A Summary of N, P, and K Research with Snap Bean in Florida
The purpose of this publication is to summarize the historical Florida research literature on nitrogen, phosphorus, and potassium fertilization of snap bean. The intent of this document is to review literature from all sources pertaining to commercial snap bean fertilization in Florida's growing conditions and to serve as a summary of the scientific basis for appropriate changes, if any, to the Florida Extension snap bean fertilization recommendations.
This publication documents the previous written literature, some of which now appears only in updated modern electronic format. All publications, including the reviewed, older paper-format papers, will be placed in PDF format on the website https://bmp.ifas.ufl.edu for future reference. The research publications created throughout the years have provided the scientific basis for the Florida Extension snap bean fertilization recommendations. Later, these recommendations formed the basis for Best Management Practices (BMP) addressing the achievement of commercial yields and quality while protecting water quality. Snap bean fertilization recommendations should be based on actual field measurements. A compilation of the literature, such as this publication, assists with making science-based fertilizer recommendations that are both commercially viable and reduce risk of environmental consequences in adjacent water bodies. As environmental regulation becomes more commonplace, it becomes more important that fertilization recommendations be based on research.
Fertilizer recommendations not only contain the recommended rate of fertilizer but also the management strategies for getting the most out of the fertilizer investment while protecting the environment. These principles for fertilization of vegetables are summarized by Hochmuth and Hanlon (2010a). Rate is only a part of the modern fertilizer recommendation. Sound fertilizer recommendations also consider irrigation rate, fertilizer materials, placement, and timing of application, among other aspects (Hochmuth and Hanlon 2010a; 2010b).
The fertilization recommendation addresses commercial yield and quality, the economics of crop production, and protection of the environment. Equally important is to have a mechanism available to the grower to adjust fertilization practices during the season due to leaching rains and extension of the growing season for additional harvests when market conditions are favorable. To address all of these concerns, UF/IFAS vegetable recommendations are given as a single target fertilizer rate that is projected to be sufficient for meeting the crop fertilizer needs for most growing seasons. This single target fertilizer rate is a recommended starting point and has been used historically in all vegetable fertilizer recommendations in Florida, as well as around the country. The target recommendation approach with footnotes was used by Montelaro (1978) and confirmed by Hochmuth and Hanlon (1995; 2000). The target value was derived from numerous fertilizer studies and represents a reasonable fertilizer rate that reflects the average maximum crop responses from all of the fertilizer research, not the extremes in responses. However the recommendation process recognizes some growing seasons are different, due to more leaching rains or increases in crop value than can lead to prolonged harvest windows. Hence the target fertilizer amount is accompanied by a series of footnotes that explain the addition of supplemental fertilizer during the growing season, which includes addressing leaching rains and additional harvests near the end of the season. It is logical to select a single target rate based on research that avoids excessive fertilizer applications that often reduce crop nutrient efficiency and increase the potential for environmental degradation.
This literature review includes all available published documentation concerning snap bean yield and fertilizer use in Florida. We chose to present all the research without being selective, as that would introduce bias to the presentation. Inclusion of all Florida literature also documents the development of the commercial production system over time and the fertilizer recommendations with respect to fertilizer use. Average commercial yields of most vegetables have been increasing, primarily due to the proper selection of new cultivars with traits that resist disease and pest pressures and more intensive (greater plant populations) farming practices. Since the basic plant has not changed, nutrient requirements of the plant have also been slow to change.
This publication is an updated version of a previous research literature review by Hochmuth and Cordasco (1999, reviewed 2008) that covers research through 1996. This new review adds any research reports published since 1996 and expands on the role of irrigation in managing fertilizer for crop production efficiency and environmental protection, where such information exists. The audience for this publication includes educators—such as Extension specialists and agents—and commercial vegetable producers, consultants, and governmental agencies.
More than fifty years of snap bean fertilization research has been conducted in Florida. During this time, many changes have occurred in snap bean production practices, including changes in cultivars and introduction of new cultural systems such as closer row spacing. Snap bean crop and fertilizer management recommendations (plant and row spacing) have changed with time in keeping with new developments in research (Hochmuth 1988; 1996a; 1996b; Hochmuth and Hanlon 1989; 1995a; 1995b; 2000; Kidder et al. 1989; Montelaro 1978; Olson et al. 2009; Simonne and Hochmuth 2010; Stal 1983). The most current recommendations for nutrient management for snap bean and other vegetables are presented in the Commercial Vegetable Production Handbook for Florida (Olson and Simonne 2010). The early research focused largely on yield and bean quality in response to fertilization. Since the early research was conducted, and since the first review of the literature in 1999, there have been increasingly strong interests in the consideration of environmental impacts (water quality) in the fertilizer research. The state (Fla. Dept. of Agr. and Cons. Serv. 2005) has been formalizing BMPs and encouraging growers to implement them. Part of the definition of a BMP includes reference to the consideration of both economics and environmental components and that a BMP reflects the best available science.
In the 10-year period from 1998 to 2008, the land area planted to snap beans in the state of Florida increased 18%, yield per acre decreased slightly from 278 to 267 bushels/acre (30-lb bushels), and total snap bean production increased to 9,787,000 bushels (30-lb/bushel) (Fla. Agri. Stat. Bulletin 2009). Most Florida-grown snap beans are harvested in May and June (40%), while 35% are harvested in November and December and 15% in January through March. Forty percent of Florida snap beans are shipped to other states and Canada. Nationally, Florida ranks first in the fresh-market value of snap bean (Olson and Santos 2010). The 2007–2008 production value of this crop was $172,050,000, which represented 8.7% of the total production value of all Florida vegetable crops and 52% of the total US snap bean value.
In 1995, IFAS rate recommendations for N, P2O5, and K2O fertilization of snap beans were revised upward by 20 to 40 lb/acre from those recommended in 1989 (Kidder et al. 1989). In 1995, the revised recommendations specified 90, 120, and 120 lb/acre N-P2O5-K2O, respectively, as maximum rates of nitrogen (N), phosphorus (P), and potassium (K) application for soils testing very low in P and K (Hochmuth and Hanlon 1995a). The current recommended N-P2O5-K2O application rates for soils testing very low in P and K is 100, 120, and 120 lb/acre, respectively (Hochmuth and Hanlon 2000; Olson and Santos 2010). When test values for residual soil concentrations of P and K increase from low to very high, P2O5 or K2O rate recommendations diminish from 100 to 0 lb/acre. Sustained high yields can be expected with fertilization practices designed to supply crop nutrient requirements and protect the environment from excessive fertilization. Use of the Mehlich-1 (M-1) soil test, initiated in 1979, refined the practice of fertilizer rate recommendation and resulted in a crop- and soil-specific guide to fertilizer application. Recommendations for N, P2O5, and K2O fertilization were based on in-field experiments in which optimum fertilizer rates were determined from yield responses to a range of fertilizer rates and with varying climatic conditions, soil types, and snap bean varieties.
Data Summary Method
To evaluate snap bean yield responses to variable rates of fertilizer, a method was needed to standardize the numerous units used for quantifying statewide yield results such as pounds/acre, tons/acre, metric tons/hectare, or cartons per acre (typically a 30 lb crate or bushel). In addition, vegetable yields varied with years and season, cultivar, and location in the state. Percent relative yield (PRY), a calculated percentage, was chosen as the unit to express snap bean yield responses to fertilization. Relative yield is an accepted scientific method for summarizing and presenting data across wide sources and reports (Brown 1987).
