Florida's Geological History
Introduction
While modern-day Florida lacks snowcapped mountains, deep river-cut canyons, exploding volcanoes, glaciers, large outcrops of sedimentary layers, or frequent earthquakes, it is nevertheless a fascinating place to study geology. Having strong ties to marine and coastal processes, Florida's geologic story is unique. The term "Florida geology" should invoke visions of azure shallow seas, bustling coral reefs, hollow caverns beneath your feet, Ice Age animals traversing the ancient landscape, massive amounts of freshwater stored deep in our aquifers, and even sinkholes large enough to consume a building. As we tread upon the ever-changing Floridian landscape, it is important to understand how Florida (as we know it) has formed over the last several hundred million years.
What is Florida?
Geologically, Florida perches on top of what geologists call the "Florida Platform," a plateau that is mostly underwater. Due to sea-level fluctuations through geologic time, the Florida Platform has both been entirely underwater (during interglacial periods) and entirely above sea-level (during glacial periods). Today, only about one-third of the Florida Platform is above sea-level (Figure 1, top). The current shape of Florida's coastline is merely a snapshot in geologic time because sea-level is constantly fluctuating with our planet's climate cycles.
In the Gulf of Mexico, the Platform extends over 100 miles to the west of the modern shoreline. While mountainous terrain does not exist on the modern-day Florida peninsula, there is an extraordinarily steep topographic (or bathymetric) landscape on the margins of the Florida Platform. At its westernmost edge, a steep slope descends over 9000 feet from the relatively shallow West Florida Shelf, down to the abyssal plain of the Gulf of Mexico (Figure 1, bottom). This feature is called the "West Florida Escarpment." From the base of the West Florida Escarpment (the abyssal plain) to the highest point in Florida (Britton Hill), the Florida Platform has a topographic relief of approximately 10,000 feet. This relief is higher than the majority of the US states, but because this varied topography is all under water and not considered part of the state, the Sunshine State holds the record for the flattest state in the United States.
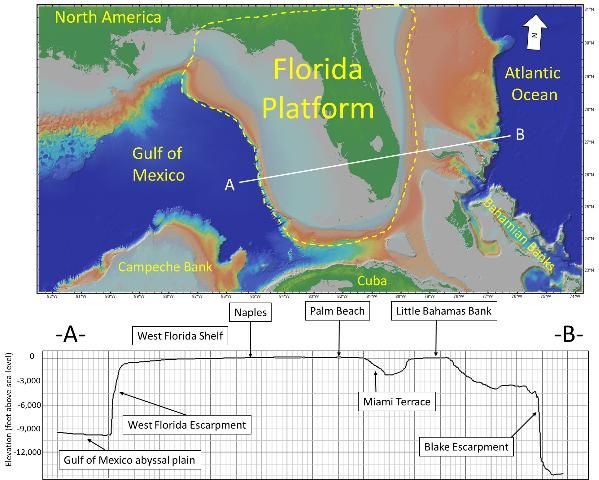
Credit: Map and elevation-depth profile of the transect was generated from GeoMapApp software provided by Ryan et al. 2009.
How did Florida form?
The hundreds of millions of years of geologic processes that lead to the formation of Florida can be divided into three phases. Each of these phases brought new materials and made the Florida Platform what it is today.
Phase 1: The Age of Plate Tectonics (More Than 160 Million Years Ago)
"Plate Tectonics" describes the large-scale motion (collision and divergence) of continental and oceanic lithosphere (the cool, rigid, outer layer of the earth) over the Earth's hot, ductile interior mantle; Florida's earliest origins are tied to this process. The two crustal fragments that underlie Florida (i.e., basement rock) have non-North American origins. They were formed on the western coast of the ancient supercontinent Gondwana (composed of Antarctica, South America, Africa, Australia, Madagascar, Arabia, and India). Florida's volcanic and sedimentary rocks (found 0.6 to 3 miles below the surface) have similar compositions and ages to rocks of Senegal, Africa, and are markedly different from those from the rest of SE North America (Dallmeyer et al. 1987; Lane 1994). This is evidence of Florida's tectonic placement before the formation of Pangea (Dallmeyer et al. 1987; Hine 2013).
