluated two N rates—100 and 200 lb/acre—and 10 soluble and CR sources of N and K. The N and K sources were broadcast 40% preplant-incorporated [KCl, NH4NO3, and Ca (NO3)2] with 60% drip applied [NH4NO3, Ca (NO3)2, and KCl] once per week for 10 weeks. A second treatment involved broadcasting of KNO3, 100% at preplant. Single beds, 4 by 24 feet, were polyethylene mulched, and peppers were irrigated at 0.75 times pan evaporation from a U.S. Weather Service Class A evaporation pan.
Highest total yield was produced with the preplant KNO3-NH4NO3 treatment, intermediate yields with NH4NO3 and coated KNO3-CR urea treatments, and slightly lower yields with remaining N sources. All yields exceeded state average yields, though higher yielding treatments also led to higher soil NO3-N concentrations later in the season. One hundred percent RY (1,095 cartons/acre) was achieved with the 200 lb/acre N rate, and 88% RY with 100 lb/acre N (significant difference).
Nitrogen fertilization experiments with pepper were conducted in Live Oak at the SVREC on Lakeland fine sand in spring 1992 (Fletcher et al. 1993). The research was conducted to calibrate petiole-sap N testing meters for drip-irrigated and polyethylene-mulched peppers. Nitrogen treatments ranged from 60 to 240 lb/acre NH4NO3-N with treatments in increments of 60 lb/acre. Potassium was applied uniformly at 130 lb/acre K2O (KCl), and no phosphorus (P) was applied due to high soil-test P concentrations. Tilled soils received fertilizer treatments in a 36-inch-wide band that was incorporated and formed into beds on 5-foot centers. The N recommendation for pepper at the time of this study (Kidder et al. 1989) was 160 lb/acre, based on a 6-foot row spacing (190 lb/acre for rows 5 feet apart).
Marketable fruit yields, comprised mostly of U.S. No. 1 and fancy-grade fruits, responded quadratically (5% probability) through 180 lb/acre N (814 cartons/acre, 100% RY). Using the quadratic equation, yield maximized with 190 lb/acre N (810 cartons/acre) as recommended (r2 = 0.76). Leaf-tissue N concentrations less than the critical range occurred with N treatments of 60 lb/acre (early harvest) and with 60 and 120 lb/acre (final harvest). Leaf-tissue N concentrations were greater than critical concentrations with all other N treatments. Petiole-sap concentrations with the optimum 180 lb/acre N treatment were 1,500 ppm (before blossom), 800 ppm (early harvest), and 500 ppm (2nd harvest).
Nitrogen fertigation trials were conducted at the University of Florida Horticulture Research Unit, Gainesville, in 1995 (Shaw et al. 1996). Ammonium nitrate was broadcast and incorporated preplant at 0, 30, 70, and 100% of the total season applied-N rates of 75, 150, 230, and 300 lb/acre N. Potassium magnesium sulfate was also broadcast preplant at 20% of the recommended K2O rate. Based on M-1 soil tests, no P and 130 lb/acre K2O were recommended. Remaining N and K2O (NH4NO3 and KNO3) were fertigated at equal rates for twelve weeks. Two-foot-wide beds were set on 6-foot centers with two plant rows per bed (28,550 plants/acre). Irrigation was applied to keep soil moisture between -5 and -15 cb, as measured by a tensiometer.
Total marketable yield responded quadratically to percent preplant N with yields decreasing with larger proportions of the total N applied preplant. Maximum yields occurred with 30% preplant N. Highest marketable yield response, 100% RY, occurred with 300 lb/acre N, producing 694 cartons/acre. Yield responses were modeled with quadratic and linear-plateau equations. Taking a midpoint value between maximum N rates of each model indicated 215 lb/acre N was sufficient for high yields, near the 230 lb/acre N rate (97% RY). Whole-leaf N concentrations exceeded the sufficiency range throughout the season. Incidence of blossom-end rot increased nine times (3.5 to 36 cartons/acre) with N rates between 75 and 300 lb/acre.
A study to evaluate the interaction of N rate and irrigation amounts was conducted with pepper (Simonne et al. 2006). Factorial combinations of N fertilizer rate (75%, 100%, and 125% of the recommended 200 lb/acre) with irrigation at 33%, 66%, 100%, and 133% of the reference irrigation rate—based on a proposed crop factor that changed based on growth stage—were used. Greatest yields resulted with 115 to 124% of the reference irrigation and with 125% N rate (250 lb/acre N).
In the drip-irrigated experiments summarized above, 0 to 100% of the preplant-applied fertilizers were derived from a soluble or a soluble-plus-CR fertilizer. The portion of fertilizer not applied at preplant was applied through the drip line throughout the season. CR fertilizers offer an opportunity to make one application of fertilizer to the soil at the time of bed formation and apply only water through the drip tubing. An experiment in the fall season of 1996 tested the yield responses of pepper to two Meister (Haifa Chemicals, Haifa, Israel) fertilizers (Hochmuth 1997a). Each fertilizer was applied entirely at preplant in a 10-inch-wide band on the bed surface and incorporated. Beds were spaced on 4-foot centers, and N rates were 75, 125, 175, 225, and 275 lb/acre N (calculated on standard 6-foot beds). The mixed N-P-K Meister fertilizers were 15-5-15 (N-P2O5-K2O) and 19-5-14 (N-P2O5-K2O); each was polymer-coated. Fertilizer placement methods were evaluated by additional trials of broadcast/incorporated NH4NO3 or CR-Meister 15-5-15 (N-P2O5-K2O), applied preplant at 175 lb/acre N. A zero-fertilizer check treatment was also included. The fall experiment occurred near Gainesville on Arredondo fine sand. Beds were mulched with white-on-black polyethylene and drip irrigated to maintain soil moisture at -10 centibars using tensiometers.
Total pepper yield response with the quadratic equation was maximized with 175 lb/acre N (100% RY, 1,079 cartons/acre). With 175 lb/acre N, yield response was 25% higher (1,200 cartons/acre) when CR-Meister 15-5-15 (N-P2O5-K2O) was broadcast and incorporated than when the same fertilizer was applied to the bed surface only. Broadcast/incorporated NH4NO3 resulted in yields 40% lower (730 cartons/acre) than the CR-Meister treatment above. Yields declined with 225 and 275 lb/acre N to 91 and 69% RY, respectively, with the surface-applied CR fertilizers. Total fruit yields or total extra-large fruit yields were not different with either CR-Meister fertilizer, but early yield of extra-large fruit was greatest with 125 lb/acre CR-N or with 175 lb/acre soluble N. Leaf-tissue N concentrations exceeded sufficiency at bloom and first harvest with all N treatments.
Additional studies were conducted at Live Oak with CR fertilizers. Fertilizer materials were different in their performance in the field (Hochmuth 1997b; 1997c; 1998a; 1998b). CR fertilizers resulted in similar or greater pepper yields with reduced N fertilizer (Hochmuth 1997b; 1998a; 1998b). Broadcast application was superior to banding of the fertilizer.
A study of N fertilization effects on specialty pepper (jalapeno) crops was conducted in Live Oak at the SVREC on Lakeland fine sand in spring 1993 (Hochmuth and Hochmuth 1993). Nitrogen treatments ranged from 0 to 240 lb/acre NH4NO3-N in increments of 80 lb/acre. Potassium was applied uniformly at 160 lb/acre K2O (KCl), and no phosphorus (P) was applied due to high soil-test P concentrations. Magnesium was supplied at 50 lb/acre Mg (MgSO4). Tilled soils received fertilizer treatments in a 36-inch-wide band and incorporated. The N recommendation for pepper at the time of this study (Hochmuth and Hanlon 1989a) was 160 lb/acre. Marketable yield of jalapeno responded quadratically to N rate throughout the range of 0 to 240 lb/acre N. The maximum yield resulted with 200 to 240 lb/acre N.