This paper uses percent relative yield to take into account the changing conditions associated with season, irrigation, pest pressures, and other cultural practices that directly affect yield. The resulting information effectively avoids the confounding effects mentioned above. Differences between fertilization rates obtained from various research efforts are put on the same scale, allowing for comparisons that could not be obtained if actual yields were used. Relative yield provides for the practical inclusion of numerous research projects in the analyses rather than rely on a single study conducted in one location at one particular time.
In his book, C. Black (1992) summarized the advantages and disadvantages of the RY approach. There are valid statistical concerns about RY, but he concluded that, when applied properly and cautiously, the RY approach can be useful in displaying general relationships. Black demonstrates examples in his book where RY yield is helpful and where it was not. We chose the RY approach because we wanted to display the historical data without making biased decisions about what to include and what not to include in the presentation. Plotting absolute yield data in original units obviously would result in a scatter of data rendering any general interpretation impossible. Black (1992) points out that decisions can always be improved with further research, but the data on hand are the best we have at the time. The highest yield for each fertilizer experiment was assigned a 100% value, and other yields were expressed as a percentage of the highest yield. The actual yield corresponding to 100% RY was presented in 30-lb crates/acre units. The RYs were plotted against rates of nutrient to determine how snap bean yields responded to fertilizer in Florida. The RY presentation allowed data from a variety of experiments to be included in the graphical summary of yield responses. For most studies, RYs of 90 to 100% were not significantly different.
Sometimes the argument is made that growers have expectations of greater yields than those obtained in research projects. It should be pointed out that realistic, regularly obtainable yields might be different from expected yields or "yield goals." Research on this subject has documented that 20% of growers actually reached their yield goals, and only 50% reached 80% of their yield goals (Schepers et al. 1986). Fertilizer rates should not be set on yield goals but rather on realistic goals based on research. This yield-goal practice is not recommended in Florida, so the effect of overfertilization has been avoided by grounding expectations in measurement of observed yields. In effect, the current Florida fertilizer recommendation includes a component that addresses yield expectation versus actual production capability concern expressed by growers. Scientists throughout the years have conducted many replicated demonstration studies in growers' fields with true commercial production practices, and those studies are included in this review where possible. Excessive fertilizer was justified in times past when fertilizer was viewed as inexpensive insurance against yield loss. Research with numerous crops has shown that nutrient use efficiency declines as nutrient rate, especially N, increases. Even given the best of production systems, N use efficiency typically is 50% and rarely exceeds 70% of the applied N. Concepts and practices for managing nutrients in vegetable production were summarized by Hochmuth (1992; 2000). Furthermore, it has been suggested that the yields in older studies were much lower than yields obtained today. However, there are older research reports where yields were as high as yields achieved today.
Fertilizer rates are expressed on a per-acre basis (amount of fertilizer used on a crop growing in an area of 43,560 sq. ft.). Changes in bed spacing often lead to needed changes in fertilizer amounts. For example, to maintain the same amount of fertilizer in the plant bed for a crop on 6-foot bed spacing as a crop with 4-foot bed spacing would mean an increase by a factor of 1.5 in the "per acre" rate of fertilizer for the crop growing in beds spaced 4-foot on center. The important aspect is to have the same amount of fertilizer per linear-bed-foot of bed (LBF) because it is the bedded area where most of the roots are contained. This linear-bed-foot system is used by the UF/IFAS Extension Soil Testing Laboratory to express fertilizer rates. The concept is explained by Hanlon and Hochmuth (1989) and by Hochmuth and Hanlon (2009). Snap bean fertilization is typically not provided by the LBF system but rather on an acre basis; however, information is provided to encourage banding of fertilizer whenever possible. Fertilizer rate expressions used in this review summary and its figures are those rates presented by the various authors in their research papers. Most authors express rates on a per-acre basis, irrespective of variations in row spacing among reports or experiments. We attempt to specify planting patterns and fertilizer rates for each experiment as far as we can determine from each report. Snap bean production systems have varied with time largely due to differences in irrigation systems. Hence the irrigation method is referenced for the fertilizer research studies. Today much of Florida's snap bean crop is grown with sprinkler irrigation.
Nitrogen Mixed-Fertilizer Trials
It is difficult to determine specific N, P, or K responses from trials with mixed N-P-K fertilizers. Since N is the most limiting nutrient in most farming situations in Florida, responses to fertilizer in these studies are assumed to be mostly to N.
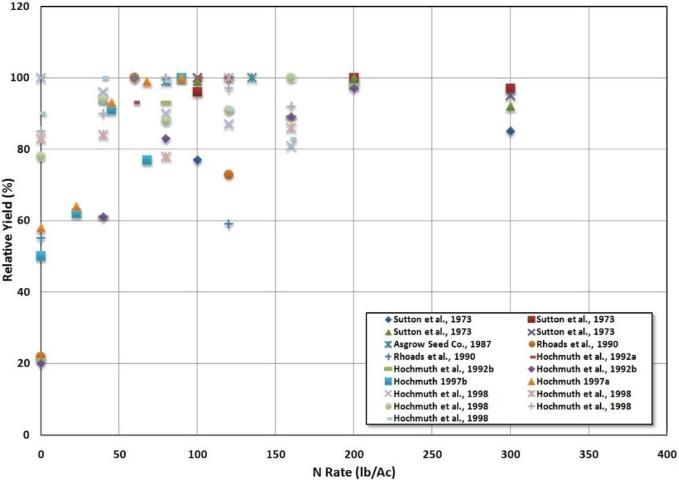
Researchers, concerned with the repeated application of soil fumigants in the prevention of root-knot nematode, conducted a three-year spring and fall season study of the effects of two fumigants on successive crop yields (Nettles 1953). Three nitrogen (N) forms and mixtures were studied simultaneously including nitrate-N, ammoniacal-N, and 1:1 nitrate:ammoniacal-N from NaNO3 and (NH4 )2SO4. Fertilizer was applied at planting by hand from a 4-7-5, N-P2O5-K2O blend, at the rate of 50 lb/acre N. An additional 24 lb/acre N was sidedressed after heavy rains in March 1951. Yield results were not obtained in the fall of 1951 and 1952 due to cold injury of plants.
Higher yields resulted from plants fertilized with the ammoniacal-N source (293 bushels/acre) compared to nitrate-fertilized plants (210 bushels/acre) in spring of 1951. Plants fertilized with ammoniacal/nitrate mixed 1:1 in spring of 1953 produced higher yields (155 bushels/acre) than those fertilized with nitrate-N (120 bushels/acre). The weight of a bushel was not specified in this study. An interaction between N sources and fumigant occurred in 1952. In all seasons, the lowest yields occurred with nitrate-fertilized plants likely due to greater leaching losses with this nutrient form than with ammoniacal-N. Annual use of soil fumigants increased yield compared to unfumigated beans and did not affect successive bean plantings. The fumigants used were dichloropropane-dichloropropene mix and 41% ethylene dibromide. Yield decreases with each successive crop were attributed to repeated bean cropping for five seasons. In the absence of fumigants and nematicides, researchers in 1963 found that NH4NO3 increased nematode activity in snap bean plants while guano-fertilized plants were least affected by nematodes (Winchester and Ozaki 1963). Snap bean yields, however, were greatest with NH4NO3 and guano and less with NaNO3, urea, or calcium nitrate.