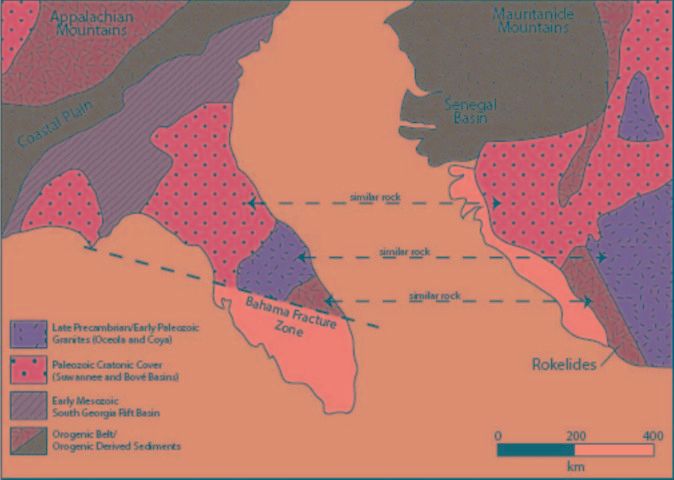
Credit: Hine 2013
Approximately 300 million years (a million years is a "mega-annum," abbreviated Ma) ago, Gondwana collided with the supercontinent Laurasia (composed of North America, Europe, and Asia) forming an even larger supercontinent called Pangea. During all of this, Florida was sandwiched in between the Americas and Africa.
Supercontinents are tectonically unstable over geologic time; one theory is that the huge continents trap underlying heat, which leads to thermal uplift, volcanic rifting, and splitting of the supercontinent. When Pangea began rifting (getting pulled apart), the Americas started migrating westward, and the crustal fragments that underlie Florida (Suwannee Basin Block and Florida-Bahama Block) were so well-fused onto the North American continent that they remained attached to south Georgia, leaving Africa behind. At that time, climate was very warm and sea level was very high; the primitive Florida crustal fragments were flooded, setting the scene for the next age of the Florida Platform's construction, the age of carbonate production.
Phase 2: The Age of Carbonate Production (160–23 Million Years Ago)
What are carbonates? Carbonates are a diverse suite of geologic materials that are composed of mainly calcium carbonate (CaCO3). Many carbonate rocks, such as limestone, are derived from skeletal material from marine organisms like corals, plankton, algae, gastropods, and sea urchins. Carbonate deposits are special geologic features because they typically only form in environments with warm, shallow, clear water of marine salinity. Additionally, organisms that produce carbonate materials cannot thrive with the abundance of siliciclastic sediment (i.e., sediment eroded from igneous rocks, such as granite) or in areas with high nutrient levels. We can observe modern carbonate production occurring in the relatively pristine waters of Bahamas, the Yucatan Peninsula, and the Western Florida Shelf.
Due to a warmer global climate and subsequently high sea level that occurred during the Middle to Late Mesozoic (190–66 Ma ago), Florida's igneous basement was flooded. Sea level was high enough that a strong current flowed between Florida and Georgia, called the "Georgia Seaway" or "Suwannee Channel" (Gardulski et al. 1991). This current prevented continental siliciclastic sediments (produced from the erosion of the Appalachian Mountains) from moving southward, keeping the Florida Platform and shallow tropical seas free of clay, quartz sand, and silt (Figure 3).
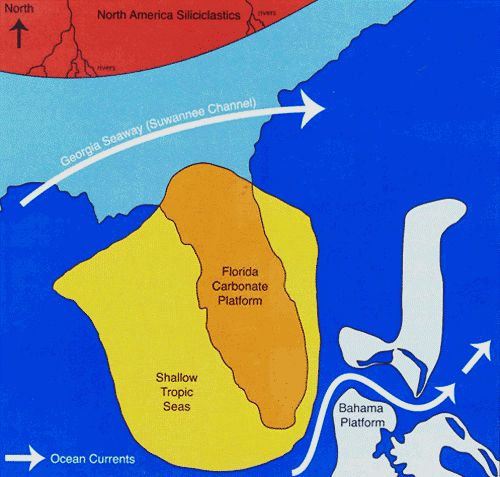
Credit: Hine 2013
As the North American continent continued migrating northwest across the tropical latitudes, the conditions for forming carbonate sediments and rocks (e.g., limestone) on top of the Florida Basement were ideal (e.g., warm, shallow, marine). At that time, all of Florida likely resembled the submerged portions of the Bahamas (Gardulski et al. 1991). The massive deposition of limestone occurred for approximately 130 million years across the Florida Platform. These deposits can be found at the surface throughout much of the state of Florida; the age of the limestone varies from modern (e.g., in the Florida Keys and along the Atlantic coastline) to ~35 million years old (e.g., west of Cedar Key); however older carbonate rock from the Mesozoic is present deep below younger exposed carbonates. Figure 4 describes how you could identify carbonate rocks, such as limestone, in the field.