Irrigation and nitrogen management impacts on water quality
Management of N depends on careful attention to irrigation practices to minimize N leaching. Most of the studies summarized in this review focused on yield and fruit quality in response to fertilizer rate. More studies in the late 1990s began to look at the impacts of fertilizer management and irrigation efficiency on nutrient movement and fertilizer-use efficiency. A method was described by Simonne et al. 2003 for using a dye injected into the drip irrigation system to demonstrate water (and thus mobile nutrients such as nitrate-N) movement in the soil with the water. Simonne (2000) described scheduling methods for increasing drip irrigation efficiencies for vegetables.
The distribution of chloride and bromide in soils under a drip-irrigated pepper crop were used as an indicator of soluble fertilizer movement (Graetz et al. 1978). When the soil was irrigated in 1977 to a moisture level of 0.3 bar, chloride was moved out of the root zone in 20 hours. In 1978, water was applied at two quantities, 0.5 pan evaporation and 1.0 pan, and at three application rates, 0.5, 1.0, and 1.5 gal/hr/plant. Chloride testing at two-thirds through the season again indicated rapid movement of nutrients away from the emitters, but there were no differences among the treatments. Increasing the discharge rate to more than 1.5 gal/hr/plant resulted in movement of water below the root zone and also increased lateral movement of water.
Vegetable growers often apply more N fertilizer than recommended to avoid the risk associated with leaching rainfall and high water tables often maintained by growers (Stanley and Clark 2002). These authors studied the new water table control technique, FES. They showed that using a lowered water table depth to 60 to 70 cm instead of 45 cm below the bed surface and using recommended N rate did not negatively impact pepper yields.
High-frequency irrigation control by soil moisture sensors resulted in equal pepper yields but with less water requirements, compared with twice-daily irrigation treatments (Dukes et al. 2003). High-frequency irrigation led to higher volumetric soil moisture with 50% less seasonal irrigation needs. Another study coupled drainage lysimeters with soil moisture sensor (SMS) irrigation control to measure N leaching using selected irrigation strategies (Dukes et al. 2006). SMS control of irrigation resulted in 37 to 66% less water used on pepper when compared to a single timed irrigation event typical of commercial grower practice. Yield was similar with all irrigation treatments, but irrigation efficiency was 2 to 3 times better with SMS. The amount of water captured in the lysimeters and the mass of nitrate-N were lower with the SMS irrigation control, compared to the timed-irrigation practice.
Plant nutrient use efficiency refers to the proportion of an applied nutrient that is absorbed and used by the crop. A study using 15-N depleted ammonium sulfate applied at 200 lb/acre N was conducted for two seasons, 1975 and 1976 in Gainesville, FL (Locascio et al. 1985). The N was applied in a single application or in splits with or without plastic mulch. During a dry season, fruit yield and N accumulation were not influenced by treatment. At the end of the season, N accumulation was 16.5% in the shoots, 2.2% in the immature fruits, and 15.8% in the harvested fruits for a total utilization of 34.5% (70 lb/acre). The soil supplied approximately 35 lb N. In a wet season, yields and N accumulation were influenced by treatment. N accumulations by shoots, immature fruits, and harvested fruits summed were 41.8% (84 lb/acre), 7.6% (15 lb/acre), and 23.9 % (48 lb/acre) for the three treatments, mulch, no-mulch-single, and no-mulch-split, respectively. N from the soil was 24, 10, and 13 lb/acre with the three treatments.
McNeal and colleagues conducted research on N and P losses from vegetable fields in Southwest Florida in the mid-1990s. The basis for the project was that nitrate-N was being found in drinking water wells in Central Florida counties (McNeal et al. 1994). These researchers noted differences in nitrate pulses in groundwater samplers between the deep sandy soils in the central ridge of the state where citrus is grown and the flatwoods soils in Southwestern Florida where vegetables are grown (McNeal et al. 1995). The research showed rapid dissipation of nitrate pulses below the vegetable area compared with the citrus area. Denitrification was offered as a mechanism to explain the differences in nitrate dissipation. These authors offered several BMPs for vegetable and citrus producers.
The same research group studied phosphorus. Unlike nitrate pulses that dissipated, phosphate tended to persist in the high upper water tables in vegetable fields. Naturally occurring phosphate deposits appeared to possibly contribute phosphate to shallow water tables (Stanley et al. 1995). Understanding the local geology relating to phosphate deposits is important in locating water retention areas.
Using PVC columns in a greenhouse, researchers evaluated N residence time in the root zone of fertilizer use by the plant (Scholberg et al. 2009). Weekly irrigation with KNO3 was applied 1, 3, and 7 days before weekly removal of residual N by leaching, and results were compared with those from an unplanted reference column. At 77 days after planting, increasing the residence time increased the weekly uptake from 1–10 to 12 lb/acre. Overall fertilizer use efficiency was 8, 31, and 45%, respectively. The authors concluded that early in the growing season, fertilizer efficiency could be increased with more frequent fertilizer applications and careful irrigation to increase the residence time of fertilizer in the root zone.
Three methods of monitoring nitrate leaching were compared for several vegetables, including pepper (Zotarelli et al. 2007). The three methods were ceramic cup suction lysimeters, drainage lysimeters, and soil cores. There were consistent patterns between drainage lysimeters and soil cores. Estimated N loading rates based on suction cups were lower than for the other two methods. Applying N rates in excess of recommended amounts increased leaching losses by 64%.
Nitrogen Summary
Research with N fertilization of pepper spans more than 50 years from many production areas using three major methods of irrigation, summarized in Figure 5. Early pepper N recommendations of 160 lb/acre (Montelaro 1978) were increased to 175 lb/acre in 1995 (Hochmuth and Hanlon 1995). The earlier review by Hochmuth and Cordasco (2000) led to a further revision of the pepper recommendations, increasing the recommended rate from 175 to 200 lb/acre. It is apparent from the literature that average pepper yields increased with time due to improved cultural systems, new varieties, etc., and these increased yields justified the increases in the N rate recommendations.
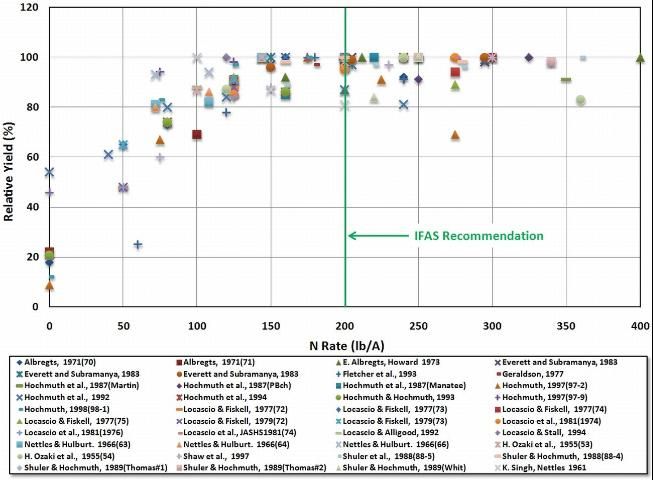
More fertilization research has been conducted in Florida since the last review in 2000. Yields with overhead-irrigated pepper responded positively to N fertilization up to 200 lb/acre N in most studies. Mulched, broadcast, and incorporated preplant N fertilizer proved the most efficient application method for pepper N uptake. Mulched pepper yielded 25% more than unmulched pepper. Overhead-irrigated, mulched soils retained 70% of the applied N three and fifteen weeks after planting. Band application proved the poorest placement method for NH4NO3 in amounts greater than 200 lb/acre N, and (NH4)2SO4 in amounts greater than 125 lb/acre N caused soluble-salt injury at higher N rates. Best yields often occurred when a portion of the N was supplied from a CR source.
