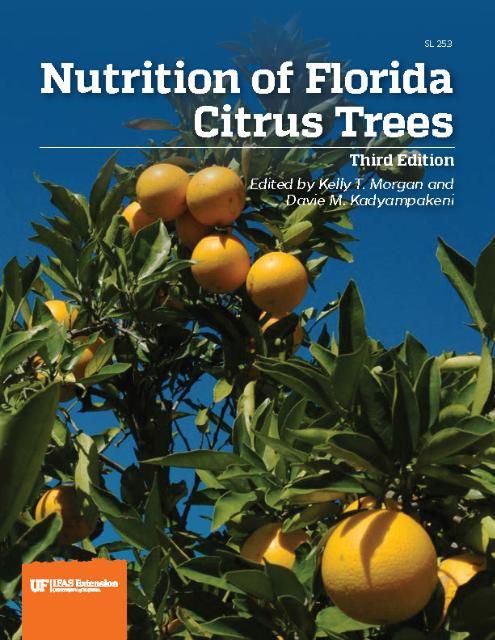
This publication is part of SL253, Nutrition of Florida Citrus Trees, 3rd Edition. For references, a glossary, and appendices, please refer to the full document at https://edis.ifas.ufl.edu/ss478.
Information in the box below applies to citrus trees affected by HLB. Other information in this chapter is valid for healthy citrus trees and trees with HLB.
Recommendations for HLB-affected trees:
Nutrient Management—Kelly Morgan, Davie Kadyampakeni, Tripti Vashisth, and Mongi Zekri
Leaf chlorosis develops as a result of infection with CLas, including interveinal chlorosis of young leaves. Symptoms are similar to Mn and Zn deficiencies that develop early in the growing season, followed by blotchy mottling of older leaves that develops later in the growing season. Leaf analysis of HLB-affected trees indicate deficiency of K, P, Mg, Ca, Mn, Zn, and Fe. HLB causes fibrous roots to decline within a few months after infection and before foliar symptoms develop. Fibrous roots are responsible for the bulk of nutrient uptake, and their decline likely explains the deficiency symptoms that develop in the canopy.
Traditionally, citrus growers try to achieve optimum nutrition through fertilizer management. A five-year study of foliar applications of Mn, Zn, and B on 5-to-7-year-old Valencia trees on Swingle rootstock was recently concluded in a commercial grove with the goal of determining the effect of improved leaf nutrient status on canopy density and yield (Morgan et al. 2016)*. The first analysis conducted was to determine whether foliar application of potassium nitrate (KNO3) affected foliar concentrations of N and K and growth and productivity of the trees. The lack of an increase in foliar N after application suggests that N moves from the mature leaves to the new growth. Unlike leaf N, foliar K concentration of K-deficient trees increased to the optimum range after KNO3 application. The application of KNO3 increased canopy volume compared to the controls. However, yields for KNO3-treated trees were not significantly greater than yields for the controls. One interesting result of this study was that the amount of Mn and Zn taken up into the leaf was not affected by KNO3, as some have speculated.
Mn, Zn, and B were applied to trees separately at three rates, plus nonsprayed controls. The three rates were 0.5, 1.0, and 2.0 times the current UF/IFAS foliar recommendations (Table 7). The nutrient sprays were applied three times per year following flushes in March, May, and September. Thus, the three rates (0.5, 1.0, and 2.0 times UF/IFAS) resulted in a total of 1.5, 3.0, and 6.0 times UF/IFAS recommendations on an annual basis. For example, the UF/IFAS recommendation for Mn and Zn is five pounds per acre per year, so trees receiving 3 times UF/IFAS recommendation would receive 15 pounds per acre per year. The highest rates of Mn and Zn application had the greatest increase in those foliar nutrients. Although increases in leaf Mn and Zn concentration were observed immediately after application, no differences were found compared with controls prior to the next foliar application. Because Mn and Zn are mobile in plants, it is suggested these nutrients move out of the leaves to growing points. Canopy volume increased with increased application of Mn and Zn, but not B. Yield increased with the 1.5 and 3.0 annual rates of Mn and Zn but was lower for the 6.0 rate compared with the 3.0 rate. These results indicate increased growth of trees proportional to Mn and Zn within the range tested but reduced yield at the highest rate. Reduced yield at high rates of nutrients is common, because excess nutrient results in increased growth at the expense of yield and could partially explain variability in tree response to nutrient applications by citrus growers.
Conclusions from the five-year study indicate that the optimum range of leaf Mn and Zn concentrations should be in the upper half of the current recommended range (Table 7). However, the current maximum optimum range should not be exceeded unless larger and/or denser tree canopies are desired at the risk of lower yields. The new suggested leaf concentration ranges can be found in the table below.
Research has demonstrated that HLB symptoms can be reduced by foliar applications of micronutrients, especially Mg, Mn, and Zn. However, foliar nutrient applications are not likely to lead to pre-HLB production levels in the short term. Despite some essential nutrients being low in the leaves, the nontreated control trees continued to increase in canopy volume and yield during the course of the study. These responses have promoted development and use of enhanced foliar nutritional programs in Florida. Efficacy of these programs has been a topic of considerable discussion and debate. Fertilization programs vary considerably among growers and consist of various rates and application schedules of essential macro- and micronutrients.
Production managers should consider foliar fertilization to complement soil-applied fertilization to ensure nutrient availability. Field research has shown that supplemental foliar feeding can increase yield by 10%–25% compared with conventional soil fertilization.