Improvements in equipment used for fertilizer application prompted the study of the effects of fertilizer placement methods on yield of snap bean (Nettles and Hulburt 1966). Fertilizer, 6-8-8 (N-P2O5-K2O), was applied at 50, 65, or 85 lb/acre N to spring-planted beans in 1963, 1964, and 1966 near Gainesville. Five fertilizer placement methods were evaluated, including band placement 2.5 inches below the seed and to one side (single band) or to both sides of the plant row (double band) and a broadcast/band method half broadcast/incorporated in an 18-inch band with the remainder double-banded. Double-bands were also applied in varying proportions above and below the seed, termed high and low placements—with half of the fertilizer placed 2.5 inches to one side and 2.5 inches below the seed (high) and half-applied 2.5 inches to the other side and 6 inches beneath the seed (low) or one-fourth was applied 2.5 inches below the seed (high) and three-fourths applied 6 inches below the seed (low).
Snap bean yields were lower overall and were unaffected by the fertilizer placement method in 1963. Yields averaged using all placement methods were optimized with 50 lb/acre N (112 bushels/acre, 100% RY). Fertilizer placement did, however, affect yields in 1964 and 1966. Plants fertilized with the half high/half low placement method in 1964 yielded 256 bushels/acre compared to 201 bushels/acre with the broadcast/band placement method. The same broadcast/band placement method in 1966 resulted in the highest yields, 292 bushels/acre compared to 264 bushels/acre with the single- or double-band placement method. Yields in 1964 and 1966 were significantly higher, 5% probability, with 85 lb/acre N (259 and 286 bushels/acre, 100% RY, respectively) than with 50 lb/acre N, 73% and 93% RY, respectively.
Nitrogen Trials
The fertilizer N or K rate to produce the highest yield of 'Kentucky 191' pole beans was sought in the spring seasons of 1964 and 1966 and fall seasons of 1964 and 1965 in Dover, Florida (Sutton et al. 1973). Nitrogen rates applied to Scranton fine sand soils were 100, 200, or 300 lb/acre from NH4NO3. These N rates were applied factorially with four K rates to row-seeded beans in the spring seasons and hill-seeded beans in the fall seasons. Fertilizer was applied in thirds at 15, 35, and 50 days after planting for row-seeded plants and divided into two applications for hill-seeded plants—one-third applied 15 days after planting and two-thirds applied 40 days after planting. All fertilizer was placed in bands 6 inches to either side of the plant row and 4 inches deep. Sprinkler irrigation was applied as needed.
Applied N did not significantly affect yields in either the fall season or in the spring of 1966. Best yield in the spring occurred with 100 lb/acre N (205 bushels/acre, 100% RY). In the fall seasons, 100% RYs occurred with 200 lb/acre N (420 and 404 bushels/acre each season) though equally high yields also occurred with 100 lb/acre N, 96 and 99% RYs each season. Significant yield differences, 5% probability, occurred in spring 1964. Best yield this year occurred with 200 lb/acre N (260 bushels/acre, 100% RY) compared to 77% RY with 100 lb/acre N. Analysis of N concentrations in the leaf tissue revealed a lack of response to applied N in both fall 1965 and spring 1966 seasons. Late-season N concentrations in the range of 2.5% to 4% (adequate) with all rates of applied N, together with a significant (1% probability) yield-to-tissue -concentration correlation with these N concentrations (1966), led researchers to conclude that sufficient N was present for this crop with 100 lb/acre N.
Researchers with Asgrow Seed Company conducted experiments with eight snap bean varieties on commercial farms in Homestead, Florida (Asgrow Seed Co. 1987). Snap beans were spaced 1, 2, or 3 inches apart and evaluated for yield responses to 40, 80, or 135 lb/acre N and for the tendency of plants to lodge. Snap bean yields were similar with all N rates resulting in 94, 99, and 100% RY (218 unspecified containers/acre) with each respective N rate. Average yields were similar (226 containers/acre) from plants spaced 1 or 2 inches apart, but yields from plants spaced 4 inches apart were lower (186 containers/acre). Incidence of plant lodging increased with closer plant spacing. Researchers recommended the 2-inch plant spacing with 60 to 80 lb/acre N. Higher yielding varieties like 'Bronco,' 'Podsquad,' and 'Strike' had a greater tendency to lodge, and researchers suggested a 3-inch plant spacing for such varieties.
While 80% of the snap beans grown in the state are planted in the southern part of Florida, production in the north is increasing. Therefore, researchers saw a need for N fertilization research in northern snap bean production areas (Rhoads et al. 1990). Experiments were conducted with 'Strike' bush snap bean during spring 1987 and 1988 seasons at Quincy, Florida, on Norfolk loamy fine sand soils. Nitrogen at 0, 60, or 120 lb/acre was applied half at the two-leaf stage and half five weeks after planting from NH4NO3 (fertilizer placement method was not described).
Snap bean yields were optimized with 60 lb/acre each year (169 and 96 bushels/acre, 100% RYs). These yields were significantly higher (5% probability) than yields with 0 lb/acre N, 22% and 55% RYs. Tissue N concentrations two weeks before harvest in 1987 increased to 3.6% in response to N throughout the range of 0 to 120 lb/acre. Yields decreased to 73% and 59% each season with 120 lb/acre N compared to 60 lb/acre N. Based on these data, researchers concluded that excessive N reduces snap bean yields.
North Florida research continued at Live Oak in the spring of 1989 with a factorial experiment to evaluate two N rates and five fertilizer application programs (Hochmuth et al. 1992a). Nitrogen rates of 60 or 100 lb/acre from NH4NO3 were applied in bands 2 inches to each side of the seed row and 3 inches below the seed or applied broadcast to simulate fertigation through a sprinkler system. There were three fertilizer programs (equal applications): at planting; at first trifoliate leaf; and at first flower bud applied in bands only (schedule 1), banded at planting and broadcast thereafter (schedule 2), or broadcast at each application (schedule 3). Fertilizer schedules 4 and 5 were divided into 4 applications, 30% applied at planting and first bloom with 20% applied at first trifoliate leaf and first flower bud stage. All fertilizer was broadcast in these schedules except the fifth schedule in which the fertilizer was banded at planting. 'Podsquad' bean seeds were planted in rows 30 inches apart.
Marketable yields of snap beans were similar with all application programs averaging 277 bushels/acre. Leaf-tissue N concentrations increased significantly from 3.3% to 3.8% with N rates 60 to 100 lb/acre, respectively (3.0 to 4.0% is adequate; Hochmuth et al. 2009), though yields were not affected by the increase in N rate. Yields with 100 lb/acre N were 287 bushels/acre (100% RY), compared to 93% RY with 60 lb/acre N. With 100 lb/acre N, plants were larger, tended to lodge, and had more beans develop rot (38 bushels/acre) due to soil contact than those plants fertilized with 60 lb/acre N (22 bushels/acre). Researchers suspected poor root development with broadcast fertilized plants since significantly more plant lodging occurred with this placement method compared to plants that received all band-placed fertilizers.