Subsurface-irrigated pepper responded to N fertilization in the range of 150 to 275 lb/acre N. Results in some studies produced yield responses to rates exceeding the current recommendations of 200 lb/acre. At N rates more than 275 lb/acre, yields were reduced. Excessive N rates were shown to be related to increased fruit disorders such as blossom-end rot. Yield responses to N fertilization of subirrigated pepper were similar for eastern (Palm Beach County) and west coast (Manatee) production sites. Research with drip-irrigated pepper documented greatest yields with 200 to 240 lb/acre. When N rates and irrigation rates were studied in factorial combinations, peppers responded to N rate at 125% (250 lb/acre) of the current recommendation.
CR fertilizers have been shown to be effective for pepper production. Materials available in the 1990s could be used to produce pepper with slightly reduced N rates, for example 175 lb/acre N compared to the current recommended rate of 200 lb/acre N. Advancements are being made in CR fertilizers, and some have been shown to be effective in reducing the N fertilizer needs for crops (Simonne and Hutchinson 2005). This new review includes several studies that support a slight increase in N rate to slightly more than the current N rate of 200 lb/acre when soluble fertilizer is used. In addition, there have been studies that show that, with careful attention to irrigation, the current recommendation for N is adequate.
These studies have provided data to determine sufficiency ranges for plant tissue testing including whole-leaf and fresh petiole sap testing (Hochmuth et al. 2009). Blossom-end rot has been associated with excessive N fertilization. Based on years of fertilizer and irrigation research with pepper in Florida, blossom-end rot prevention strategies were summarized by Hochmuth and Hochmuth (2009).
More work is needed to better define effects of N management on soil and groundwater N concentrations. Research is needed to define the most efficient and environmentally safe management techniques to apply N with emphasis on soluble-N application timing, N fertigation, and CR sources.
Phosphorus and Potassium
Soil Testing
Knowledge of soil nutrient levels, particularly phosphorus (P) and potassium (K), before planting is the starting point to predicting pepper response to varying rates of applied P and K. The Mehlich-1 (M-1) (double-acid) solution is the extractant currently used by Florida and several other Southeastern US states for sandy soils (Mylavarapu 2009).
M-1 extractant indices (expressed as ppm soil-extracted nutrient) are classified as very low, low, medium, high, and very high; and a crop-specific fertilizer recommendation is made from that classification (Hochmuth and Hanlon 1995). The M-1 solution became the accepted extractant standard in 1979 at the University of Florida. Prior to M-1, ammonium acetate and water extractants were used. Indices recorded from these methods cannot be directly equated with M-1 indices, but review of research results from studies with these extractants presents a profile of pepper response in varying fertilization conditions. This review also summarizes practices in water management, fertilizer application methods, fertilizer source, and the effect of mulch in the nutrient management system.
Phosphorus
Relatively few studies, summarized in Figure 6, have been reported concerning P requirements of pepper. Current P recommendations for pepper are based on M-1 soil-test results. IFAS Standardized Fertilization Recommendations for Vegetable Crops (Hochmuth and Hanlon 1995; 2000) provides five rating levels, from very low to very high, for extractable soil P. Recommended application rates in lb/acre P2O5 are given for each rating level. Soils testing high and very high in P require no P fertilization. Yield results from a Live Oak study (Hochmuth et al. 1992b) support this recommendation, with an optimum RY of 95% (712 cartons/acre) with 0 lb/acre P2O5. Addition of 50 or 100 lb/acre P2O5 did not improve the yield, quality, or grade of peppers at this site testing high in M-1 P.
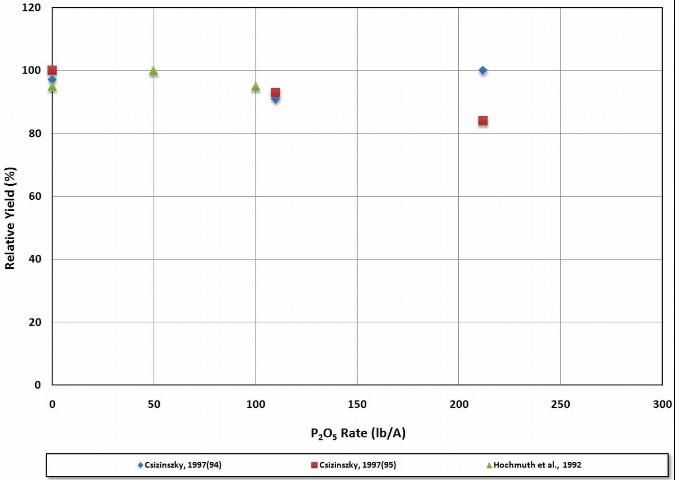
Fine sand soils, in an early study on the lower east coast of Florida, contained water-extractable P concentrations ranging from trace levels to 2 ppm (Ozaki and Hortenstine 1962). Phosphorus application of 50 lb/acre P2O5 (100% RY, 652 cartons/acre) doubled the yield compared to the check (zero P) treatment, while additional P at 100 lb/acre P2O5 gave no additional yield response (68-inch beds). Side-dressing half of the P during the growing season did not increase yields compared to banding all of the P at planting. Plants with all treatments, including the check treatment, had more than 0.3% leaf-tissue P concentrations. Leaf-tissue concentrations more than 0.3% are considered adequate for leaf tissue sampled at first blossom, early fruit set, and early harvest (Hochmuth et al. 2009). Plants with zero P, however, were slow to mature, had less vegetative growth, and yielded 45% less than P-treated plants.
A M-1 extractable P index of 18 lb/acre (9 ppm) reported in an N and K subsurface-irrigated pepper study was interpreted as very low for pepper production (Everett and Subramanya 1983). Beds were spaced on 6-foot centers. For this experiment on Immokalee fine sand, 240 lb/acre P2O5 were added with excellent yield results (1,375 cartons/acre, average yield during the three seasons and three N rates). Current Institute of Food and Agricultural Sciences (IFAS) standardized pepper recommendations, based on M-1 soil-index values, predict that 160 lb/acre P2O5 would have been needed in this case for optimum growth.
Phosphorus fertilizer sources used with fertigation systems were limited by cost, tendency to bind with Ca and Mg in the water source and clog emitters, and by their immobility away from the emitter once applied (Csizinszky 1997). Availability of a new, fully water-soluble monopotassium phosphate (KH 2PO 4) led to experiments to evaluate bell pepper response to ratios of dry P (applied preplant) and to soluble injected P. Experiments were conducted in Bradenton, at the Gulf Coast Research and Education Center, on EauGallie fine sand soils, in fall 1994 and spring 1995. Soil P concentrations—extracted using M-1—were considered medium (21 ppm), and 100 lb/acre P 2O 5 were recommended (Hochmuth and Hanlon 1995). Dry fertilizer sources, NH 4NO 3 (85 lb/acre N) and K 2SO 4, were applied preplant in a band above the irrigation tube. Additional N and K were injected as NH 4NO 3, KNO 3, and KH 2PO 4. Total N applied to all treatments was 260 lb/acre. Phosphorus treatments included P rates of 0, 106, or 213 lb/acre P 2O 5 from either dry or liquid P sources or a combination of both. With the 106 lb/acre P 2O 5 treatment, P was applied all preplant in an 8-inch-wide band before bedmaking as either 33% preplant (superphosphate) and 67% liquid injected (KH 2PO 4) or as 100% liquid injected (KH 2PO 4). A fourth treatment was 213 lb/acre P 2O 5 (superphosphate) applied preplant. Beds were spaced on 5-foot centers and mulched with white-on-black polyethylene in the fall and black polyethylene in the spring. Soil moisture concentrations were maintained with tensiometers.