Reference
*Morgan, K. T., R. E. Rouse, and R. C. Ebel. 2016. "Foliar Applications of Essential Nutrients on Growth and Yield of 'Valencia' Sweet Orange Infected with Huanglongbing." HortScience 51(12): 1482–1493.
pH Moderation and Root Management—Kelly Morgan, Jim Graham, and Evan Johnson
Typically, citrus trees in Florida groves irrigated with low-volume microsprinklers concentrate fibrous roots in the wetted zone. In recent decades, soils in the irrigated zone under citrus tree canopies have increased in pH and bicarbonate concentrations because of irrigation with alkaline water from deep wells extending into Florida's limestone aquifers. As soils become more alkaline, some nutrients become more available (e.g., N and Mg) for uptake by plants, while others (e.g., Fe, Mn, Zn, and B) become less available. However, declines in tree vigor and productivity caused by HLB alone have been documented in trees growing in soils impacted by alkaline irrigation water.
In a recent greenhouse study, water uptake by trees receiving water supplemented with calcium bicarbonate was significantly reduced (10%–15%) compared with healthy trees and was further reduced by HLB (>20%). Tree heights were similar for HLB-affected and healthy trees irrigated with calcium carbonate but significantly smaller than healthy trees not receiving modified irrigation water. The cause of reduced water and nutrient uptake was found to be reduced root density (examples shown in tables below).
Two field studies determined that Ca, Mg, Mn, Zn, and B concentrations in leaves were greater with irrigation acidification and reduced soil pH than in nontreated controls. Leaf Ca, Mg, Mn, and Zn concentrations were significantly different among treatments in the mature tree grove, but only significantly different for Ca, Mn, and Zn at the young tree grove when averaged over the entire 3-year study period. Root density samples indicated a significantly greater root length density with soil pH below 6.5. These results verify previous finding that leaf nutrient concentrations increase with soil pH below 6.5.
Fertilizer Rates, Application Frequency, and Timing for Nonbearing Trees (First 3 Years in Grove)
Solid Plantings
Nitrogen
Management of young trees requires managing irrigation, nutrition, weeds, diseases, pests, and cold protection at intensities that stimulate rapid canopy growth. Irrigation and N availability are the most important factors affecting growth of young trees. Obtaining optimum growth requires substantial irrigation and N inputs, but excesses of either are nonproductive and costly, and may result in loss of N by leaching or runoff.
Numerous young-tree fertilization studies across Florida led to N recommendations for nonbearing trees (Table 4 and Figure 1). These guidelines include a range of rates by tree age because a number of factors influence the N fertilizer requirement. Criteria for selecting a rate within the recommended range include:
- Soil type—Trees planted in soils high in organic matter (i.e., 2% or greater) or with a loamy texture require less fertilizer than trees on low-organic-matter sandy soils.
- Land history—New plantings on land previously used for pasture or vegetable production require less fertilizer during the first 1 to 2 years compared with trees replanted in established groves due to mineralization of accumulated organic matter.
- Fertilizer source—Use of controlled-release formulations may allow a reduction in fertilizer rate.
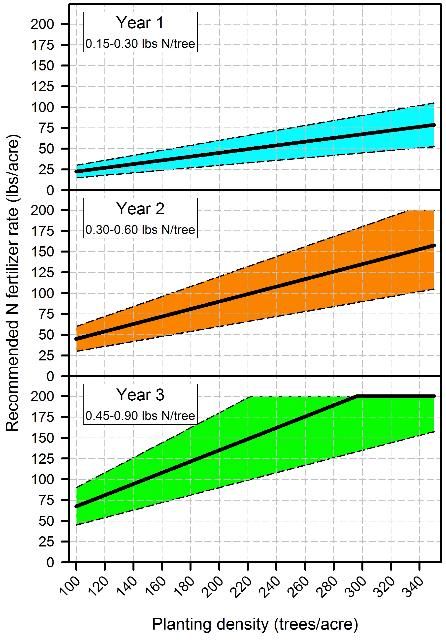
Phosphorus
Before deciding to apply P fertilizer to young trees, test the soil for P and compare the results with the ranges in Chapter 4, Tables 4 or 5.
- If soil test P is in the high or very high range, do not apply P fertilizer.
- If soil test P is in the medium range, apply P fertilizer at a P2O5 rate equal to 50% of the N rate.
- If soil test P is in the low range, apply P fertilizer at a P2O5 rate equal to 75% of the N rate.
- If soil test P is in the very low range, apply P fertilizer at a P2O5 rate equal to the N rate.
If soil testing justifies P fertilizer application, test the soil again the following year and compare with Chapter 4, Tables 4 or 5 to determine if P fertilization can be decreased or omitted. Initiate a leaf tissue testing program for P in year 3, and compare the results with the standards in Chapter 4, Table 2.
Potassium
Apply K fertilizer at a K2O rate equal to the N rate.
Calcium
If the soil pH is in the optimum range of 5.5 to 6.5, there is no need to apply Ca. If soil pH is below 5.5, the soil should be limed to pH 6.5, which will supply needed Ca. If soil pH is above 6.5, the soil will contain abundant Ca.
Magnesium
If soil test Mg is medium or lower (Chapter 4, Tables 4 or 5), apply Mg fertilizer at a rate equal to 20% of the N rate. Curtail Mg fertilizer application if a subsequent soil test shows Mg in the high range.
Micronutrients
If trees are planted on previously cultivated land (e.g., complete grove renovation or land converted from other agricultural uses where fertilizer was applied), do not apply micronutrients unless leaf analysis indicates they are below optimum or leaf/twig/fruit deficiency symptoms appear.