Additional Live Oak experiments were conducted in the spring of 1989 and 1990 to evaluate yield responses to N rates, which increased in 40 lb/acre increments from 0 to 200 lb/acre (Hochmuth et al. 1992b). Klej fine sand soils were planted with 'Podsquad' seeds and fertilized with NH4NO3 that was divided in thirds and applied in a band at planting (3 inches beside the row and 2 inches deep), broadcast at the first trifoliate leaf, and broadcast at the flower bud stage each season. The soil organic matter content was 1.7% in 1990.
Marketable yields increased quadratically both years, leveling off at rates of more than 120 lb/acre N (279 and 322 bushels/acre each year, 100% RYs). Minimum N requirements, described by the linear-plateau model, were 73 lb/acre in 1989 and 92 lb/acre in 1990 with r2 values of 0.83 and 0.64 each respective year. The quadratic model predicted optimum yield with 150 lb/acre N and an r2 of 0.66. Leaf-tissue N concentrations increased linearly to 4.4% with the highest N rate in 1989 and quadratically in 1990. With plateau N rates of 73 and 92 lb/acre, corresponding leaf tissue N concentrations of 3.6 and 3.8% were in the adequate range of 3.5 to 4.0% at full-bloom sampling (Hochmuth et al. 1991; 2009). Marketable yields failed to increase with more than 120 lb/acre, but at this N rate, plant lodging became increasingly severe in both seasons, and yield losses above 20 bushels/acre resulted from decay after bean contact with the ground. Other quality characteristics were affected by higher N rates in 1989, but not in 1990, such as increased incidence of broken and pin (undersized) pods (58 bushels/acre with 120 lb/acre N) and increased incidence of curved pods with higher N rates.
Researchers experimented with the humate compound "Grow-Plex SP" (Earthgreen Products Inc., Dallas, TX) and rates of N in the spring (Hochmuth 1997a) and fall of 1997 (Hochmuth 1997b). Researchers assessed the effects of humate and N on early plant vigor and yield in both experiments conducted at the University of Florida Horticultural Research Unit near Gainesville. All plants fertilized with N received 20 lb/acre NH4NO3-N broadcast and incorporated preplant in a raised bed as a portion of the total applied N. The balance of the applied N to reach total rates of 0, 23, 45, 68, or 90 lb/acre was applied as a side-dress application. Total N rates were calculated as 0, 25, 50, 75, or 100% of the 90 lb/acre recommended N rate.
In spring 1997, seed furrows (two per bed with beds on 4-ft centers) were sprayed with Grow-Plex SP humate in suspension with water and Earthgreen Synfactant at rates of 0, 1, or 2 lb/acre humate. 'Caro' snap bean seeds were planted, covered immediately, and wet with overhead irrigation. Side-dressed fertilizer was applied in a band between the double rows at first trifoliate leaf and when plants were 4 inches tall. Soil moisture was maintained at -10 cb using tensiometers and overhead irrigation. Due to a loss of 20% of the plots from an overspray of herbicide, an analysis of variance was not performed on the data. Early plant vigor was rated from 1 to 5 for yellow to dark green before emergence of the trifoliate leaf. Results were similar for all humate treatments averaging a 3.7 vigor rating. Yields were similarly unaffected by humate treatment averaging 227 bushels/acre with 0 to 2 lb/acre humate. Yields were similar with 68 lb/acre N or the recommended 90 lb/acre N—272 and 276 bushels/acre (100% RY), respectively.
In fall 1997, humate immersed in water was sprayed in furrows seeded with 'Seville' snap beans. Humate and N rates were applied as in the spring with two foliar applications of 0, 1, or 2 lb/acre humate made at first flower bud and again at full flower with the 68 lb/acre N treatment only. Two fertilizer side-dress applications, timed as before, were broadcast between the seed rows and incorporated with a rake this season. Overhead irrigation was maintained as in the spring. Foliar application of humate late in the season had little effect on plant vigor compared to those plants that received humate in the furrow only with the 68 lb/acre N treatment. The overall effects of humate on snap bean yield were minimal; although responses to humate were noted with lower rates of applied N, optimum yield occurred with 90 lb/acre N (234 bushels/acre, 100% RY), regardless of the humate applied at planting. Significant, 1% probability, yield differences resulted with increasing N rates as yield doubled with the application of 90 lb/acre N compared with the 0 lb/acre N treatment.
Snap bean fertilization experiments were conducted each winter from 1993 to 1995 in Homestead, Florida (Hochmuth et al. 1998). Five studies were conducted (one in 1993–1994 and two each in 1994–1995 and 1995–1996 seasons) on Rockdale soils. Nitrogen was applied by banding to side of row at plant emergence (50% of total N) and again when plants were 2 to 3 inches tall (50%). Snap beans were planted in rows approximately 30 inches apart and irrigated by overhead sprinkler (portable gun or linear-move system). Nitrogen was applied from 0 to 160 lb/acre in increasing 40 lb/acre increments. Yields did not respond to N fertilization in three experiments in which average yields were 234, 281, and 368 bushels/acre. In other seasons, N reduced yields (5% probability) from 100% RY with zero lb/acre N (252 bushels/acre) to 81% RY with 160 lb/acre N, or N increased yields (5% probability) from 78% RY with zero lb/acre N to 100% RY (234 bushels/acre) with 160 lb/acre N. Factors generally not affected by N fertilization were plant stand, yields of rotten pods, and yields of misshapen snap beans. Nitrogen reduced (1% probability) the yield of undersized beans in one experiment and had an effect on small snap bean yields in two other experiments; however the responses did not fit a specific trend. Researchers in these experiments noted that, at lower N rates, fruit set increased, but fewer fruits reached maturity. Yield responses with the grower treatments, ranging from 70 to 100 lb/acre N, were similar to those with the experimental N rates. Leaf N concentrations were sufficient or above sufficiency with all treatments in all experiments.
Nitrogen Summary
Research with N fertilization of snap beans was conducted mainly in the northern and central regions of the state during the spring planting season (about two-thirds of the research) with the remaining one-third of research conducted in the southern region where most of snap bean production occurs. Yields were generally optimized with N rates between 60 and 100 lb/acre. In experiments where N was applied at rates greater than 100 lb/acre, yields either were not affected, optimized between 120 and 200 lb/acre in 30% of experiments, or decreased in three experiments. Nitrogen rates at 100 lb/acre and greater resulted in larger plants with a greater tendency to lodge and produce more rotten beans due to ground contact. Plant lodging could be reduced with heavier yielding varieties like 'Bronco,' 'Podsquad,' and 'Strike' if plants were spaced 3 inches apart. Optimum N rates, as determined by a linear-plateau model, were 73 and 92 lb/acre in two experiments at Live Oak, Florida. Snap bean plants were negatively affected by broadcast-placed fertilizers. A greater tendency toward plant lodging resulted with broadcast-fertilized plants, possibly due to poorer root development, compared to band-fertilized plants. With this unmulched crop, researchers recommended split-fertilizer applications, part applied at planting and the remaining N side-dressed in bands at least once early in the growing season. Plants fertilized with ammonium-containing N fertilizer produced higher yields, compared to fertilizers made from 100% NO3-N.