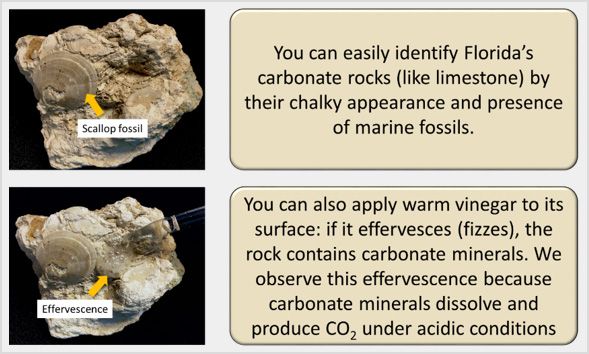
Credit: K. Bostick
Natural acids dissolve limestone over millions of years to form caves, sinkholes, and complex underground drainage systems (Palmer 1991; Screaton et al. 2004; White 2002; Worthington 1999). This property gives limestone great porosity and permeability and creates large aquifers throughout the state (Martin and Dean 2001). An aquifer is an underground geologic formation that stores and transmits usable quantities of water. The Floridan Aquifer underlies the entirety of Florida and parts of Alabama, Georgia, Mississippi, and South Carolina (Figure 5). It is one of the world's most productive aquifers and supplies drinking water for nearly 10 million people, as well as aiding the thriving agriculture industry in Florida. The limestone unit that hosts much of the Floridan Aquifer is named the "Ocala Limestone Formation," a 33.9- to 55.6-Ma-old limestone formation (Martin and Dean 2001; Martin and Screaton 2001).
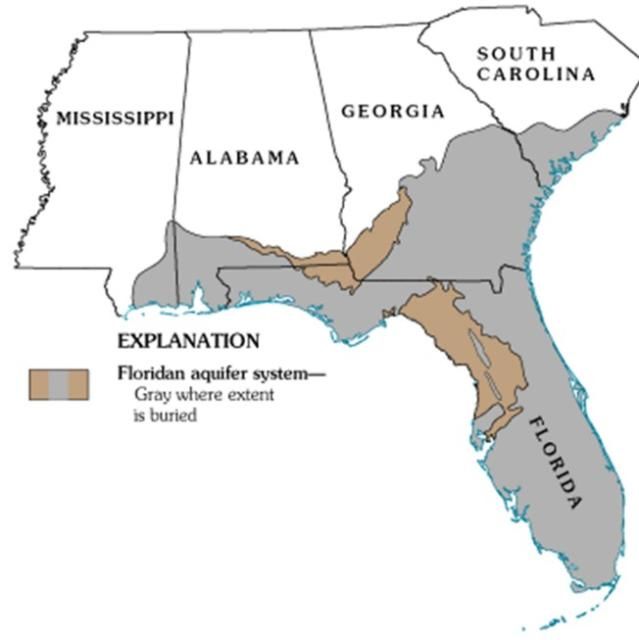
Credit: USGS 2017
Phase 3: The Age of Siliciclastic Invasion (23 Million Years Ago–Present)
As North America tectonically began moving northwest into its current position, other continents were also drifting to their current positions. When South America fully detached from Antarctica (~30 Ma), the climate cooled significantly as the Antarctic Circumpolar Current formed and promoted the formation of large ice sheets. With the formation of ice sheets, sea level began to fall significantly. As the Georgia Seaway became more shallow, its ability to deflect siliciclastic sediments from entering Florida Platform was diminished (Missimer and Maliva 2017). Around 23 million years ago, the massive deposition of siliciclastic sediment began in Florida. As a result, carbonate production reduced substantially in north and central Florida, while relatively siliciclastic-free south Florida continued to produce carbonate materials.