If trees are planted on previously noncultivated land, apply Mn, Cu, and B at 5%, 2.5%, and 0.33% of the N rate, respectively, until soil and leaf analysis and/or tree appearance indicate that one or more may be omitted. Boron may need to be applied every year because it leaches readily. Do not routinely apply Zn, Fe, or Mo unless prompted by visual symptoms.
Nutrient Management
Applying fertilizer in several small doses increases fertilizer efficiency by maintaining more constant N availability and by reducing leaching if unexpected rain occurs. A minimum of 4 to 6 applications of dry fertilizer is recommended. Splitting fertigation into 10 or more applications per year is common. The cost of liquid injection during irrigation is relatively small, particularly if the injection can be automated. One or two applications of controlled-release fertilizer are satisfactory because nutrients are protected from leaching rains. Controlled-release formulations may be applied preplant, incorporated after planting, or broadcast to ensure uniform distribution of nutrients throughout the enlarging root zone of young trees.
Nonbearing trees fertilized after October 1 may be slightly less cold-hardy. However, citrus tree growth is triggered by favorable temperatures and soil moisture, not by fertilization. Omitting fertilizer in the fall will not prevent growth. Fertilizer uptake is reduced at lower soil temperatures. This condition is particularly true for trees on Swingle citrumelo rootstock, which can become quite chlorotic in appearance during the winter months, even with fall fertilization.
Irrigation management of young trees is critical because water stress can occur rapidly as the soil surrounding the limited root system dries, and because young tree growth is particularly sensitive to water stress. Some instances where young tree growth improved after a grove was converted to fertigation may have been due more to improved soil water regime than nutrient delivery method.
Excessive irrigation is often a problem when managing young trees. Small microsprinkler wetted patterns used to irrigate small trees apply water at high rates. Short irrigation durations of 30 minutes or less may be required to avoid nutrient loss below the root zone. Irrigation line flushing times after fertigation must also be minimized to avoid nutrient leaching.
Resets in Established Groves
Resets in established groves should be fertilized similarly to solid-set nonbearing trees. Resets may not grow well if they only receive fertilizer during mature tree application because only a small amount of material may be deposited in the young tree root zone. Resets will most likely not require P fertilizer, but this can be checked with a soil test. Controlled-release materials can be applied 1 to 2 times per year without compromising tree growth in reset situations. In closely spaced groves, reset growth may be restricted due to competition from the adjacent older trees.
Example Fertilizer Program for Nonbearing Trees
Fertilizer rates for trees during the first 3 years in the grove are calculated on a per tree basis. For example, if a 2-year-old tree is scheduled to receive 0.4 lb N per tree per year in four equal applications, then 0.1 lb N will be applied each time. Using a fertilizer containing 10% N, the tree will receive 1.0 lb of fertilizer per application.
Fertilizer Rates for Bearing Trees (4+ Years in Grove)
Nutrient management for bearing trees requires many of the same considerations important for nonbearing trees. Nitrogen continues to be the most important element for tree growth, fruit yield, and fruit quality, but others also have substantial effects on production and fruit quality. Harvesting the crop removes a significant amount of nutrients from the grove, but fruit production accounts for only part of the fertilizer requirement.
Nitrogen
Continued strong vegetative growth is an objective for several years after fruit production begins, so N fertilizer application supports both canopy expansion and fruit production. In addition, fruit quality becomes important for both processed and fresh fruit. Orange and grapefruit groves tend to receive higher N fertilizer rates if the fruit is grown for processing, because returns are based on lb solids/acre (total sugar) production. If the fruit is grown for the fresh market, where fruit size, shape, peel thickness, texture, and color are important, the N fertilizer rate is usually lower, perhaps two-thirds or three-quarters of the processed fruit rate.
Young bearing trees (years 4 through 7 in the grove). Recommended N fertilizer rates (Table 5) provide enough N for canopy expansion toward containment size while producing maximum economic yields of high-quality fruit. The N rate selected should be based on soil characteristics, yield potential, and tree needs as indicated by leaf analysis interpretation (Chapter 4, Table 2).
- For grapefruit, the recommended annual N rate is 120 to 160 lb/acre.
- For oranges and other varieties, the recommended annual N rate is 120 to 200 lb/acre.
Mature bearing trees (years 8+ in the grove). Once trees reach containment size, further canopy growth is not desired, so nutrition inputs can be stabilized and possibly reduced. Nitrogen fertilizer management should focus on 1) maintaining tree biomass, 2) generating sufficient vegetative growth to replenish fruiting wood, and 3) replacing N exported with the harvested crop. The guidelines for annual N fertilizer rates accounts for the needs of both vegetative growth and crop removal (Table 5).
Grapefruit
- The recommended annual N rate is 120 to 160 lb/acre. For groves producing more than 800 boxes/acre, 180 lb/acre may be considered. The N rate selected should be based on tree needs as indicated by leaf analysis interpretation, soil characteristics, desired fresh-fruit quality characteristics, and yield potential.
- The optimum leaf N concentration associated with best grapefruit quality is around 2.2% (Figure 2), which is lower than the optimum leaf N range for orange production (2.5% to 2.7%). To achieve high yields of large fruit, growers should adjust N fertilizer rates to maintain grapefruit leaf N around 2.2%.