Phosphorus and Potassium Soil Testing
Knowledge of soil nutrient levels, particularly P and K, before planting is the starting point to predicting crop response to varying rates of applied nutrient. Using soil testing to determine preplant soil nutrient concentrations provides information so research results may be reviewed for the degree of support of existing fertilization recommendations. The M-1 (double-acid) solution is the current extractant used by Florida and several other Southeastern US states for sandy soils.
M-1 extractant indices (expressed as ppm soil-extracted nutrient) are classified as very low, low, medium, high, and very high; and a crop-specific fertilizer recommendation is made from that classification (Hochmuth and Hanlon 1995a; Mylavarapu 2009). The M-1 solution became the accepted extractant standard in 1979 at UF. Previous to M-1, ammonium acetate and water extractants were used. Indices recorded from these methods cannot be directly equated with M-1 indices or fertilizer recommendation rates, but review of research results from studies with these extractants presents a profile of crop response to fertilizer with varying conditions. The effects of water management, fertilizer source, fertilizer placement, and time of application on the nutrient management system should also be considered in P and K fertilization programs.
Phosphorus
Responses of snap bean to P fertilization are summarized in Figure 2. Plant P uptake and yield were evaluated following application of five P fertilizers at P2O5 rates of 0, 45, 90, or 140 mg/2.2 lb of soil (0, 91, 182, or 272 lb/acre P2O5) (Rhoads 1991). The experiment with 'Strike' bush beans occurred in the spring of 1988 in Quincy at the North Florida Research and Education Center (NFREC). Plants were grown in a glasshouse in pots filled with 13 lb of Norfolk loamy fine sand soil taken from the Ap horizon. Soils were considered deficient in P, and an M-1 soil test of the check treatment after harvest resulted in 2.5 ppm P—a very low soil P concentration. Researchers sought to evaluate yield responses to ammonium-containing P fertilizers (ammonium polyphosphate [APP], monoammonium phosphate [MAP], and diammonium phosphate [DAP]) with calcium-containing P fertilizers (superphosphate [SP] and concentrated superphosphate [CSP]).
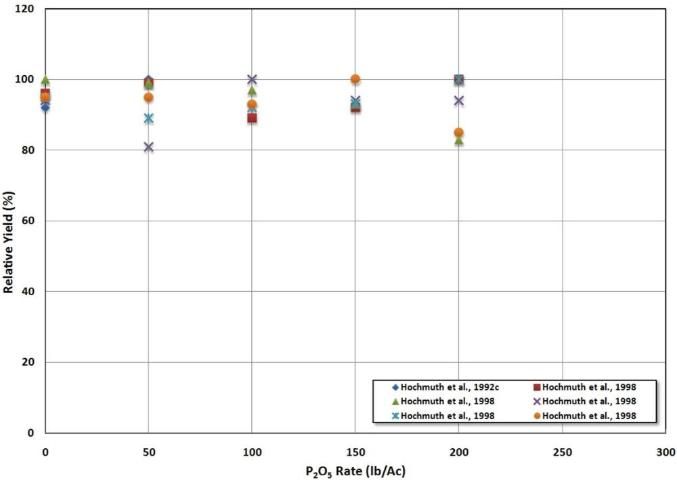
Plant P uptake (the product of dry matter yield of five-week-old plants and tissue-P concentration) was greatest with APP-fertilized plants, intermediate with OSP- and TSP-fertilized plants, and least with MAP- and DAP-fertilized plants. Residual soil P concentrations at harvest reflected plant P uptake. The lowest residual M-1 P soil concentration, the greatest P uptake, and the highest dry matter yield were from APP-fertilized plants where average dry matter yield was 7 times higher than with the zero P check treatment. High dry matter yields with all treatments occurred with 140 mg/kg of P2O5. Significantly lower plant P uptake, highest residual M-1 soil P, and a low average dry matter yield (only 3 times more than dry matter yield with the check treatment) occurred with DAP-fertilized plants. Plants fertilized with P sources MAP, OSP, and TSP resulted in average dry matter yields 4, 5, and 7 times greater, respectively, than dry matter yield of plants with the check treatment. Researchers concluded that P availability from MAP and DAP was 50% lower than with other P sources.
In the spring of 1991 at the same Quincy site, P research continued with 'Strike' glasshouse-grown bush beans in pots with 4.4 lb of Norfolk loamy fine sand soil (Ap horizon) (Rhoads et al. 1993). A remaining question was the effect of soil on the availability of P. Application of P in forms unavailable to the crop presents an environmental concern in situations where these fertilizers can potentially be leached or carried off by erosion to lakes and streams. Researchers chose TSP, which was found in the previous experiment to be a readily available P fertilizer, and DAP, which was found to be a less available P-fertilizer source. Calcite lime (fine) was applied at 2 grams/2.2 lb of potted soil either four weeks before P application or four weeks after P application. Eighteen separate treatments—including P2O5 rates of 90, 180, or 280 mg/2.2 lb of soil; two P sources; and lime applied before or after P application—were used to determine the effect of lime reaction with the soil or P reaction with the soil on P availability from TSP or DAP. All P treatments were incubated for four weeks with moist soil before planting. No P was applied in two additional treatments on limed and unlimed soil.
The shoots and roots of plants were collected 6.5 weeks into the season and dried to a constant weight. Plants fertilized with TSP at the highest P rate had greater than 100% more dry-shoot matter than DAP-fertilized plants and had 70% higher shoot P concentrations averaged using all lime and P treatments compared to DAP-fertilized plants. Addition of lime before TSP application, with the highest P rate, increased P uptake 16% compared to plants that received lime after TSP application. Lime applied before DAP, with the highest P rate, increased P uptake in shoots 85% compared with plants that received lime after DAP application. As with the previous study, TSP supplied more available P than DAP.
No P fertilizer was applied to plots containing soil of varying M-1 soil P concentrations during spring 1987 and 1988 field experiments conducted in Quincy, NFREC (Rhoads et al. 1990). 'Strike' snap beans were planted in April each year, and yield and postharvest plant tissue P concentrations were analyzed to determine the optimum soil P concentration required for bush snap bean on Norfolk loamy fine sand soils. Preplant soil-P concentrations in the test plots determined by M-1 soil test were 7, 11, 29, and 66 ppm P in 1987 and 7, 12, 21, and 42 ppm in 1988. One ton/acre of calcitic lime was applied in February 1988.
Snap bean yield response was quadratic in 1987 resulting in peak yield with 29 ppm M-1 soil-extracted P (169 bushels/acre, 100% RY). In 1988, yields were 40% lower and responded linearly to soil test P concentrations through 42 ppm extracted P (100 bushels/acre, 100% RY) with 96% RY with 21 ppm soil P. Researchers suspected low soil P had limited yields in 1988 based on soil test P concentrations that were lower in the later two samples than the previous season. Application of lime in the second season was also cited for lower P availability due to the formation of calcium phosphate at higher pH. Yield data for these experiments accurately represented the current breaking point between soil test P interpretations of medium to high soil P concentrations (Hochmuth and Hanlon 1995a b; Kidder et al. 1989). Optimum yields occurred at extractable values more than 30 ppm P, and lower yields occurred with soil P indices less than 30 ppm. Whole-plant tissue P concentration was correlated with yield in 1987 but not in 1988.
