Introduction
Tomato (Solanum lycopersicum L.) is a high-value, nutrient-dense vegetable crop. The fruit is a multiseeded berry containing antioxidants such as lutein and lycopene (Bhowmik et al. 2012; Castner 2004). Tomato is in high demand because of its taste and health benefits; global tomato production in 2018 was nearly 244 million tons grown on 14.3 million acres. The three countries with highest production were China (~66.8 million tons), India (~21.4 million tons), and the United States (~13.4 million tons) (FAO 2019). In Florida, tomato is the number one vegetable crop in terms of both acreage (~27,000 acres) and value (~$426 M per year) (USDA-NASS 2019). Because of its high value and wide acreage, it is important for tomato production to be efficient in its water and nutrient use, which may be improved through fertigation practices. Therefore, the objective of this article is to disseminate research-based methods of tomato production utilizing fertigation to enhance yield and nutrient use efficiency.
Tomato can be grown in greenhouses or open fields and for fresh or processing markets. A variety of tomatoes have been cultivated in Florida fields and greenhouses, including beefsteak, cluster, heirloom, grape, and small cocktail. Greenhouse tomatoes are typically indeterminate in growth habit and require regular irrigation, typically 1–2 quarts (0.95–1.89 liters) of water per day. While greenhouse tomato production offers greater control in protected conditions, allowing for uniform growth and development, greenhouse tomato production typically has greater production cost and carbon cost compared to field production (Mahajan and Singh 2006; Ntinas et al. 2017). Florida tomato cultivars should be able to set fruit in winter, have suitable disease resistance traits, and be free from cracking disorders and green shoulders (Hochmuth 2012). Planting dates for open-field Florida tomato are from July–August and February–August in north Florida, from August–September and January–February in central Florida, and from August–February in south Florida. Plants are spaced 48"–72" between rows and 18"–32" between plants. Most cultivars mature in 70–90 days (Freeman et al. 2019). When growing tomato, it is important to consider the technique of fertilization, because nutrients can be applied through a variety of techniques and fertilizer sources.
Conventionally, growers use dry granular fertilizers. Fertigation, however, is a novel method of nutrient application that involves injecting water-soluble fertilizer into irrigation, which results in improved water use efficiency, nutrient use efficiency, and yield. Fertigation is an advantageous method of nutrient application, particularly in areas where irrigation is consistently essential for crop production (Havlin et al. 2014). Because liquid fertilizer is supplied to plants through the irrigation system, fertigation allows for precise fertilizer management and reduction of nutrient application rates and costs (Burt 1995; Havlin et al. 2014). This opportunity for precise management allows for optimal absorption of water and applied nutrients that results in greater yields of crop per unit applied nutrient and water (Sureshkumar et al. 2017). Fertigation typically results in greater nutrient use efficiency because loss is mitigated by the frequent application of nutrients in small quantities (Sureshkumar et al. 2017). The benefits of fertigation on tomato production are supported by many researchers. Mahajan and Singh (2006) found that N fertigation increased yield by 59.5% in the greenhouse and 116.2% in the field compared to conventionally applied fertilizer at recommended rates. Similarly, Hebbar et al. (2003) found that water-soluble monoammonium phosphate (12-52-0), potassium nitrate (13-0-46), and urea (46-0-0) fertilizer application at the rates recommended through subsurface drip irrigation increased tomato growth and yield compared to soil-applied fertilizers with furrow irrigation (Hebbar et al. 2003).
Fertigation System Establishment
There are demonstrated environmental and economic benefits for fertigation technology. Yield enhancement results from increasing nutrient use efficiency, and the production costs are reduced via reduced labor requirements. However, there is typically a high initial investment cost for the setup of fertilizer injection systems (Sureshkumar et al. 2017; Table 1). While fertigation may be also implemented in sprinkler irrigation systems, such as center pivot, it is more common to use micro-irrigation systems for fertigation because of the application frequency, operator-controlled inputs, and better control of leaching and nutrient accumulation (Havlin et al. 2014; Sureshkumar et al. 2017). When establishing a fertigation system, it is important to consider (i) crop species and growth stage, (ii) soil characteristics, (iii) water quality, and (iv) fertilizer composition (Sureshkumar et al. 2017). Fifteen-minute flushing after each use is recommended to prevent common problems, such as precipitation, emitter clogging, or corrosion. Acidification of irrigation water may be needed if soil pH is high (Beck et al. 2002).