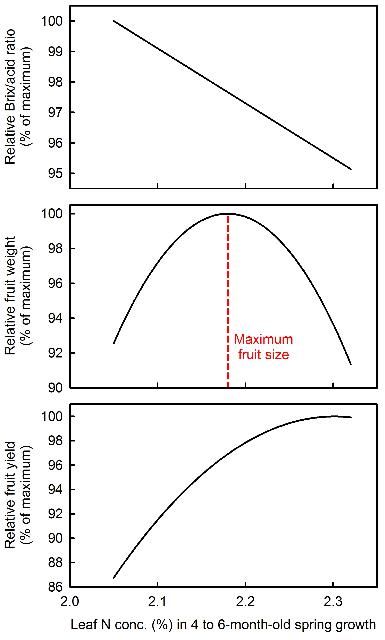
Oranges
-
The annual N rate should fall within the range of 125 to 245 lb/acre. The recommended rate for a specific grove depends on either expected yield potential (for 8-to-11-year-old trees) or 4-year running average production history (for trees 12 years or older) expressed as either fruit yield or soluble solids production (Figure 3).
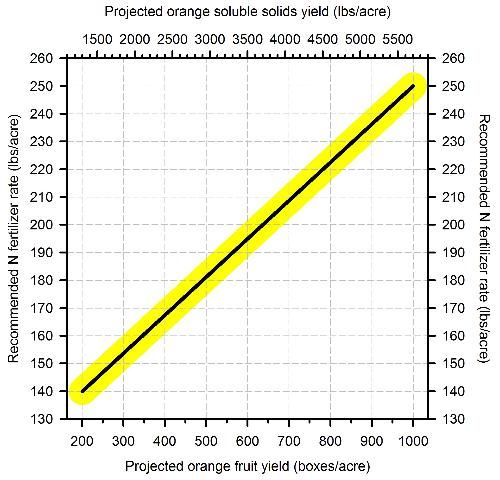
- When basing N fertilization on expected yield potential, the rate should be selected considering 1) how well the young bearing trees have produced and 2) leaf tissue analysis.
- If leaf N is consistently maintained in the optimum range, additional fertilizer will not increase yield (Figure 3) and may reduce some aspects of fruit quality.
- The base N rate recommendation (125 lb/acre) is for groves producing 200 boxes/acre or 1300 lb solids/acre.
- The high end of the N rate range (245 lb/acre) is for groves producing 1000 boxes/acre or 5800 lb solids/acre.
- Beginning at the base N rate, the recommended N rate increases:
- lb N/acre for every 100 box/acre increase in expected yield potential or 4-year running average yield; or
- 7 lb N/acre for every 100 lb solids/acre increase in expected yield potential or 4-year running average yield.
Other Varieties
- For other varieties, the recommended annual N rate is 125 to 245 lb/acre using the method above to calculate a production-based rate for specific groves.
- For the special case of Orlando tangelos, the high end of the annual recommended N rate range is 250 lb N/acre.
- For the special case of Honey tangerines (Murcotts), the high end of the annual recommended N rate range is 300 lb N/acre.
Example N fertilizer rate calculation for mature, bearing orange trees using box/acre yield:
Recommended N rate = Base N rate +
{[(Avg. yield – 200 boxes/acre)/100] × 15 lb/acre}
If a grove has produced an average of 500 boxes/acre during the past 4 years, then
Recommended N rate = 125 lb N/acre +
{[(500 – 200)/100] × 15 lb N/acre}
= 125 + 45 = 170 lb N/acre
Example N fertilizer rate calculation for mature, bearing orange trees using lb solids/acre yield:
Recommended N rate = Base N rate +
{[(Avg. yield – 1300 lb sol./acre)/100] × 2.7 lb/acre}
If a grove has produced an average of 3500 lb solids/acre during the past 4 years, then
Recommended N rate = 125 lb N/acre +
{[(3500 – 1300)/100] × 2.7 lb N/acre}
= 125 + 59 = 184 lb N/acre
Leaching rain rule. If more than 3 inches of rain falls within 72 hours after an N fertilization, "replacement" fertilizer may be applied up to one-half of the N rate affected by the rain (not to exceed 30 lb/acre). If the affected N fertilizer source was 100% slow-release or controlled-release, this rule does not apply. If the source was a mixture of water-soluble and slow- or controlled-release N, this rule applies only to the soluble N fraction.
Phosphorus
Determine the need for P fertilization using leaf tissue and soil test results.
- Sample leaves and soil using the procedures described in Chapter 4.
- Compare the analytical results with the interpretations provided in Chapter 4, Tables 2, 4, and 5.
- Follow the P fertilization guidelines in Table 6.
Potassium
Apply K fertilizer at a K2O rate equal to the N rate. If leaf K is consistently below optimum from year to year, increase the K2O rate by 25%, especially if the grove soil is calcareous.
Calcium
If the soil pH is in the optimum range of 5.5 to 6.5, there is no need to apply Ca. If soil pH is below 5.5, the soil should be limed to pH 6.5, which will supply needed Ca. If soil pH is above 6.5, the soil will likely contain abundant Ca.
Magnesium
If soil test Mg is medium or lower (Chapter 4, Tables 4 and 5) or if leaf Mg is below optimum (Chapter 4, Table 2), apply Mg fertilizer at a rate equal to 20% of the N rate. Alternatively, Mg may be applied in a foliar spray. Curtail Mg fertilizer application if a subsequent soil test shows Mg in the high range, or if leaf Mg improves to optimum or greater.