Phosphorus recommendations were field tested near Live Oak at the Suwannee Valley Agricultural Research and Education Center (SVREC) on Klej fine sand soils where M-1 soil P concentrations averaged 97 ppm, and soil pH was 6.8 (Hochmuth et al. 1992c). These soil P concentrations were interpreted as very high, and no additional P was recommended (Kidder et al. 1989). To test this recommendation, P was either not applied or applied at planting in a band 2 inches to the side of the row of 'Podsquad' beans and 3 inches deep at 50 lb/acre P2O5 from TSP. Application of P did not affect bean yield on these very high P soils. In addition, bean length, condition (broken or rotten), or tendency toward plant lodging were not affected by added P. Yields were not different with 0 lb/acre P2O5, 93% RY, or 50 lb/acre P2O5 (226 bushels/acre), 100% RY. These results supported the recommendation of 0 lb/acre P2O5 for soils very high in extracted P.
Field experiments were conducted on two commercial farms near Homestead during the winter seasons of 1994 through 1996 (Hochmuth et al. 1998). Snap beans were grown on Rockdale soil and were sprinkler irrigated by portable gun or linear-move systems. All P from TSP was applied in a band to one side of the row at plant emergence. Soil P concentrations extracted with AB-DPTA solution before fertilization ranged from 56 to 113 ppm and were considered adequate for optimum yields. Phosphorus at rates of 0, 50, 100, 150, or 200 lb/acre P2O5 had no effect on yields in two experimental seasons. Average yields for these seasons were 216 and 252 bushels/acre. Yields increased slightly in two other experimental seasons (5% probability) from zero to 200 lb/acre P2O5 (296 to 312 bushels/acre, 100% RY) and from zero to 100 lb/acre P2O5 (228 to 242 bushels/acre, 100% RY). Yields decreased (5% probability) in a fifth season from 95% RY (381 bushels/acre) with zero lb/acre P2O5 to 85% RY with 200 lb/acre P2O5. Yields from these experiments are graphed in Figure 2. Phosphorus did not affect yields of rotten or misshapen bean pods in any experimental season and did not affect yield of undersized pods, except in one season where fewer small pods were produced with P at 200 lb/acre P2O5. Researchers suspected P enhanced maturity or acted to reduce fruit set and to allow existing pods to develop. Additional work was suggested on the effect of P fertilization on growth, fruit set, and maturity of snap beans.
Phosphorus Summary
Research with P fertilization of snap bean was conducted near Quincy and Live Oak in Northern Florida and in the Homestead area. Yields did not respond to added P on soils with high and very high M-1 soil-extracted P. These results concurred with the IFAS recommendation of zero lb/acre P2O5 on these soils (Hochmuth and Hanlon 1995; Kidder et al. 1989). Snap bean yields increased slightly with P fertilization at two Homestead, Florida, locations where AB-DPTA soil-extracted P concentrations were not available or were 81 ppm. Yield at a third Homestead location decreased with P fertilization where soil P concentrations were 56 ppm. In other Homestead experiments, yield did not respond to applied P when AB-DPTA soil-extracted P concentrations were 113 ppm or 91 ppm. Experiments were not conducted to test snap bean yield response to applied-P fertilizer on soils very low, low, and medium in extracted P because these low-P soils were not found in the commercial snap bean farms of Dade County. Phosphorus recommendations (Hochmuth and Hanlon 1995) for these soil P concentrations were not tested in field research. Earlier research with pot-grown snap beans stressed the application of fertilizer sources containing readily available P. Plant P uptake and yield were highest with APP and TSP and lowest with DAP. Phosphorus not absorbed by plants can potentially be carried off by soil erosion to pollute water systems. Application of lime four weeks before P application increased P availability from DAP, but TSP remained a more readily available P source with or without early lime application. Researchers suggested additional study on the effect of P fertilization on growth, fruit set, and maturity of snap beans based on results from an experiment where reduced yield of undersized beans occurred with added P.
Potassium
Snap bean responses to potassium fertilization are presented in Figure 3. Nitrogen and K were applied factorially in the spring seasons of 1964 and 1966 and fall seasons of 1964 and 1965 to Scranton fine sand soils in Dover (Sutton et al. 1973). Yields of 'Kentucky 191' pole beans were evaluated for spring 1964 and 1966 seasons and fall 1964 and 1965 seasons following factorial applications of three N rates and three K rates—113, 224, and 336 lb/acre K2O from KCl. Fertilizers were applied in thirds to row-seeded beans in the spring, at 15, 35, and 50 days after planting and divided in two applications with hill-seeded beans in the fall seasons, one-third 15 days after planting and two-thirds 40 days after planting. All fertilizer was placed in bands 6 inches to either side of the plant row and 4 inches deep. Overhead irrigation was applied as needed.
Yields were not affected by K rate in any season except the fall of 1965 (Figure 3). High yield this season occurred with 113 lb/acre K2O (463 bushels/acre, 100% RY) and decreased to 90% RY with 336 lb/acre K2O, significant at 1% probability. A significant negative correlation, -0.479, resulted between leaf-tissue K concentrations sampled at the last half of the harvest period and yield. Researchers suspected soluble salt concentrations 3 to 4 times higher in 1965 than 1966 may have contributed to the yield reduction that season. High yields in other seasons (though yields were similar with all K rates) occurred with 113, 336, and 224 lb/acre K2O (269, 483, and 235 bushels/acre, 100% RYs) in the spring and fall of 1964 and the spring of 1966, respectively.
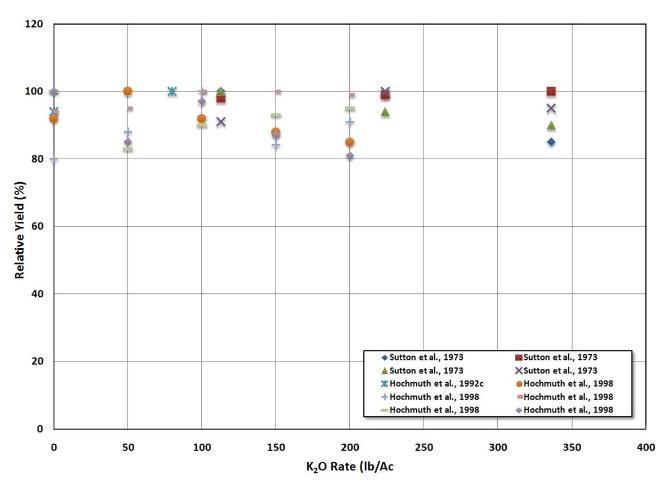
Potassium research was conducted on Norfolk loamy fine sand soils at Quincy, UF/IFAS NFREC, in 1987 and with 0, 85, or 170 lb/acre K2O from K2SO4 applied preplant in 1988 (Rhoads et al. 1990). Yield responses were evaluated based on M-1 soil-extracted K concentrations, which were tested before planting (February 1987) and after planting and fertilization (April 11, 1988). Yield responses in both seasons were linear, increasing through 73 and 78 ppm M-1 soil-extracted K each season (169 and 101 bushels/acre, 100% RY, respectively). These K concentrations are currently interpreted as high and no additional K fertilizer would be recommended (Hochmuth and Hanlon 1995a). Yields in both Quincy experiments were optimized with higher soil K concentrations. Response to added K fertilizer also occurred in the plant tissue. There was greater correlation between snap bean tissue K concentration and soil K concentration in 1988—0.90 compared to a 0.60 correlation between plant tissue and soil K in 1987 when no additional K fertilizer was applied. Although added K increased the concentration of K, as measured in the plant tissue in 1988, overall yields were lower in 1988 than in 1987.