Marketable yields did not respond to P fertilization rates, time of application (preplant or injected), or P source in either experiment season. Yield of fancy-grade fruit was decreased with P fertilization in the fall 1994 season. Researchers noted that the demand for P was greatest early in the growing season and that application thereafter may only have been effective to correct late-developing P deficiency symptoms. Researchers concluded that no P was needed for optimum pepper yields where soils tested 21 ppm M-1 P (current recommendations are for 100 lb/acre P2O5). Yields in the fall of 1994 surpassed the West Central Florida average yield of 666 cartons/acre with an average of 1,047 cartons/acre across all P treatments that season. Yields in the spring of 1995 were less than the average regional yield of 911 cartons/acre with an average of 663 cartons/acre.
Potassium
Overhead Irrigation
The overhead irrigation studies presented here predate the use of M-1 extractant for K; therefore only changes in yield response are measured against increases in applied K.
For pepper mulched with polyethylene-coated paper at Dover, Florida (Albregts 1971), the best yield in 1970 was achieved with 160 lb/acre K2O. One-third of the fertilizer was incorporated, the remainder was banded in the bed center, and rows were 56 inches apart. A 79% RY (574 cartons/acre) was produced without fertilizer on this site that contained 102 ppm water-extractable K. The following year, K had depleted to 16 ppm (measured with water-extractant method), and 200 lb/acre K2O were required to produce 97% RY (644 cartons/acre).
For overhead-irrigated mulched pepper, yield was maximized with 200 lb/acre K2O and declining thereafter as K2O rate was increased to 360 lb/acre. Current UF/IFAS recommendations are for 160 lb/acre K2O on soils testing low in K. Results from this mulched crop indicate favorable yield responses with successive annual crops when one-third of the fertilizer is incorporated and two-thirds are banded preplant at bed center with K2O rates of 160 to 200 lb/acre for each season.
Subsurface Irrigation
Crop response to increasing rates of N and K fertilizer was measured for two seasons in an unmulched factorial experiment at Plantation Field Lab near Fort Lauderdale (Ozaki et al. 1955). The water table was maintained two feet below the bed surface, and N and K were applied in five equal monthly applications. Row spacing was not indicated in this study, but plants within the row were 12 inches apart. Relative yield of fancy plus No. 1 grade peppers increased significantly in 1953–1954 with increasing K2O from 100 to 200 lb/acre, 66% to 94% RY (796 cartons/acre), respectively. Relative yield did not increase significantly when K2O rates were increased to 300 lb/acre (100% RY). In the second season, 1954–1955, plants were thinned to 9 inches, and RY responses of fancy fruit were not different—96, 94, and 100% RY (572 cartons/acre) with K2O rates of 120, 240, and 360 lb/acre, respectively. Yields were generally down from the previous year due to a dry second season. A shortage of available moisture during this dry season caused salts to accumulate at the surface, leading to soluble-salt injury.
Early studies on K fertilization studied the interaction of N and K with respect to a disorder referred to as "bronzing" of leaves (Ozaki and Hamilton 1954). Bronzing typically appeared after the first harvest and was intensified by high N rates. Bronzing was identified as a potassium deficiency in which dried, bronzed leaves had a K concentration of 0.32%, and healthy leaves were 1.24% K.
Nitrogen and K rates from 50 to 1,800 lb/acre were evaluated in an early N and K "ratio" study (Iley and Ozaki 1966). Subirrigated beds were fertilized with 30-500-30 lb/acre N-P2O5-K2O, which were broadcast and incorporated preplant. Additional N and K fertilizers were banded between rows for a total N application of 300 lb/acre and total K rates of 50, 300, 900, and 1,800 lb/acre K2O. Beds were mulched with polyethylene. Best yields occurred with 300 lb/acre K2O, 99% RY (70 lb fancy and U.S. No. 1 fruit/20 x 5.5-foot double-row plot). Potassium treatments between 50 and 300 lb/acre K2O were not included, making this study incomplete for rates nearer the current recommended K rates. Results from a "ratio" study are difficult to interpret. It is impossible to determine because the "ratio" effect is confounded with the N or K rate effects. A range of "ratios" must by definition be composed of changes in rates of the nutrients involved.
Commercial fields in three South Florida counties, Manatee, Martin, and Palm Beach, were used in a 1987 K study (Hochmuth et al. 1987a; 1990; and 1993). The fields tested low, 23 to 24 ppm, for M-1-extracted K, and 130 lb/acre K2O was recommended. There were five fertilizer treatments in each experiment. Part of the fertilizer was broadcast and incorporated, and the remaining K was banded in single, double, or triple bands, depending on the experiment. All locations had a spodic horizon and were polyethylene mulched and subsurface irrigated. Beds were spaced on 6-foot centers with two plant rows per bed. Pepper plants efficiently extracted sufficient K from the low-K soils for adequate crop yields with starter-fertilizer K rates—40, 25, and 50 lb/acre K2O at Martin, Palm Beach, and Manatee counties, respectively. Leaf-tissue K concentrations, however, were less than 3.0% and were considered inadequate for K with the starter rate at Martin and Palm Beach counties and with 100 lb/acre K2O at Martin County. Warmer spring temperatures induced greater K uptake, resulting in 3.6% midharvest leaf-tissue K concentration in Manatee County with the starter K rate. Yield responses were not different with increasing K rates in any county through 280 lb/acre K2O. The K rate resulting in the highest RY for all three counties was 100 lb/acre K2O—99% RY, 1,121 cartons/acre, in Martin County; 100% RY, 847 cartons/acre, in Palm Beach County; and 100% RY, 1,370 cartons/acre, in Manatee County (Hochmuth et al. 1987a; 1990; and 1993).
Peppers were transplanted in late September 1987 in a Boynton Beach demonstration, where conditions were favorable for a fall crop (Shuler et al. 1988a). Soils tested medium in M-1-extracted K (60 ppm), and 100 lb/acre K2O were recommended. Two side-band applications of starter fertilizer containing half of the recommended K rate, 48-64-48 lb/acre N-P2O5-K2O, resulted in 97% RY. Additional K fertilizer banded at bed center resulted in 100 (1,540 cartons/acre) and 97% RY with 110 and 170 lb/acre K2O, respectively.
A reduced K rate of 150 lb/acre K2O was compared with a higher grower rate of 512 lb/acre K2O at two Palm Beach County demonstration sites. At a third site, the reduced rate was 130 lb/acre K2O, and the grower rate was 306 lb/acre K2O (Shuler and Hochmuth 1989). Starter fertilizer was broadcast at all three sites for both experimental and grower rates—50 lb/acre K2O were broadcast at the first two sites, and 30 lb/acre K2O were broadcast at the third site. Beds were spaced 6-feet on center with two plant rows per bed.
Pepper yield was similar with either 150 or 512 lb/acre K2O at both trial sites. Soil tests, following application of the starter fertilizer, resulted in medium (43 ppm) M-1-extracted K, and 100 lb/acre k2O were recommended (Shuler and Hochmuth 1989). Relative yields with the 150 lb/acre K2O rate were 91 and 100% (1,622 and 1,013 cartons/acre) at each location. No yield advantage occurred with the 512 lb/acre K2O grower rate. Leaf K concentrations were deficient at early-harvest sampling at the first location and adequate at the second location. The third Delray Beach site, tested after 30 lb/acre K2O was broadcast, was low to very low (20 ppm) in soil K, and 130 lb/acre K2O was recommended. Yield response with the higher grower rate of 306 lb/acre K2O was significantly greater—100% RY (1,200 cartons/acre) compared to 83% RY with 130 lb/acre K2O. Deficient leaf-tissue K concentrations of 2.0% at early harvest indicated 130 lb/acre K2O was not sufficient at this site.