Micronutrients
The quantities of micronutrients in 100 boxes of fruit are extremely small (Chapter 3, Table 3). Removal of micronutrients by the harvested fruit, even from a high-producing grove, is negligible compared with the amount present in the soil. In high pH (>7) soils, micronutrient availability (except Mo) decreases considerably. Micronutrients (with the exception of B and Cu) should be applied as needed based on visual, persistent foliar deficiency symptoms or low leaf-analysis values. Recommended methods, timing, and rates for micronutrient application to citrus groves are shown in Table 7.
Factors influencing the effectiveness of foliar sprays include the formulation used, metallic rate per acre, and timing of the spray with respect to leaf age. Many commercially available micronutrient formulations, when applied at their recommended label rates, will maintain sufficient leaf concentrations but usually will not correct moderate to severe deficiencies. Water-soluble micronutrient fertilizers may be included with postbloom or summer sprays after full expansion of leaves.
Copper. Some central Ridge and Atlantic Coast grove soils contain 300 to 400 lb Cu/acre in the top 6 inches of soil. A moderate routine disease-control spray program contributes an additional 3 to 4 lb Cu/acre/year, so no soil application of Cu fertilizer is needed in this situation. A soil pH below 5.5 can solubilize soil Cu, which is toxic to plants. As little as 1 ppm Cu in the soil solution can kill roots. Maintaining soil pH close to neutral is recommended to reduce the potential for Cu toxicity. Some rootstocks (e.g., Swingle citrumelo) that perform poorly in high-pH soils are also sensitive to high soil Cu. When replanting on old grove sites with low soil pH, the potential for toxicity is high.
While the emphasis with old grove soils is on managing high soil Cu, most previously noncultivated Florida sandy soils are deficient in Cu. If a grove is developed on a virgin sandy soil, Cu fertilizer should be included in the fertilizer blend (see section "Solid Plantings").
Iron. Soil applications of non-chelated, inorganic Fe fertilizers usually cannot correct visible Fe deficiency symptoms. Because these Fe fertilizers readily precipitate, they are unavailable to the tree. In neutral to slightly acidic soils, Fe deficiency can also be a problem if Cu is present at high concentration.
Calcareous soils may contain high total Fe, but it is extremely insoluble. Visible Fe deficiency is common on these soils. The primary factor causing Fe chlorosis in an alkaline soil is the effect of the bicarbonate ion (HCO3-) on Fe uptake and/or translocation in the plant. The result is an inactivation or immobilization of Fe in plant tissue. Citrus rootstocks vary widely in their ability to overcome low Fe stress (Table 8). The easiest way to avoid alkaline-induced Fe chlorosis in citrus trees to be planted on calcareous soils is to use tolerant rootstocks.
Iron chlorosis should be corrected by soil application of chelated Fe fertilizer. Chelates are superior sources of Fe for plants because sufficient Fe can be supplied at lower rates compared with inorganic Fe sources. The effectiveness of an Fe chelate depends greatly on soil pH (Table 9). Fe-EDTA and Fe-HEDTA, which are relatively inexpensive, will correct Fe deficiency if soil pH is less than 6.5. Do not apply these chelates to alkaline soil, because they will readily break down, resulting in loss of available Fe by precipitation.
Iron chlorosis of citrus trees on susceptible rootstocks growing on calcareous soil is not easily remedied. Effective Fe chelates for these soils are available, but the treatments can be expensive and leaf greening is usually transient. Fe-DTPA should be chosen for mildly alkaline soils (pH 7.5 or less), whereas Fe-EDDHA is the chelate of choice for highly calcareous soils (pH greater than 7.5).
Organically complexed Fe exists in by-products like wastewater residuals (biosolids) or certain drinking-water treatment residuals (Fe-humates). Biosolids are potentially useful because they contain a high concentration of Fe that exists in a complexed form that does not readily precipitate. Research with Fe-humate applied to citrus trees showed that moderate to severe Fe deficiency could be corrected at relatively low cost.
Zinc. Soil pH is the most important factor regulating plant-available Zn. At alkaline pH, Zn precipitates and availability markedly decreases, so soil pH less than 7 is the preferred situation. Although there are natural mechanisms in the soil-plant system that increase the availability of Zn in alkaline soils, Zn deficiencies are common.
Special consideration should be given to groves being visually monitored for HLB symptoms (see section 3.9). Trees on Carrizo citrange rootstock tend to show Zn deficiency symptoms more readily, even though the tree is not likely Zn deficient.
Application of foliar Zn fertilizer is usually combined with pesticide sprays scheduled in April or May at 3 to 5 lb of metallic Zn/acre using either ZnO or ZnSO4. A number of other formulations are available for foliar application, including nitrates and organically chelated forms using lignin sulfonate, glucoheptonate, or alpha-keto acids. Practically speaking, inorganic and organic Zn fertilizer sources are about equally effective with respect to foliar absorption.
Application of Zn directly to acidic soils is not economically practical due to the massive rates required to correct a deficiency. Zinc should not be ground-applied to groves on calcareous soils because the alkaline pH renders the Zn unavailable almost immediately.
Manganese. The behavior of Mn in the soil is similar to that of Zn, especially with respect to relative availability in acidic and alkaline soils. Either sulfate, oxysulfate, or some oxide forms of Mn can be used to correct Mn deficiency, with the degree of effectiveness decreasing in that order. Soil application of Mn is not recommended on calcareous soils where Mn deficiency is commonly encountered.