North Florida began to form its characteristic peninsula shape when siliciclastic sediment began migrating southward. One of the most well-known siliciclastic-rich deposits in Florida is the Hawthorn Group (Bryant et al. 1992). The Hawthorn Group is an entire suite of geological formations that are mainly composed of sand, silt, and clay brought southward into Florida via rivers and coastal currents. The Hawthorn Group is rich in fossils such as saber-toothed cats, camels, tapirs, rhinoceroses, giant ground sloths, elephants, horses, dugongs, whales, and sharks (usually just their teeth). These animals roamed the primitive Florida Peninsula or swam across it during periods of high sea level (Webb et al. 1981).
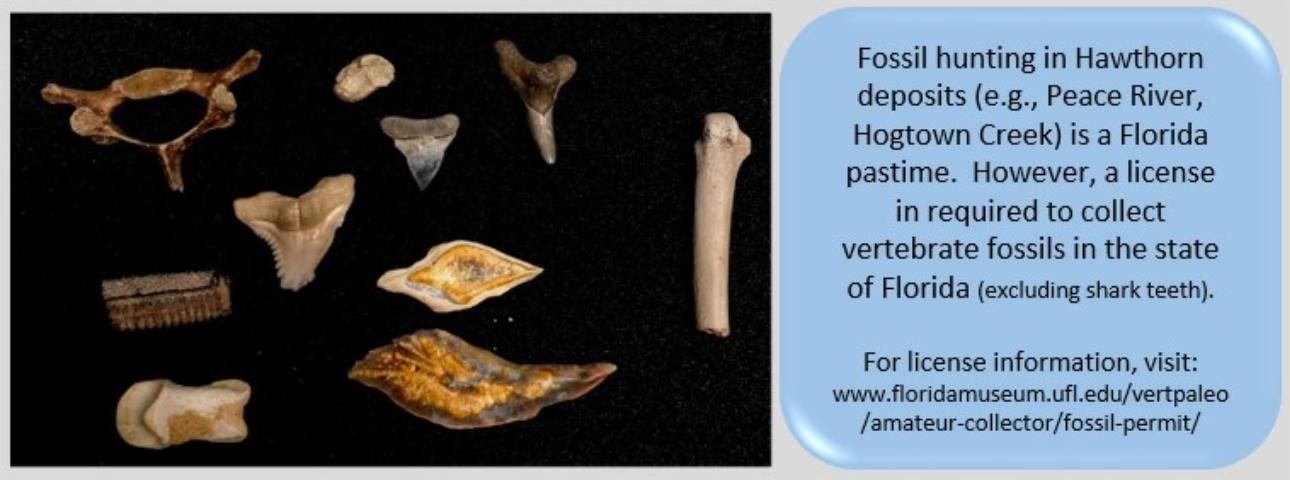
Credit: K. Bostick
The terrestrial and marine fossil assemblages from this stage reveal that Florida underwent several sea-level fluctuations during the Age of Siliciclastic Invasion. During periods of low sea-level (glacial periods), the majority of siliciclastic sediment was transported via fluvial processes (i.e., riverine transport). The siliciclastic material (dominantly clay, silt, and quartz sand) was deposited southward as deltas began overlaying the carbonate platform. These clayey sediments have low permeability, thus water cannot flow through them as it does through limestone. As a result, the Hawthorn Group forms a hydrologically impermeable confining layer, which is what holds the water in the Floridan Aquifer.
During periods of high sea-levels (interglacial periods), the majority of deposited sediment was quartz sand and phosphate. The sand was transported southward via longshore currents. We can observe this transport occurring today on north-south oriented barrier islands lining the coast of Florida. These deposits can also be observed inland as long sandy ridges (Figure 7). The elevations at these ridges can exceed 300 feet above current sea level; they are usually host to pine-dominated upland forest. The most modern of these is the Atlantic Coastal Ridge, upon which Miami is constructed.