Current IFAS recommendations for subsurface-irrigated mulched pepper specify preplant incorporation of 10 to 20% K with the remainder banded 6 to 10 inches from plants at 2- to 3-inch depths (Hochmuth and Hanlon 1995). Maximum yields, 100% RY (1,339 cartons/acre), occurred in a Delray Beach study when 80 lb/acre K2O of the fertilizer was broadcast preplant under polyethylene mulch (Hochmuth et al. 1994). Experimental plots were 5.5 x 18 feet. Pepper production with banded K fertilizer was more inefficient, leaving large amounts of residual K in the band at the end of the season and resulting in 1,117 cartons/acre with the same 80 lb/acre K2O rate. Higher yields with banded K fertilizer, 1,161 cartons/acre, required 240 lb/acre K2O.
Unfertilized pepper plants extracted sufficient K from very low, 18 ppm, soils for adequate leaf-tissue K (3.7%) at early fruit set (Hochmuth et al. 1994). Fruit yields, however, were not sustained on unfertilized soil, yielding 561 cartons/acre compared to 1,339 cartons/acre with 80 lb/acre K2O. A fruit-shrivel rating from 1 to 10, beginning with no shrivel to fruit wrinkled and soft, was made for pepper fruits. Shrivel rating generally increased with increasing K rate advancing from ratings of 5.0 to 6.3 to 7.0 for respective K2O rates of 80, 160, and 240 lb/acre. Higher rates of K2O fertilization increased shrivel and decreased shelf life of pepper. Excellent yields, 35% more than the average state yield for 1993—1994, indicated water management practices were satisfactory.
The authors chose the quadratic equation to express extra-large and large fruit yield responses when all of the broadcast K was supplied from CR-K. At this CR application, extra-large and large fruit yield peaked with a total K2O application of 160 lb/acre (1,362 cartons/acre) and then declined with 240 lb/acre. Total marketable fruit yields with these same rates responded linearly. The slight declines in extra-large and large fruit yield were attributed to excess K with the 240 lb/acre rate. When a portion of K was supplied from a CR source in quantities of 0, 50, or 100% of the broadcast-K, fruit-quality parameters of wall thickness, firmness, shrivel, and fruit dry weight were not affected.
Pepper response to applied K varied, and at times, unexpected amounts were extracted by the crop from soils testing very low in K. Peppers grown in cooler winter-season plantings responded to K near the recommended rates, but peppers grown in warm-season plantings responded to rates near half the recommended rate. Absorption of K was most efficient with subirrigated pepper when some of the K was incorporated in the soil as opposed to all of the K banded on the bed surface.
Drip Irrigation
Studies of K fertilization of pepper grown with drip irrigation are minimal. Several studies are summarized here. The first is an N and K study conducted at the University of Florida Horticultural Research Unit in Gainesville (Locascio and Alligood 1992).
Soluble and CR fertilizers were evaluated from ten N and four K sources. The soluble K source was KNO3 + NH4NO3. CR-K sources were coated KCl + NH4NO3, coated KNO3 + NH4NO3, and coated KNO3 + coated urea. Nitrogen and K were applied 40% preplant (87 lb/acre K2O) and 60% fertigated (130 lb/acre K2O) once weekly for 10 weeks. Treatments that included KNO3 received all of the KNO3 preplant. Single-row beds were spaced 4 feet apart. Pepper plants yielded significantly more with the soluble KNO3 + NH4NO3 source (1,175 cartons/acre) than with the coated KCl + NH4NO3 (975 cartons/acre) source. Similar yields to both the high and low yields above resulted with the CR-K sources coated KNO3 + coated urea (1,068 cartons/acre) and coated KNO3 + NH4NO3 (1,010 cartons/acre).
Potassium recommendation for a Live Oak site with medium (56 ppm) M-1 K was 100 lb/acre K2O (Hochmuth et al. 1992b). Potassium chloride was applied by hand and incorporated at rates of 0, 50, and 100 lb/acre K2O. Beds were set on 5-foot centers, two rows per bed, and 9-inch between-plant spacing. Early- and total-harvest yields increased linearly with added K. The greatest yield increases for both harvests were between zero and 50 lb/acre K2O, 67 and 97% early-harvest RY and 86 and 97% (655 cartons/acre) total-harvest RY, with each respective rate. Yield appeared to level off with 50 lb/acre K2O, increasing only 3% with the recommended 100 lb/acre rate. Whole-leaf K concentrations at early fruit set were adequate with all K2O rates including zero lb/acre K2O.
Experiments with N and K fertilization of pepper were conducted in Live Oak (SVREC) on Lakeland fine sand in spring 1992 (Fletcher et al. 1993). Additional data from petiole-sap testing were sought to calibrate N and K concentrations from peppers grown with drip irrigation and polyethylene mulch. Potassium from KCl, with 160 lb/acre NH4NO3-N, was broadcast across a 3-foot swath of soil, tilled, and formed into beds for K treatments of 0, 50, 100, 150, and 200 lb/acre K2O. Very low residual soil K concentrations (18 ppm) were measured with an M-1 soil test, and the IFAS K recommendation for plants grown on 6-foot bed centers was 160 lb/acre K2O. A response to 190 lb/acre K2O was expected for this experiment where beds were spaced on 5-foot centers. Beds were drip irrigated and soil moisture tensiometer-maintained between -8 and -12 centibars. Cardy (Spectrum Technologies, Plainfield, Illinois) ion meters were used to test petiole-sap N and K concentrations.
Yields of marketable and U.S. No. 1 grade fruits increased quadratically (1% probability) with increased K fertilizer. Marketable fruit yields leveled off when the rate exceeded 100 lb/acre K2O (717 cartons/acre, 100% RY carton weight not specified). Likewise, leaf-tissue K concentrations increased quadratically at all sampling dates, leveling off at rates more than 100 lb/acre K2O (4.8%) at 1st flowering and early fruit set and at more than 150 lb/acre K2O (4.9%) during harvest. Plants grown on very low residual-soil K concentrations (0 lb/acre K2O) had deficient to near-deficient leaf K concentrations at all sample dates. Potassium fertilization had a positive (linear) effect on average fruit weight and on petiole-sap K concentrations at all sampling dates. Petiole-sap concentrations of 3,200 ppm through early fruit set and 3,900 ppm during harvest were tied with the optimum-yielding K treatment of 100 lb/acre K2O.
In a Live Oak study, low (20 ppm) M-1-K soils received a recommendation of 130 lb/acre K2O (Hochmuth and Hochmuth 1995; Hochmuth and Hanlon 1995). Soluble KNO3 and CR polymer-coated KNO3 were used as the K sources. Fertilizers were blended, applied to the bed, and incorporated at K2O rates of 100 or 150 lb/acre, with percentages of 0, 25, and 50% supplied from the CR-K source. Beds were set on 5-foot centers with fertilizer-rate calculations based on 6-foot bed centers. Black polyethylene mulch was applied, and drip irrigation was tensiometer-controlled at -8 to -12 centibars.
Total marketable yield was similar with 100 or 150 lb/acre K2O (545 or 554 cartons/acre, respectively). Significantly higher fancy-fruit yield, fewer U.S. No. 2 fruit, and better average fruit weight were reported when 150 lb/acre K2O were applied compared to the 100 lb/acre rate. Applying the very-low-soil K fertilizer recommendation of 160 lb/acre K2O on this site, interpreted as either very low or low, improved pepper quality (Hochmuth and Hochmuth 1995). Data from studies did not show continued improvement in fruit quality and weight with K rates greater than the recommended rate.
Leaf-tissue K concentration at first flowering was 5.6% with 100 lb/acre K2O and 6.0% with 150 lb/acre K2O, both high concentrations for this stage of growth (Hochmuth et al. 2009). Tissue K concentrations remained high through the second harvest period and increased slightly with 25 and 50% K2O applied from a CR source. Success with lower fertilizer rates were attributed to careful water management practices and CR fertilizers. Application of the recommended 130 lb/acre K2O adequately ensured good crop production at this site.