Snap bean yields in the above experiments required greater amounts of soil K than those cited by IFAS. However, snap bean in a spring 1989 experiment near Live Oak (SVAREC) required less than the recommended K rate for optimum yields (Hochmuth et al. 1992c). A potassium recommendation of 80 lb/acre K2O was made (Kidder et al. 1989) for these Klej fine sand soils, which tested low (24 ppm) for M-1 soil-extracted K. Potassium treatments were 0 or 80 lb/acre K2O from KMgSO4 applied preplant in a band 2 inches beside the row and 3 inches deep. 'Podsquad' snap beans were planted on April 19. Snap bean yields responded similarly to both K treatments with 80 lb/acre K2O (226 bushels/acre), 100% RY, compared to 94% RY with 0 lb/acre K2O. Researchers concluded that more research is needed with K fertilization of snap bean. Bean quality characteristics—straightness, length, broken, and empty or rotten pods—were all unaffected by the addition or omission of K fertilizer. The tendency of plants to lodge was also unaffected by K fertilization.
Experiments were conducted in Homestead, Florida, to evaluate yield responses to K rates of 0, 50, 100, 150, or 200 lb/acre K2O (Hochmuth et al. 1998). Soils used for all K studies were Rockdale soils on commercial farms with sprinkler irrigation (portable gun or linear-move). Potassium from KCl was banded to the side of the row, 50% at plant emergence and 50% when plants were 2 to 3 inches tall (with the second N application). Soil K concentrations, tested with AB-DPTA soil extractant, ranged from 71 to 281 ppm and were considered adequate for optimum yield without additional K. Yields in three of the experiments were similar with K rates from 0 to 200 lb/acre, while yields decreased in a fourth experiment and increased slightly in a fifth experiment with added K. Yields of small, rotten, or misshaped beans were not affected by K fertilization in any experiment nor were plant stands affected by increased K. Whole-leaf K concentrations exceeded the sufficiency range in all experiments and occasionally increased with K fertilization.
Potassium Summary
Snap bean yields were not always affected by K fertilization at K rates through 336 lb/acre K2O. Yields were not always optimized with the recommended K rate based on M-1 soil test, at times requiring more than the recommended rate and at other times less than the recommended rate. This response behavior could be related to the mobility of the K ion in sandy soils, thus making K fertilizer prediction difficult. Potassium rate recommendations were increased for soils very low, low, and medium in M-1 soil-extracted K from 80, 80, and 60 lb/acre K2O (1989) to 120, 100, and 80 lb/acre K2O (1995), respectively. Yield responses appear to fall as K rates exceed the recommended 120 lb/acre K2O, and 70% of the experiments were not affected by increased K. Yields decreased with added K in 20% of experiments, and yields were optimized with 100 lb/acre K2O in 10% of the experiments. Based on unpredictable yield responses to residual soil K concentrations and K applied as fertilizer in the above summarized experiments, more research is needed on K fertilization of snap bean. Yields of undersized, rotten, or misshapen snap beans were not affected by increased K fertilizer nor were plant stands affected by increased K. As with all unmulched and overhead-irrigated crops, split-N and split-K application was recommended due to the leaching potential of these mobile nutrients. Researchers recommended preplant application of 25% to 50% of the N and K fertilizer, with the remaining N and K fertilizer applied in side-dress bands early in the season.
Summary
For snap beans, no yield advantage resulted from application of N in excess of recommended rates in experiments in North Florida. Plant lodging increased with N application when N rates exceeded 100 lb/acre due to larger plant size with high N. Yield losses occurred when beans touched the soil and developed rot. The tendency toward plant lodging increased with broadcast N application compared with plants fertilized with band-applied N. Statistical analysis and leaf tissue correlation with yield resulted in a range of optimum N rates between 73 to 100 lb/acre, which approximated the 90 lb/acre N currently recommended by IFAS for this crop. With N and K fertilization, researchers recommended split application of these nutrients—part applied preplant and part as an early-season side-dress due to leaching with this unmulched, overhead-irrigated crop.
Phosphorus soil concentrations of 16 to 30 ppm, measured by the M-1 soil test, were sufficient to produce optimum yields in two experiment seasons, though IFAS recommends addition of 80 lb/acre P2O5 for soils with these P concentrations. Snap beans grown on soils with P concentrations greater than 30 ppm M-1 soil P produced optimum yields without added P fertilizer confirming IFAS recommendations. Additional research with P fertilization of snap bean is needed on soils with very low and low M-1 P concentrations. Research is also needed at larger commercial scale farms to test nutrient and irrigation management programs in the commercial setting.
Yields of snap bean plants fertilized with K were generally not significantly affected by increases in rates of this nutrient at more than those currently recommended. Researchers recommend no K application where soil K concentrations exceed 60 ppm as tested by M-1 extractant, but in two experiments, snap bean yields responded to soil K concentrations through 73 and 78 ppm M-1 soil K. Additional research is needed with this highly mobile nutrient to determine K rate recommendations for snap bean.
Literature Cited
Asgrow Seed Co. 1987. Fresh market beans. Nitrogen and spacing response. Misc. publ. WBC/5-19-87.
Black, C. A. 1992. Soil fertility evaluation and control. Lewis Publishers, Boca Raton, FL. 746 pp.
Brown, J. R. 1987. Soil testing: sampling, correlation, calibration, and interpretation. Soil Science Society of Amer. Special Publ. No. 21. Soil Science Society of America, Inc. Madison, Wisc.
Florida Dept. of Agriculture and Consumer Services. 2005. Water quality/quantity best management practices for Florida vegetable and agronomic crops. http://www.floridaagwaterpolicy.com/PDF/Bmps/Bmp_VeggieAgroCrops2005.pdf.
Florida Agriculture Statistical Bulletin. 2009. Season vegetables highlights, https://www.nass.usda.gov/Statistics_by_State/Florida/Publications/Annual_Statistical_Bulletin/2009/E1thru20Veg-2009.pdf [Accessed October 2022].
Hanlon, E. A., and G. J. Hochmuth. 1989. Calculating fertilizer rates for vegetable crops grown in raised-bed cultural systems in Florida. Fla. Coop. Ext. Serv. Spec. Series SS-SOS-901.
Hochmuth, G. J. 1988. Commercial Vegetable Fertilization Guide. Fla. Coop. Ext. Serv. CIR 225C.
Hochmuth, G. J. 1992. Concepts and practices for improving nitrogen management for vegetables. HortTechnology. 2 (1): 121-125.
Hochmuth, G. J. 1996a. Vegetable fertilization pp. 3-17. IN: G. Hochmuth and D. Maynard (eds.) Vegetable production guide for Florida. Fla. Coop. Ext. Serv. Circ. SP 170.
Hochmuth, G. J. 1996b. Commercial Vegetable Fertilization Guide. Fla. Coop. Ext. Serv. CIR 225D.
Hochmuth, G. J. 1997a. Response of sweet corn and snapbean to Growplex humate. Fla. Agr. Expt. Sta. Res. Rep., Suwannee Valley AREC 97-7.