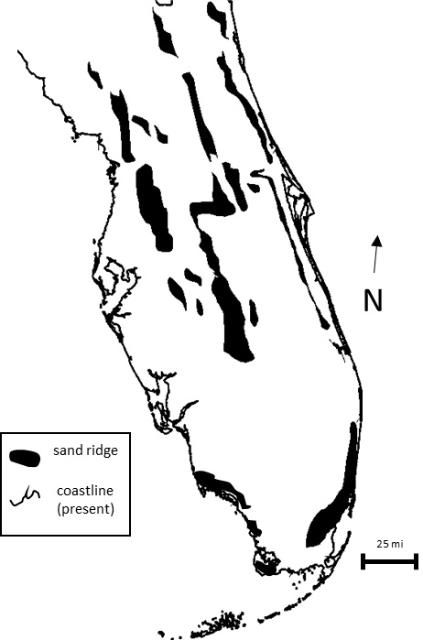
Credit: K. Bostick
In addition to its sand ridges, Florida's phosphate deposits also formed during periods of high sea level. The phosphate deposits in Florida (in the form of pebbles or nodules) are thought to form when phosphate precipitates from seawater, but phosphate deposition is highly linked to complex marine biogeochemical processes. In 1881, the Army Corps of Engineers discovered Florida's massive phosphate deposits in the Peace River, a reserve formed approximately 16 Ma ago. A large mining industry grew up around the reserve to extract Florida's phosphate for use in fertilizer. Today, 80% of the phosphate used in the United States and 25% of the phosphate used around the world comes from Florida.
The Age of Siliciclastic Invasion still occurs today. We observe both fluvial transport and longshore transport bringing siliciclastic sediments southward towards south Florida. However, southernmost Florida (e.g., the Florida Keys) is still very much in the Age of Carbonate Production, as carbonate-producing habitats (e.g., coral reefs, algal reefs) continue to form in the absence of excess siliciclastic sediments. We may expect that through the next 20 to 30 million years, southward transport of siliciclastic sediments will hinder south Florida's ability to produce carbonate sediments and rocks.
How has Florida changed?
Now that we understand how the Florida Platform has been created, we can specifically discuss the geological processes that affect Florida. There are two major processes that reform the morphology of the Florida Platform: sea-level change and karstification.
Sea-Level Change
Sea level has varied greatly over geological time scales. The predominant driver of sea level over shorter timescales is global ice volume. During glacial periods, much of the Earth's surface water is relocated onto ice sheets. During interglacial periods, ice sheets are partially or fully melted, resulting in higher sea levels. Sea level during last interglacial period (115 to 130 thousand years ago) was much higher than it is today; it is thought that sea level at peak was probably 6 to 9 m (20 to 30 feet) higher than today (Figure 8). Because the last interglacial was only about 100,000 years ago, much of the features it formed can still be observed in Florida today with relatively good resolution (e.g., above sea-level fossil coral reefs in the Florida Keys, massive fossil shell beds in southwest Florida, inland erosional scarps).
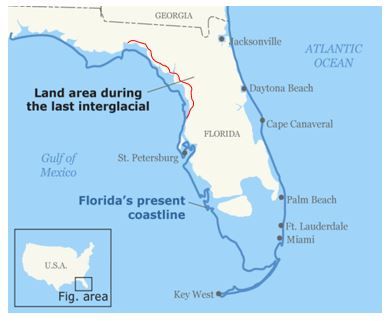
Credit: Modified from USGS (https://www.usgs.gov/media/images/shoreline-florida-usa-it-looked-during-last-interglacial-period)
Overall, Florida's geological history has been driven by changing sea levels, which have influenced the production of carbonate bedrock, siliciclastic input/transport, hydrology, and surface topography. During the last interglacial period, the majority of south Florida was a shallow carbonate environment with some siliciclastic input. Today, we can find massive, fossiliferous shell beds (115,000 years old) throughout inland southwest Florida (Daley 2002). Lake Okeechobee is hypothesized to have been a large river delta that distributed sand, silt, and clay over what would eventually become the Florida Everglades (Petuch 1987).
Margins of the northern Florida Peninsula were also flooded during the last interglacial period. Where the sea level rose thousands of years ago, you can observe today that the Hawthorn Group was eroded away, resulting in a drop in elevation around the Gulf Coastal Lowlands. This is because wave action and fluvial erosion scoured the siliciclastic sediment exposing the carbonate rocks below. The erosional face that we can observe is named the Cody Scarp (figure 8). It is a topographic break of about 100 feet in elevation difference between the Gulf Coastal Lowlands and the Northern Highlands (Katz et al. 1998).