Potassium Summary
Responses of pepper production to K fertilization have been variable. Yield responses to K fertilization leveled off between 150 and 300 lb/acre K2O in most studies. However, optimum yields with less-than-recommended rates of K also were reported. In some studies, only small responses to K resulted when large responses were predicted by soil tests. Predictive soil testing for K, a mobile element in sandy soils, appears questionable. Yield responses in most studies on soils testing very low in K leveled off at approximately 160 lb/acre K2O. Some responses were still evident to approximately 200 lb/acre K2O (Figure 7).
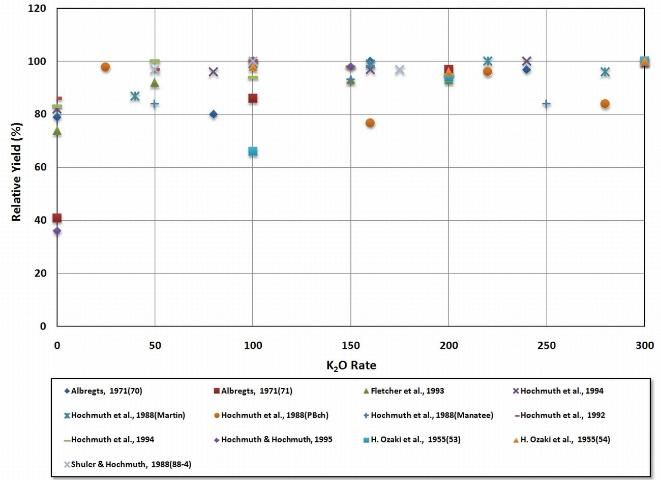
Summary
Bell peppers shipped from Florida growers to major cities in the US account for 39% of the marketed peppers in the US Pepper production acreage declined 9.5% from 21,000 acres in 1996 to 19,000 acres in 2008. The per-acre yield has increased from 14.5 million cartons (730 cartons/acre) to more than 18.2 million cartons (962 cartons/acre). Improvements in plant nutrition and water management practices, as well as improvements in weed and pest management, likely contributed to recent yield increases.
Continued yield increases require efficient use of water and fertilizers to sustain plant nutritional demands throughout the season while minimizing nutrient losses to the environment. Positive yield results from experiments with CR N-P-K fertilizers encourage further research with single-application, long-term nutrient supply materials. Studies are needed on optimal timing of N application with drip-irrigation systems and on the fate of N in the soil system as it relates to N and irrigation management. Large-scale demonstrations of recommended nutrient management programs on commercial farms are also needed. Some success occurred with CR-K fertilizers used as starter fertilizers on fertigated pepper, but further experimentation is needed to document pepper yield response to fertigated K.
Research for P fertilization of pepper plants has only been conducted in a few field experiments. Although P is not mobile within most soils (as with N and K), P accumulates readily with excess application on sandy soils and is readily carried with eroding soils becoming a pollutant. Pepper yields responded to less than the recommended P rate in three experiments in which soils had very low and medium soil-test P concentrations. No yield response resulted from dry or liquid-injected P sources in two experiments. Additional research is needed to document pepper yield responses to varying P rates and varying methods of P application.
As a result of improved irrigation technologies and practices, there is a greater potential for obtaining high bell pepper yields while reducing fertilizer and irrigation water inputs. The FES system has been reported to reduce irrigation requirements by 30–40% by increasing the soil rainfall storage capacity and decreasing rainfall runoff (Smajstrla et al. 2002). Although additional studies would be beneficial, the results from using the FES system in bell pepper production supported the IFAS recommended N and K fertilization rates. The potential of this system for reducing costs and minimizing impacts on the environment should be further investigated.
Overall, research conducted since the previous review supported the current IFAS fertilizer recommendations for N, P, and K. There were a few studies, especially seepage-irrigated pepper, where pepper yield responded to up to 250 lb/acre N. The changing technologies involving irrigation management, improved fertilizer sources, and disease-resistant cultivars have all contributed to advancing and improving pepper yields. Documenting these innovations for appropriate fertilizer and irrigation methods is an ongoing challenge for the industry, the environmental community, and the University of Florida. Pepper has been demonstrated to be inefficient in utilizing fertilizer. There are some studies that may provide some justification for a slight increase in N rate for soluble fertilizers, e.g., with drip fertigation. More work is needed to better describe the N and irrigation practices that result in increasing pepper N utilization efficiency and in minimizing N losses. There is a paucity of research on P fertilization. There were few studies that demonstrated a response to P. Potassium studies generally demonstrated that the current IFAS recommendations for K are adequate for preventing K deficiencies.
Some pepper fertilizer best management practices supported by the research:
- Several studies documented low N-use efficiency with pepper, 30 to 50%, showing the need to carefully manage N fertilizer, including rate, timing, and irrigation.
- Polyethylene mulch results in increased fruit yields and improved N efficiency. Leaching of N was reduced with mulch compared to bare ground production.
- Pepper fruit yield most often was maximized with 200 lb/acre N but sometimes responded to more N, up to 240 lb/acre, especially with extra harvests (extended seasons). This extra N could be applied by drip irrigation or the liquid fertilizer injection wheel.
Literature Cited
Albregts, E. E. 1971. Effect of nitrogen and potassium on bell pepper grown under paper mulch. Soil Crop Sci. Soc. Fla. Proc. 31:116-118.
Albregts, E. E., and C. M. Howard. 1973. Effect of fertilization and mulching with biodegradable polyethylene-coated paper on responses of okra and peppers. HortScience 8:36-38.
Black, C. A. 1992. Soil fertility evaluation and control. Lewis Publishers, Boca Raton, FL. 746 pp.
Brown, J. R. 1987. Soil testing: sampling, correlation, calibration, and interpretation. Soil Science Society of Amer. Special Publ. No. 21. Soil Science Society of America, Inc. Madison, Wisc.
Csizinszky, A. A. 1997. Response of micro-irrigated bell pepper to phosphorus sources and rates. Soil Crop Sci. Soc. Fla. Proc. 56:20-24.
Dukes, M. D., E. H. Simonne, W. E. Davis, D. W. Studstill, and R. Hochmuth. 2003. Effect of sensor-based high-frequency irrigation on bell pepper yield and water use. Proc. 2nd International Conf. on Irrigation and Drainage. P. 665-674.
Dukes, M. D., L. Zotarelli, J. M. S. Scholberg, and R. Munoz-Carpena. 2006. Irrigation and nitrogen best management practices under drip-irrigated vegetable production. Procs. ASCE EWRI World Water and Environ. Resource Cong. P. 1-11.
Everett, P. H. 1977. Controlled-release fertilizers: Effect of rates and placements on plant stand, early growth and fruit yield of peppers. Proc. Fla. State Hort. Soc. 90:390-393.
Everett, P. H., and R. Subramanya. 1983. Pepper production as influenced by plant spacing and nitrogen-potassium rates. Proc. Fla. State Hort. Soc. 96:79-82.
Fiskell, J. G. A., S. J. Locascio, S. Singholka, and F. G. Martin. 1978. Effects of fertilizer N sources, rates, and placement on soil test values for bedded peppers with and without mulch. Soil and Crop Sci. Soc. Fla. Procs. 37: 185-187.
Fletcher J., R. Hochmuth, and G. J. Hochmuth. 1993. Calibration of N and K fresh-sap quick-test procedures for polyethylene-mulched peppers. Proc. 24th Nat'l Agric. Plastics Congress, Amer. Soc. for Plasticulture.
Florida Dept. of Agriculture and Consumer Services. 2005. Water quality/quantity best management practices for Florida vegetable and agronomic crops. http://www.floridaagwaterpolicy.com/PDF/Bmps/Bmp_VeggieAgroCrops2005.pdf.
Florida Dept. of Agriculture and Consumer Services. 2009. Florida Agricultural Statistics - http://www.florida-agriculture.com/fass_activities.htm.