For groves on acidic soils that show persistent Mn deficiency symptoms on young foliage, soil application of 7 to 10 lb of Mn as MnSO4 per acre is recommended. On calcareous or heavily limed acid soils, foliar application of 3 to 5 lb of Mn per acre is recommended. A special effort to prevent Mn deficiency symptoms should be made in groves being visually monitored for citrus greening disease symptoms (see section 3.9).
Boron. Boron is required in very small amounts, and there is only a small range between deficient and toxic amounts. It should be applied annually as a foliar spray or in a dry fertilizer mixture at approximately 1/200 of the N fertilizer rate. Irrigating citrus with reclaimed water may provide sufficient B such that supplemental fertilization is not required.
Molybdenum. Molybdenum is also required in very small amounts. If Mo deficiency occurs, it usually means that the soil is very acidic. The deficiency is corrected by a foliar spray, which may last for several years. Soil applications are not satisfactory.
Timing and Frequency of Fertilizer Application for Bearing Trees
Bearing-tree nutrition management must support both vegetative growth and the current fruit crop. Spring vegetative growth is particularly important because it forms the fruiting wood for the following year's crop. The period of highest nutrient requirement begins in late winter and extends through early summer. During this time, flowering and fruit development competes with spring vegetative growth flushes. Flowers and fruitlets take up accumulated nutrients, but some of these are temporarily lost during the flower-fruitlet shedding process. The tree is then left with the fruit it can sufficiently support to maturity. This process continues until the May–June drop of fruitlets is completed. Nutritional requirements for fruit development decrease after this period. Best fruit quality is obtained when fall and early winter nutritional status, particularly N, is moderately low.
Based on the nutritional demands during a typical year, a basic fertilizer application schedule divides the total annual requirement into three equal increments:
- The first increment should be made available between early February and the time flowering occurs.
- The second increment should be made available between flowering and mid-June.
- The third increment should be made available between mid-June and mid-October, avoiding the summer rainy season as much as possible. Thus, this increment should be applied during late summer or early fall.
Fertilizer may be applied during the fall and winter, particularly in the southern portion of the citrus-growing region, where trees often grow throughout the year. Any time growth is induced by warm weather, sufficient nutrients should be available. However, be aware that fall or winter fertilizer applications can make psyllid control more difficult (see section 3.9), delay fruit color development, and increase the susceptibility of trees to freeze injury.
Much of Florida's citrus industry exists on shallow Flatwoods soils with intensive irrigation, so the danger of root damage from high salinity is greater than it is on deep sandy soils. Split fertilizer applications minimize salt damage potential, decrease leaching during the summer rainy season, and help maintain a continuous nutrient supply during south Florida's long growing season.
Slow-Release and Controlled-Release Fertilizers
Slow-Release N Sources
Citrus growers can reduce the number of fertilizer applications per year when slow-release N sources like sulfur-coated urea, urea formaldehyde, methylene urea, or IBDU make up 40% to 60% of the total N in the fertilizer. For example, a grower applying 100% water-soluble N fertilizer (e.g., ammonium nitrate) four times per year could cut the application frequency to twice per year if the N source was changed to a 50-50 blend of ammonium nitrate and IBDU. However, the total N rate applied per year should be the same in both cases.
If a natural organic material like manure or biosolids is included as part of the N applied to citrus, the mineralization rate must be considered when determining the rate to apply. Organic matter decomposes relatively quickly in Florida's warm and humid environment, so N mineralizes much faster than it would in a cooler northern climate. Roughly half of the N in biosolids and two-thirds of the N in poultry (layer) manure becomes plant-available during the first year after application. For example, if a poultry manure application supplied 100 lb/acre of total N to a grove, about 66 lb N/acre would become available to the trees during the next 12 months.
Research conducted in several Florida locations showed that N mineralization is front-loaded in the case of both poultry manure and biosolids (Table 10). Most of the N becomes plant-available in the first month after application, followed by a gradual release of the remainder during the subsequent 11 months. Research has shown that the amount of plant-available N released from either material after 12 months is negligible. The N not accounted for by the plant-available fraction most likely volatilizes, denitrifies, is used by soil microbes, or remains in the soil as recalcitrant organic matter.
Controlled-Release Fertilizers
Commercial fertilizer companies have blended together individual coated fertilizer materials, each with a different release rate, to create controlled-release mixtures suitable for single annual applications to citrus. For example, a fertilizer made to match the nutrient requirements of citrus trees might be composed of a suite of water-soluble 3-month, 6-month, and 9-month materials. A blend like this would be applied once, in February. Ideally, it would release two-thirds of its nutrients from February to June, and the remaining one-third would gradually release between June and October.
Coated fertilizers provide the highest nutrient uptake-efficiency potential of any fertilizer class. Recent research suggests they can sustain equal or increased fruit production when applied at lower N rates compared with a standard water-soluble N fertilization program. Because they are considerably more expensive than water-soluble materials, applying coated fertilizers at lower rates may be necessary to keep a fertilizer program economically competitive. Because the marketing of coated fertilizers for mature citrus groves is relatively new, growers are encouraged to consult the fertilizer manufacturer or blender for the latest rate recommendations.
Foliar Application of N, P, and K
The amount of plant nutrients that can be taken up through the leaves of a citrus tree is miniscule compared with the amount that can enter through the roots. Micronutrients can be successfully applied with foliar sprays because the tree does not require them in large amounts (Chapter 3, Table 1), but leaves are not usually thought of as a major uptake site for macronutrients. However, there are special instances where foliar application of N, P, and/or K is justified. Citrus growers should realize that in the cases of N and P, a positive response to foliar sprays may be due to additional effects of the materials on tree physiology beyond simple enhancement of tree nutrition.