Karstification
Karstification involves the dissolution of limestone by acid to form geologic features such as caves, sinkholes, springs, etc. As rainwater reacts with atmospheric carbon dioxide, carbonic acid (a weak acid) is formed. Furthermore, as rainwater percolates through organic-rich soils, organic acids are incorporated into groundwater, furthering the effective dissolution of limestone. The karst features of Florida's caves are formed slowly and gradually at the interface of the vadose (the unsaturated zone) and the phreatic (the saturated zone). As the water table fluctuates, dissolution can occur at various depths forming caverns. These caverns can collapse and form larger caves. Fluctuating sea levels during the most recent glaciations shifted the zone of dissolution up and down, allowing caves to form throughout the carbonate platform.
Most karst features can be seen in the Gulf Coastal Lowlands (Figure 9) where dissolution of limestone is more visually apparent at the surface. Siliciclastic overburden (i.e., siliciclastics capping the carbonate bedrock) often collapses over caverns to form sinkholes, a major geohazard for people living in certain regions of Florida. Small sinkholes are common along the Cody Scarp where the overburden accumulates thickly enough over the thin Hawthorn Group that it collapses. Sinkholes are much less common up on the Northern Highlands (i.e., hilly inland areas); this is because the Hawthorn Group and sand ridge sediment overburden is much too thick. When sinkholes do occur in the Northern Highlands, they are usually very spectacular. For example, in Alachua County, the Devil's Millhopper is a massive, 130-foot-deep, 500-foot-wide sinkhole.
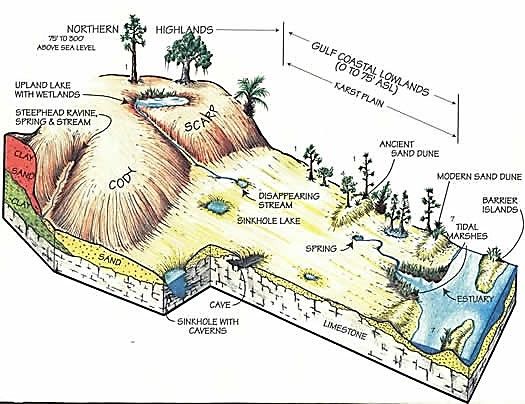
Credit: Suwannee River Hydrologic Observatory, Santa Fe Maps
Humankind and Florida Geology
Humans first reached Florida at least 12,000 years ago, and we have been living with its constantly shifting landscape ever since. Today, Florida is the third most populated state in the United States. Tourism, a major part of Florida's economy, can be attributed to the warm climate and the natural landscapes such beaches, lakes, springs, and wetlands. Humans have modified natural landscapes that developed over geologic time. Practices such as urban development, mining, coastal engineering, agriculture, water diversion and extraction, and forestry present new ways in which Florida's landscapes are formed and reworked.
When humans entered into the Florida landscape, sea level was substantially lower; the Florida Peninsula was approximately twice as large as it is today. Due to natural climate warming since the last glacial maximum (~20,000 years ago), sea level has risen to its modern position. Today, as climate warming accelerates, sea levels will continue to rise and the coast of Florida is expected to retreat inland further (Church et al. 2013).
References
Bryant, J. D., B. J. Macfadden, and P. A. Mueller. 1992. "Improved Chronological Resolution Of The Hawthorn And The Alum Bluff Groups In Northern Florida—Implications For Miocene Chronostratigraphy" Geological Society of America Bulletin, v. 104, p. 208–218. https://doi.org/10.1130/0016-7606(1992)104<0208:ICROTH>2.3.CO;2
Church, J. A., P. U. Clark, A. Cazenave, J. M. Gregory, S. Jevrejeva, A. Levermann, M. A. Merrifield, G. A. Milne, R. S. Nerem, P. D. Nunn, A. J. Payne, W. T. Pfeffer, D. Stammer, and A. S. Unnikrishnan. 2013. "Sea Level Change." In: Climate Change 2013: The Physical Science Basis. Contribution of Working Group I to the Fifth Assessment Report of the Intergovernmental Panel on Climate Change. Cambridge University Press.