Geraldson, C. M., 1977. Pepper production efficiency using the gradient-mulch concept. Proc. Fla. State Hort. Soc. 90:385-388.
Graetz, D. A., J. G. A. Fiskell, S. J. Locascio, B. Zur, and J. M. Myers. 1978. Chloride and bromide movement with trickle irrigation of bell peppers. Proc. Fla. State Hort. Soc. 91: 319-322.
Hanlon, E. A., and G. J. Hochmuth. 1989. Calculating fertilizer rates for vegetable crops grown in raised-bed cultural systems in Florida. Fla. Coop. Ext. Serv. Spec. Series SS-SOS-901.
Hochmuth, G. J. 1996. Fertilization of pepper in Florida. Fla. Coop. Ext. Serv. Circ. 1168.
Hochmuth, G. J. 1997a. Response of pepper to Meister controlled-release fertilizers. Fla. Agr. Expt. Sta. Research Report: Suwannee Valley REC 97-02.
Hochmuth, G. J. 1997b. Mulched pepper response to Pursell Polyon controlled-release fertilizers. Fla. Agr. Expt. Sta. Research Report: Suwannee Valley REC 97-04.
Hochmuth, G. J. 1997c. Response of pepper to Meister controlled-release fertilizers. Fla. Agr. Expt. Sta. Research Report: Suwannee Valley REC 97-09.
Hochmuth, G. J. 1998a. Response of pepper to fertilization with Meister controlled-release fertilizers. Fla. Agr. Expt. Sta. Research Report: Suwannee Valley REC 98-01.
Hochmuth, G. J. 1998b. Evaluation of Pursell controlled-release polymer-coated urea for tomato and pepper, 1997-98. Misc. research report, North Florida Res. And Educ. Center-Suwannee Valley.
Hochmuth, G. J. 1992a. Fertilizer management for drip-irrigated vegetables in Florida. HortTechnology 2:27-32.
Hochmuth, G. J. 1992b. Concepts and practices for improving nitrogen management for vegetables. HortTechnology 2 (1): 121-125.
Hochmuth, G. J. 2000. Management of nutrients in vegetable production systems in Florida. Soil and Crop Sci. Soc. Fla. Proc. 59:11-13.
Hochmuth, G. J., and K. Cordasco. 1999 (reviewed in 2009). Summary of N, P, and K, research on pepper in Florida. Fla. Coop. Ext. Serv. Fact Sheet HS 756.
Hochmuth, G. J., and E. A. Hanlon. 1989a. Commercial vegetable crop nutrient requirements. Fla. Coop. Ext. Serv. Circular 806.
Hochmuth, G. J., and E. A. Hanlon. 1989b. Fertilizer management for bell pepper production in Florida. Fla. Agr. Expt. Sta. Circular S-357.
Hochmuth, G. J. 1994a. Plant petiole sap-testing guide for vegetable crops. Fla. Coop. Ext. Serv. Circ. 1144.
Hochmuth, G. J. 1994b. Sufficiency ranges for nitrate-nitrogen and potassium for vegetable petiole sap quick tests. HortTechnology 4:218-222.
Hochmuth, G. J., and E. A. Hanlon. 1995. IFAS standardized fertilization recommendations for vegetable crops. Fla. Coop. Ext. Serv. Circ. 1152.
Hochmuth, G. J., and E. A. Hanlon, 2000. IFAS standardized fertilization recommendations for vegetable crops. Circular 1152. University of Florida, Institute of Food and Agricultural Sciences, Gainesville, FL.
Hochmuth, G. J., and E. A. Hanlon. 2009. Calculating recommended fertilizer rates for vegetable crops grown in raised-bed mulched cultural systems. Fla. Coop. Extension Serv. Circ. SL 303. https://edis.ifas.ufl.edu/pdffiles/ss/ss51600.pdf.
Hochmuth, G. J., E. A. Hanlon, and B. Hochmuth. 1992a. Response of pepper to N fertilization in a polyethylene mulch and drip-irrigation production system at Live Oak, Fl. Spring 1988. Fla. Agr. Expt. Sta. Research Report: Suwannee Valley AREC 92-29.
Hochmuth, G. J., E. A. Hanlon, and R. Hochmuth. 1992b. Response of pepper, muskmelon, watermelon, and sweet corn to P and K fertilization at Live Oak, FL. Fla. Agr. Expt. Sta. Research Report: Suwannee Valley AREC 92-28.
Hochmuth, G. J., E. A. Hanlon, B. Hochmuth, G. Kidder, and D. Hensel. 1993. Field fertility research with P and K for vegetables-interpretations and recommendations. Soil and Crop Sci. Soc. Fla. Procs. 52:95-101.
Hochmuth, G. J., and B. Hochmuth. 1993. Jalapeno Pepper Response to N and K Fertilization. Fla. Agr. Expt. Sta. Research Report: Suwannee Valley REC 94-05.
Hochmuth, G. J., and B. Hochmuth. 1995. Effects of K rate and proportion of K supplied from controlled-release K on pepper. Fla. Agr. Expt. Sta. Research Report: Suwannee Valley REC 95-8.
Hochmuth, G. J., and R. C. Hochmuth. 2009. Blossom-end rot in bell pepper: causes and prevention. Fla. Coop. Ext. Cir SL 284. https://edis.ifas.ufl.edu/pdffiles/ss/ss49700.pdf.
Hochmuth, G. J., D. N. Maynard, and M. Sherman. 1988. Pepper production guide for Florida. Fla. Coop. Ext. Serv. Circ. 102E. 18 pp.
Hochmuth, G. J., D. Maynard, C. Vavrina, and E. A. Hanlon. 1991b. Plant-tissue analysis and interpretation for vegetable crops in Florida. Fla. Coop. Ext. Serv. Spec. Ser. SS-VEC-42.
Hochmuth, G. J., D. Maynard, C. Vavrina, E. A. Hanlon, and E. H. Simonne. 2009. Plant tissue analysis and interpretation for vegetable crops in Florida. Fla. Coop. Ext. Serv. Fact Sheet HS 964, https://edis.ifas.ufl.edu/ep081.
Hochmuth, G. J., K. Shuler, and P. Gilreath. 1990. Fertility management for peppers grown with drip and seepage irrigation. Fla. Coop. Ext. Serv. Proc. Fla. Pepper Inst. 1990. SSVEC-002.
Hochmuth, G. J., K. D. Shuler, P. R. Gilreath, and R. L. Mitchell. 1987a. Field-testing of revised Mehlich-I predicted potassium fertilizer recommendations for mulched pepper. Soil Crop Sci. Soc. Proc. 47:30-35.
Hochmuth, G. J., K. D. Shuler, E. A. Hanlon, and N. Roe. 1994. Pepper Response to fertilization with soluble and controlled-release potassium fertilizers. Proc. Fla. State Hort. Soc. 107:132-139.
Hochmuth, G. J., K. D. Shuler, R. Mitchell, and P. Gilreath. 1987b. Nitrogen crop nutrient requirement demonstrations for mulched pepper in Florida. Proc. Fla. State Hort. Soc. 100:205-209.
Iley, J., and H. Ozaki. 1966. Nitrogen-potash ratio study with plastic-mulched pepper. Proc. Fla. State Hort. Soc. 74:211-216.
Kidder, G., E. A. Hanlon, and G. J. Hochmuth. 1989. IFAS standardized fertilization recommendations for vegetable crops. Fla. Coop. Ext. Serv. Spec. Ser. SS-SOS-907.
Locascio, S. J., and M. Alligood. 1992. Nitrogen and potassium source and N-rate for drip irrigated pepper. Proc. Fla. State Hort. Soc. 105:323-325.