Nitrogen
Commercial forms of urea are available that can be readily absorbed by citrus leaves, particularly if applied with a non-ionic surfactant. Foliar urea sprays applied during the winter have enhanced the number of flowers and yield of Valencia oranges in both research plots and commercial trials. These sprays presumably work only if some induction has taken place from natural cold or drought stress. Therefore, for effective use in the winter, urea sprays should be applied after some natural flower induction has occurred but before most bud differentiation starts. In Florida, significant induction may not start until late December some years, while in other years some buds may be differentiating by early January. After some cool temperature induction or 30 days of drought stress, foliar sprays of 50–60 lb of spray-grade urea per acre can enhance flower bud induction and may increase fruit yield. Care must be taken to apply the correct rate, because leaf damage can occur if urea application is excessive.
Maximum penetration of urea into citrus leaves occurs within 12 to 24 hours after spray application. Optimum conditions for foliar uptake include:
- Air temperature between 77°F and 88°F
- High relative humidity
- Spray solution with a pH between 7 and 8 to prevent urea breakdown
Under favorable environmental conditions, roughly half of foliar-applied urea penetrates the leaves, while most of the other half is lost through volatilization. The rate of foliar-applied N should be considered as part of the total annual N rate applied to the grove. For example, a foliar spray of 50 lb urea/acre applies 23 lb N/acre. If the fertilization plan calls for a total of 180 lb N/acre/year, only 157 lb N/acre should be included in the soil-applied fertilizer program.
In Florida citrus production areas where groundwater nitrate contamination exists or is seen as a potential problem, urea sprays should be evaluated to provide a portion of the tree N requirements, especially during the summer months when the leaching potential is greatest.
Phosphorus
Citrus leaves are extremely impervious to the phosphate (PO43-) form of P, so foliar application of a liquid P material like ammonium polyphosphate is not recommended. Conversely, the phosphite (PO33-) form of P is more readily absorbed into plant tissue, and once inside the plant it remains stable. Phosphite does not readily convert to phosphate in the plant, so the nutritional value of absorbed PO33- is uncertain. However, phosphite is officially recognized by FDACS as a source of P for crops.
In California, research showed that foliar applications of phosphite were able to replace standard P fertilization in citrus crops suffering from P deficiency. The conversion of phosphite to phosphate likely occurred prior to plant absorption, resulting from slow chemical oxidation or by oxidizing bacteria and fungi found living on citrus leaves. Phosphite also showed fungicidal activity and increased citrus floral intensity, yield, fruit size, total soluble solids, and anthocyanin concentration, usually in response to a single foliar application.
In Florida, a prebloom foliar application of 2.6 quarts of 28% P2O5 as potassium phosphite per acre to Valencia oranges significantly increased flower number, fruit yield, and total soluble solids yield compared with an untreated control. These results suggest that the effect of phosphite was not due to the molecule's fungicidal attributes, but due to other growth-stimulating properties.
Citrus growers should identify their production goal for the year (e.g., increased yield, increased fruit size, or improved fruit quality) to determine if a phosphite application is justified. Be aware that phosphite materials, if not formulated correctly, have significant phytotoxicity potential and may induce adverse reactions with other materials in the spray tank, like micronutrients or pesticides.
Potassium
Many factors contribute to the size of fruit in a particular year, such as fruit load, rainfall pattern, fertilization program, hedging and topping, and rootstock/scion combination. However, it is difficult to predict how these factors combine to affect final fruit size at harvest. The easiest factor to manipulate is nutrient management. Among other fruit qualities, increased K fertilization is associated with larger size (Chapter 3, Table 4).
Effects of low K on fruit yield and quality generally precede appearance of leaf deficiency symptoms. Decreased yield and small fruit have been observed on trees with leaf K in the range of 0.5% to 0.8%, while K concentrations of 1.2% or more have been associated with maximum yield of high-quality fruit.
Applying foliar sprays of K cannot entirely substitute for soil-applied fertilizer, but they can serve as a supplement, and their ability to increase fruit size has been demonstrated. Foliar-applied K has also corrected K deficiency of citrus on calcareous soil. Applying potassium nitrate (KNO3) in this manner increases leaf K more rapidly compared with soil-applied fertilizers because plant uptake is much faster, but the positive effect is shorter-lasting.
Salt index. The salt index of a fertilizer (discussed in Chapter 11, section "Saline Soils and Water") measures its tendency to increase the osmotic pressure of the soil solution compared with an equal amount of sodium nitrate. High soluble salt concentrations in the soil may develop an osmotic pressure exceeding that of the plant sap, possibly resulting in dehydration and permanent injury. When salt solutions are sprayed on leaf surfaces, similar results may occur. Typically, the higher the salt index, the greater the potential to burn leaves or fruit.
Materials. Potassium sources used for foliar K application include KNO3 (13-0-44), monopotassium phosphate (MKP, 0-52-34), and dipotassium phosphate (DKP, 0-18-20). DKP is made by combining MKP and potassium hydroxide. When applying MKP or DKP, a small amount (3% to 5%) of low-biuret urea should be included to enhance uptake. KNO3 is usually applied without surfactants or urea.
Application rates and timing. Research in the Indian River production area showed that about 8 lb of K2O per acre were needed per foliar application to achieve satisfactory results. Higher rates did not show additional benefit, and lower rates resulted in less fruit enlargement. Beyond spring applications, successive sprays through the summer did not improve performance.