Daley, G. M. 2002. "Creating a paleoecological framework for evolutionary and paleoecological studies: An example from the Fort Thompson Formation (Pleistocene) of Florida." Palaios, v. 17, p. 419–434. https://doi.org/10.1669/0883-1351(2002)017<0419:CAPFFE>2.0.CO;2
Dallmeyer, R. D., M. Caenvachette, and M. Villeneuve. 1987. "Emplacement Age Of Posttectonic Granites In Southern Guinea (West-Africa) And The Peninsular Florida Subsurface - Implications For Origins Of Southern Appalachian Exotic Terranes." Geological Society of America Bulletin v. 99, p. 87–93. https://doi.org/10.1130/0016-7606(1987)99<87:EAOPGI>2.0.CO;2
Gardulski, A. F., M. H. Gowen, A. Milsark, S. D. Weiterman, S. W. Wise, and H. T. Mullins. 1991. "Evolution Of A Deep-Water Carbonate Platform - Upper Cretaceous To Pleistocene Sedimentary Environments On The West Florida Margin" Marine Geology. v. 101, p. 163–179. https://doi.org/10.1016/0025-3227(91)90069-G
Hine, A. C. 2013. Geologic History of Florida. University Press of Florida, v. 2.
Katz, B. G., J. S. Catches, T. D. Bullen, and R. L. Michel. 1998. "Changes in the isotopic and chemical composition of ground water resulting from a recharge pulse from a sinking stream." Journal of Hydrology. v. 211, p. 178–207. https://doi.org/10.1016/S0022-1694(98)00236-4
Lane, E. 1994. Florida's Geologic History and Geologic Resources. Florida Geological Survey Special Publication, v. 35, p. 64.
Martin, J. B., and R. W. Dean. 2001. "Exchange of water between conduits and matrix in the Floridan aquifer." Chemical Geology v. 179, p. 145–165. https://doi.org/10.1016/S0009-2541(01)00320-5
Martin, J. B., and E. J. Screaton. 2001. "Exchange of Matrix and Conduit Water with Examples from the Floridan Aquifer" in Kuniansky, E.L., ed., U.S. Geological Survey Karst Interest Group: Water-Resources Investigations Report, p. 38–44.
Missimer, T. M., and R. G. Maliva. 2017. "Late Miocene fluvial sediment transport from the southern Appalachian Mountains to southern Florida: An example of an old mountain belt sediment production surge" Sedimentology v. 64, p. 1846–1870. https://doi.org/10.1111/sed.12377
Palmer, A. N. 1991. "Origin And Morphology Of Limestone Caves" Geological Society of America Bulletin, v. 103, p. 1–21. https://doi.org/10.1130/0016-7606(1991)103<0001:OAMOLC>2.3.CO;2
Petuch, E. J. 1987. "The Florida Everglades—A Buried Pseudoatoll" Journal of Coastal Research v. 3, p. 189–200.
Ryan, W.B.F., S. M. Carbotte, J. O. Coplan, S. O'Hara, A. Melkonian, R. Arko, R.A. Weissel, V. Ferrini, A. Goodwillie, F. Nitsche, J. Bonczkowski, and R. Zemsky. 2009. "Global Multi-Resolution Topography synthesis" Geochemistry Geophysics Geosystems v. 10. https://doi.org/10.1029/2008GC002332
Screaton, E., J. B. Martin, B. Ginn, and L. Smith. 2004. "Conduit properties and karstification in the unconfined Floridan Aquifer" Ground Water v. 42, p. 338–346. https://doi.org/10.1111/j.1745-6584.2004.tb02682.x
Webb, S. D., B. J. Macfadden, and J. A. Baskin. 1981. "Geology And Paleontology Of The Love Bone Bed From The Late Miocene Of Florida" American Journal of Science v. 281, p. 513–544.
White, W. B. 2002. "Karst hydrology: recent developments and open questions" Engineering Geology v. 65, p. 85–105. https://doi.org/10.1016/S0013-7952(01)00116-8
Worthington, S. R. H. 1999. "A comprehensive strategy for understanding flow in carbonate aquifers" in Palmer, A.N., M. V. Palmer, and I. D. Sasowsky, eds., Karst Modeling, Volume 5: Charles Town, West Virginia, Karst Waters Institute, p. 30–37.