Hochmuth, G. J. 1997b. Snapbean and sweet corn response to N rate and furrow-placed Growplex humate. Fla. Agr. Expt. Sta. Res. Rep., Suwannee Valley AREC 97-21.
Hochmuth, G., E. Hanlon, S. O'Hair, J. Carranza, and M. Lamberts. 1998. Report to S. Fla. Water Mgt. Dist. (draft).
Hochmuth, G. J. 2000. Management of nutrients in vegetable production systems in Florida. Soil and Crop Sci. Soc. Fla. Proc. 59:11-13.
Hochmuth, G. J., and K. Cordasco. 2000 (reviewed in 2008). A summary of N, P, and K research with snap bean in Florida. Fla. Coop. Ext. Serv. HS756.
Hochmuth, G. J., and E. A. Hanlon. 1989. Commercial vegetable crop nutrient requirements. Fla. Coop. Ext. Serv. Circular 806.
Hochmuth, G. J., and E. A. Hanlon. 1995a. IFAS standardized fertilization recommendations for vegetable crops. Fla. Coop. Ext. Serv. Circ. 1152.
Hochmuth, G. J., and E. A. Hanlon. 1995b. Commercial vegetable crop nutrient requirements in Florida. Fla. Coop. Ext. Serv. SP 177.
Hochmuth, G. J., and E. A. Hanlon. 2000. IFAS Standardized fertilization recommendations for vegetable crops. Fla. Coop. Ext. Serv. Circ. 1152. https://nutrients.ifas.ufl.edu/nutrient_pages/BSFpages/PIndices/cv00200.pdf [Accessed May 10, 2010].
Hochmuth, G. J., and E. A. Hanlon. 2009. Calculating recommended fertilizer rates for vegetable crops grown in raised-bed, mulched cultural systems. Fla. Coop. Extension Serv. Circ. SL 303. https://edis.ifas.ufl.edu/pdffiles/ss/ss51600.pdf.
Hochmuth, G. J., and E. A. Hanlon. 2010a. Commercial vegetable fertilization principles. Fla. Coop. Extension Serv. Cir. SL 319. https://edis.ifas.ufl.edu/pdffiles/cv/cv00900.pdf.
Hochmuth, G. J., and E. A. Hanlon. 2010b. Principles of sound fertilizer recommendations. Fla. Coop. Extension Serv. Cir. SL 315.
Hochmuth, G. J., E. A. Hanlon, S. O'Hair, J. Carranza, and M. Lamberts. 1998. Report to South Florida Water Management District. Unpublished.
Hochmuth, G. J., B. Hochmuth, and E. A. Hanlon. 1992a. Comparison of various N scheduling methods for snapbeans. Fla. Agr. Expt. Sta. Res. Rep., Suwannee Valley AREC 92-5.
Hochmuth, G. J., B. Hochmuth, and E. A. Hanlon. 1992b. Response of snapbean to nitrogen fertilization on a sandy soil. Fla. Agr. Expt. Sta. Res. Rep., Suwannee Valley AREC 92-6.
Hochmuth, G. J., B. Hochmuth, and E. A. Hanlon. 1992c. Field test of IFAS P and K recommendations for snapbeans. Fla. Agr. Expt. Sta. Res. Rep., Suwannee Valley AREC 92-15.
Hochmuth, G. J., D. Maynard, C. Vavrina, and E. A. Hanlon. 1991. Plant tissue analysis and interpretation for vegetable crops in Florida. Fla. Coop. Ext. Serv. Spec. Ser. SS-VEC-42.
Hochmuth, G. J., D. Maynard, C. Vavrina, E. A. Hanlon, and E. H. Simonne. 2009. Plant tissue analysis and interpretation for vegetable crops in Florida. Fla. Coop. Ext. Serv. Fact Sheet HS 964, https://edis.ifas.ufl.edu/publication/ep081.
Kidder, G., E. A. Hanlon, and G. J. Hochmuth. 1989. IFAS standardized fertilization recommendations for vegetable crops. Fla. Coop. Ext. Serv. Spec. Ser. SS-SOS-907.
Montelaro, J. 1978. Commercial vegetable fertilization Guide. Fla. Coop. Ext. Serv. Circ. 225-B.
Mylavarapu, R. S. 2009. UF/IFAS Extension Soil Testing Laboratory (ESTL) Analytical Procedures and Training Manual. Fla. Coop. Ext. Serv. Circ 1248. https://doi.org/10.32473/edis-ss312-2009.
Nettles, V. F. 1953. Yield responses of beans to repeated use of soil fumigants and three sources of nitrogen. Proc. Amer. Soc. Hort. Sci. 62:320-324.
Nettles, V. F., and W. C. Hulburt. 1966. Effect of placements and levels of fertilizer on the yield of vegetables. Proc. Fla. State Hort. Soc. 79:185-191.
Olson, S. M., and E. H. Simonne (eds.). 2010. The Vegetable Production Handbook. Fla. Coop. Extension Serv. https://edis.ifas.ufl.edu/entity/topic/vph.
Olson, S. M, B. Santos. 2010. Vegetable Production Handbook for Florida. Univ. of Fla. IFAS Extension. https://ufdcimages.uflib.ufl.edu/UF/00/09/91/59/00001/Binder1.pdf [Accessed October 19 2022].
Olson, S. M., E. H. Simonne, W. M. Stall, S. A. Webb, S. Zhang, and S. Smith. 2009. Legume production in Florida: snap bean, lima bean, southern pea, and snow pea. Fla. Coop. Ext. Serv. Cir. HS 727. https://edis.ifas.ufl.edu/pdffiles/cv/cv12500.pdf.
Rhoads, F. M., E. A. Hanlon, Jr., S. M. Olson, and G. J. Hochmuth. 1990. Response of snapbean to N-rates and soil-test P and K. Soil Crop Sci. Soc. Fla. Proc. 49:10-13.
Rhoads, F. M. 1991. Phosphorus source, soil-test P, snapbean growth, and P uptake. Soil Crop Sci. Soc. Fla. Proc. 50:122-124.
Rhoads, F. M., E. A. Hanlon, and S. M. Olson. 1993. Phosphorus availability to snap beans as affected by lime sequence and phosphorus source. Soil Crop Sci. Soc. Fla. Proc. 52:90-94.
Schepers, J. S., K. D. Frank, and C. Bourg. 1986. Effect of yield goal and residual nitrogen considerations on nitrogen fertilizer recommendations for irrigated maize in Nebraska. J. Fertilizer Issues 3:133-139.
Simonne, E. H., and G. J. Hochmuth. 2010. Soil and fertilizer management for pepper production in Florida. Vegetable Production Handbook. Fla. Coop Extension Serv. https://edis.ifas.ufl.edu/pdffiles/cv/cv10100.pdf.
Stall, W. M. (ed.). 1983. Snap bean production in Florida. Fla. Coop. Ext. Serv. Cir. 100.
Sutton, P., E. E. Albregts, and C. M. Howard. 1973. Influence of N and K on pole bean (Phaseolus vulgaris L.) response and soil and plant analysis. Soil Crop Sci. Soc. Fla. Proc. 32:136-137.
Winchester, J. A., and H. Y. Ozaki. 1963. Some effects of nitrogen source on beans and peppers growing in root-knot infested soil. Proc. Fla. State Hort. Soc. 76:163-168.