If foliar spraying with 100 or more gal of water/acre, any K source is acceptable, so the least expensive should be chosen. When applying foliar K with low-volume equipment, MKP or DKP should be used to minimize the burn potential. MKP has been applied to grapefruit at rates as high as 106 lb MKP in 125 gal water per acre under hot, dry conditions with no adverse effects. The low salt index of MKP (only 1/6 that of KNO3 per unit of K2O; see Appendix C, Table 1) makes it very "safe" to use.
Caution: Be careful if tank-mixing MKP with other materials. The MKP-water solution has a pH of about 4.5, which may not be suitable for some tank-mix combinations. DKP makes a pH-neutral solution that would be a better choice if tank-mixing with other materials.
Timing is important for K applications to enhance fruit size. Potassium is a primary component of cell walls, accounting for more than 40% of fruit mineral content. About 70% of final fruit size is related to the number of cells in the fruit, so more cells usually means larger fruit. Cell division typically stops by late April, and size change throughout the rest of the year comes from cell enlargement. Therefore, the maximum effect of foliar K is achieved from applications that make it available during bloom and postbloom when it can be used during both cell division and rapid cell enlargement phases. An additional application with the summer spray (normally in July) is also recommended to ensure sufficient K through the summer growing season.
Grapefruit size enhancement occurred in about half the fall field trials in the Indian River area, suggesting that late summer or fall K applications may be effective some years. Fall applications were most effective in years with wet summers and falls. Shorter day lengths and cooler weather results in a dramatically decreased fruit expansion rate after mid-October in most years. Thus, if foliar K applications for fruit enlargement are considered during late summer or fall, they should be made in August or September to be most effective.
Expected results. Studies on Sunburst and Valencia showed that foliar-applied K produced 25% to 33% more larger-sized fruit compared with nontreated plots. In addition, there was a corresponding increase in soluble solids yield in the Valencia experiments. Combining prebloom, postbloom, and summer K sprays can increase average fruit diameter 0.16 to 0.24 inches, which can equal 1 or more pack sizes. When fall application was successful, grapefruit diameter increased 0.08 to 0.16 inches, or about ½ to 1 size category. Foliar K will not produce large fruit from small fruit, but it can move a significant portion of the fruit into a larger size class. The following observations and recommendations are based on the Indian River–area experiments:
- The recommended program for most citrus varieties is 8 lb K2O/acre per application, applied prebloom (typically February), postbloom (typically April), and summer (July).
- If the summer and fall are wetter than usual, later K applications may be considered. When exercising this option, schedule the applications for August and September.
- Foliar K application has had little or no effect on juice volume, acid, Brix, or Brix:acid ratio.
- Diameters of smaller fruit tended to increase more than larger fruit when foliar K applications were made.
- Fruit burn was not observed at the following spray concentrations:
- lb KNO3/acre applied in 125 gal of water/acre.
- lb MKP/acre applied in 32.5 gal of water/acre.
- lb MKP/acre applied in 10 gal of water/acre by airplane.
Calcium, Magnesium, and Micronutrients
Foliar applications of Ca, Mg, and micronutrients (Zn, Mn, Cu, B, and Mo) have proven to be an excellent means of satisfying citrus tree requirements. However, there can be difficulties associated with leaf tissue absorption and translocation of Ca, Mg, B, and Mo. Choosing the correct fertilizer sources for these nutrients can be critical. Foliar application of nutrients is of great importance when the root system is unable to keep up with crop demand or when the soil has a history of problems that inhibit normal nutrient uptake. Foliar nutrition is proven to be useful under prolonged periods of wet conditions, droughty conditions, calcareous soil, cold weather or any other condition that decreases the tree's ability to take up nutrients when there is a demand. Foliar feeding may be effectively utilized when a nutritional deficiency is diagnosed. Foliar application is absolutely the quickest method of getting nutrients into plants. However, if the deficiency can be observed on the tree, the crop has already lost some potential yield.
Application of Zn sprays on the spring flush is recommended, but it may be necessary on each major flush of growth to keep the trees free of deficiency symptoms, because Zn does not translocate readily to successive growth flushes. Maximum benefit is obtained if spray is applied to the young growth when it is two-thirds to nearly fully expanded and before it hardens off. Soil application of Zn in the fertilizer is neither an economical nor an effective way to correct Zn deficiency.
Foliar spray application of Mn quickly clears up deficiency symptoms on young leaves, but older leaves respond less rapidly and less completely. When Mn sprays are given to Mn-deficient orange trees, fruit yield, total soluble solids in the juice, and pounds solids per box of fruit increase. Foliar spray of a solution containing Mn on two-thirds to fully expanded spring or summer flush leaves is recommended. Adding 7 to 10 lb of low-biuret urea will increase Mn uptake.
In Florida, foliar spray applications of B have been found much safer and more efficient than soil application. Soil applications frequently fail to give satisfactory results during dry falls and springs and may result in toxicity problems if made during the summer rainy season. Foliar spray may be applied during the dormant period through postbloom, but preferably during early flower development. Treating at this growth stage is important because boron does not move very readily from other parts of the tree to the buds. Applying B at this time will assist in flower initiation and pollen production, satisfy the needs for pollen tube growth, and enhance fruit set. Use care not to apply more than the recommended amount, because it is easy to go from deficiency to toxicity.
Recommendations for P fertilization of bearing citrus trees based on leaf tissue and soil tests taken according to the guidelines described in Chapter 4 (leaf and soil samples taken in July or August of each year).1