Locascio, S. J., and J. G. A. Fiskell. 1977. Pepper production as influenced by mulch, fertilizer placement, and nitrogen rate. Soil Crop Sci. Soc. Fla. Proc. 36:113-117.
Locascio, S. J., and J. G. A. Fiskell. 1979. Pepper response to sulfur-coated urea, mulch and nitrogen rate. Proc. Fla. State Hort. Soc. 92:112-115.
Locascio, S. J., J. G. A. Fiskell, D. A. Graetz, and R. D. Hauck. 1985. Nitrogen accumulation by pepper as influenced by mulch and time of fertilizer application. J. Amer. Soc. Hort. Sci. 110: 325-328.
Locascio, S. J., J. G. A. Fiskell, and F. G. Martin. 1981. Responses of bell pepper to nitrogen sources. J. Amer. Soc. Hort. Sci. 106:628-632.
Locascio, S. J., and W. Stall. 1994. Bell pepper yield as influenced by plant spacing and row arrangement. J. Amer. Soc. Hort. Sci. 119 (5):899-902.
McNeal, B. L., C. D. Stanley, L. A. Espinosa, and L. A. Schipper. 1994. Nitrogen management for vegetables and citrus: some environmental considerations. Soil and Crop Sci. Soc. Fla. Procs. 53:45-51.
McNeal, B. L., C. D. Stanley, W. D. Graham, P. R. Gilreath, D. Downey, and J. F. Creighton. 1995. Nutrient-loss trends for vegetable and citrus fields in west-central Florida. I: nitrate. J. Environ. Qual. 24 (1): 95-100.
Montelaro, J. 1978. Commercial vegetable fertilization guide. Fla. Coop. Ext. Serv. Circ. 225-B.
Mylavarapu, R. S. 2009. UF/IFAS Extension Soil Testing Laboratory (ESTL) Analytical Procedures and Training Manual. Fla. Coop. Ext. Serv. Circ 1248. https://doi.org/10.32473/edis-ss312-2009.
Nettles, V. F., and W. C. Hulbert. 1966. Effect of placements and levels of fertilizer on the yield of vegetables. Proc. Fla State Hort. Soc. 64: 185-191.
Olson, S. M., and E. Simonne (eds.). 2010. The Vegetable Production Handbook. Fla. Coop. Extension Serv. https://edis.ifas.ufl.edu/topic_vph.
Olson, S. M., E. H. Simonne, W. M. Stall, K. L. Pernezny, S. E. Webb, T. G. Taylor, and S. A. Smith. 2010. Pepper production in Florida. Fla. Coop. Extension Serv. https://edis.ifas.ufl.edu/pdffiles/cv/cv13000.pdf.
Ozaki, H. Y. 1961. Effects of spacing, fertilizer, and variety upon pepper yields. Proc. Fla. State Hort. Soc. 74:178-180.
Ozaki, H. Y., and M. G. Hamilton. 1954. Bronzing and yield of peppers as influenced by varying levels of nitrogen, phosphorus, and potassium fertilization. Soil Crop Sci. Soc. Fla. Procs. 14:185-189.
Ozaki, H. Y., and C. C. Hortenstine. 1962. Effect of applied phosphorus on yield and growth of peppers. Soil Crop Sci. Soc. Fla. Proc. 22:89-92.
Ozaki, H. Y., C. T. Ozaki, and M. G. Hamilton. 1955. The effects of applied nitrogen, phosphorus, and potash on yield and growth of peppers. Proc. Fla. State Hort. Soc. 68:230-233.
Ozaki, H. Y., H. E. Ray, and C. T. Ozaki. 1957. Yields and quality of pepper as influenced by ammonium nitrate and sulfate of potash treatments. Soil Crop Sci. Soc. Fla. Proc. 17:210-215.
Schepers, J. S., K. D. Frank, and C. Bourg. 1986. Effect of yield goal and residual nitrogen considerations on nitrogen fertilizer recommendations for irrigated maize in Nebraska. J. Fertilizer Issues 3:133-139.
Scholberg, J. M., L. Zotarelli, R. S. Tubbs, M. D. Dukes, and R. Munoz-Carpena. 2009. Nitrogen uptake efficiency and growth of bell pepper in relation to time of exposure to fertilizer solution. Commun. Soil Sci. and Plant Analysis. 40:2111-2131.
Shaw, N., G. J. Hochmuth, and E. A. Hanlon. 1996. N fertilization management for drip irrigated bell pepper (Capsicum annuum L.). Proc. Fla. State Hort. Soc. 109:136-141.
Shuler, K. D., and G. J. Hochmuth. 1988a. Nitrogen and potassium fertilization of bell peppers, Boynton Beach, FL, fall/winter, 1987-88. Palm Beach. Coop. Ext. Report 1988-4.
Shuler, K. D., and G. J. Hochmuth. 1989. Reduced nitrogen and potassium fertilization of bell peppers in Boynton Beach and Delray Beach, FL, fall/winter 1988-1989. Palm Beach Co. Ext. Report 1989-6.
Shuler K. D., and G. J. Hochmuth. 1988b. Nitrogen fertilization of bell peppers, Jupiter, FL. Spring 1988. Palm Beach. Co. Ext. Report. 1988-5.
Simonne, E. H. 2000. Adaptation of an irrigation scheduling method for bell pepper grown with plasticulture. Acta. Hortic. 2000 (537): 543-548.
Simonne, E. H., M. D. Dukes, R. C. Hochmuth, D. W. Studstill, G. Avezou, and D. Jarry. 2006. Scheduling drip irrigation for bell pepper grown with plasticulture. J. Plant Nutrition. 29 (10): 1729-1739.
Simonne, E. H., and G. J. Hochmuth. 2010. Soil and fertilizer management for pepper production in Florida. Vegetable Production Handbook. Fla. Coop Extension Serv. https://edis.ifas.ufl.edu/pdffiles/cv/cv10100.pdf.
Simonne, E. H., and C. M. Hutchinson. 2005. Controlled-release fertilizers for vegetable production in the era of best management practices: Teaching new tricks to an old dog. HortTechnology 15:36-46.
Simonne, E. H., D. W. Studstill, R. C. Hochmuth, G. McAvoy, M. D. Dukes, and S. M. Olson. 2003. Visualization of water movement in plastic-mulched beds with injections of dye with drip irrigation. Proc. Fla. State Hort. Soc. 116:88-91.
Singh, K., and V. F. Nettles. 1961. Effect of defloration, defruiting, nitrogen and calcium on the growth and fruiting responses of bell peppers (Capsicum annum L). Proc. Fla. State Hort. Soc. 74:204-209.
Smajstrla, A. G., B. J. Boman, G. A. Clark, D. Z. Haman, D. S. Harrison, F. T. Izuno, D. J. Pitts and F. S. Zazueta. 2002. Efficiencies of Florida agricultural irrigation systems. Fla. Coop. Ext. Serv. Bull. 247. https://ufdc.ufl.edu/IR00004382/00001/pdf. Accessed February 16, 2023.
Stanley, C. D., and G. A. Clark. 1993. Water use and nitrogen balance for subirrigated fresh-market bell pepper, fall '92 crop. Proc. Fla. State Hort. Soc. 106:202-204.
Stanley, C. D., and G. A. Clark. 2002. Subirrigated bell pepper production with reduced water table depths and N and K fertilizer rates. Soil Crop Sci. Soc. Florida Proc. 61:84-87.
Stanley, C. D., B. L. McNeal, P. R. Gilreath, J. F. Creighton, W. D. Graham, and G. Alverio. 1995. Nutrient-loss trends for vegetable and citrus fields in west-central Florida: II. Phosphate. J. Environ. Qual. 24:101-106.
Zotarelli, L., J. M. Scholberg, M. D. Dukes, and R. Munoz-Carpena. 2007. Monitoring of nitrate leaching in sandy soils: comparison of three methods. J. Envir. Qual. 36:953-962.