Because fertigation systems utilize chemicals that could backflow into chemical or water storage, causing breakage or contamination, it is required by Florida law (Florida Statute 487.055 Backflow Prevention Laws of Florida Ch. 84-338 Sec. 17) to prevent backflow by installing a vacuum breaker, low pressure drain, and check valves (Figure 1; Table 1). A vacuum relief valve allows for air to enter the pipe to prevent suction and vacuum. A low-pressure drain discharges any water that may seep past a check valve. Check valves ensure water flows in one direction and do not allow for any back-siphoning of solution to the source. Check valves can be placed on the main line to protect the water source and on the suction line to protect the chemical source. At the end of the suction line is a foot valve that functions as a check valve. The foot valve is connected to a strainer inside the chemical tank to prevent backflow and to prevent contaminants from entering the suction line (Figure 1). The suction line is connected to the injector, which is responsible for mixing the fertilizer with the irrigation water and powered by a motorized pump connected to a power source (Figure 1). Injectors may vary depending on methods but may include use of centrifugal pumps (small, radial flow booster pumps dependent on pressure in the irrigation mainline), positive-displacement pumps (in which a piston displaces solution, creating high pressure), proportional injectors (which utilize water flow and a hydraulic motor to drive a hydraulic dosing pump), pressure-differential methods (which reduce pressure at the point of injection relative to the point of intake), venturi principles (using tapered constriction that reduces pressure as water changes velocity as it passes through the constriction), or a combination of these methods. A pressure gauge can be placed on the main line to help properly maintain the system with pressure high enough to keep fertigation running, but not too high to cause damage or backflow (Figure 1). Emitters are placed at the end of the fertigation system to supply the solution to the crop and are typically drip emitters (Figure 1). Fertigation systems may last many years, depending on the parts. According to Dr. Jonathan Crane, a drip-fertigation system for papaya in Homestead, Florida, can last five years with proper maintenance. However, tomato fertigation systems tend to have a shorter life span because of the crop life cycle. Subsurface drip-irrigation systems may last 12–15 years with proper upkeep and good water quality, but thin-walled drip lines must be replaced each crop cycle (Thompson et al. 2018).
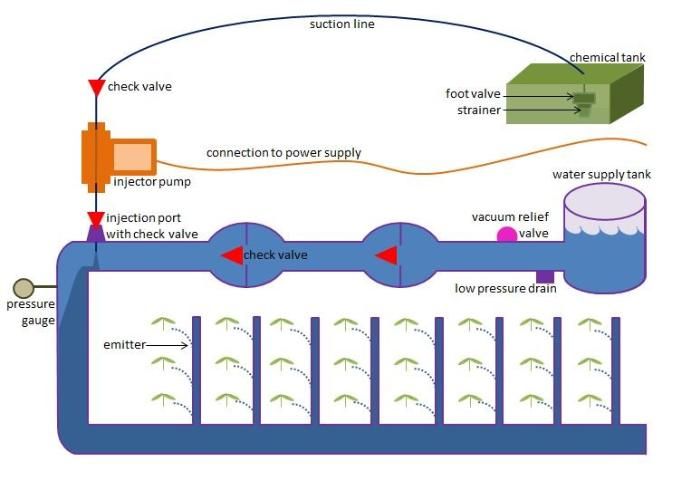
Credit: Mary Dixon and Iain Dixon
Solution Preparation
Ensuring use of high-quality water is important to avoid problems with clogging or scaling. Use of irrigation water filters are recommended to reduce debris ingress into the system (Liu and McAvoy 2012). Because fertigation supplies nutrients in aqueous forms, the fertilizer source must be water soluble and completely dissolved before injection. Therefore, because of delayed moisture absorption, use of coated granular fertilizer is not recommended (Havlin et al. 2014). These coatings that slow the dissolving rate may include organic polymers, elemental sulfur, and resin (Du et al. 2006; Lamont et al. 1987; Rindt et al. 1968). Sodium (Na)-based fertilizer can be occasionally used in fertigation systems for tomato production but should be avoided for other crops because of salinity concerns (Scaife and Bar-Yosef 1995). It is recommended to minimize other nutrients added with N and K if electrical conductivity (EC) of the irrigation water is greater than 1.4 mS/cm (Scaife and Bar-Yosef 1995).
It is recommended to use fertilizers with high purity, high solubility, low salt index, and near-neutral pH regulated to mitigate precipitation reactions (Sureshkumar et al. 2017). Tomato grows well at slightly acidic to near-neutral pH, with optimal pH values ranging from 5.5–6.8 and a target pH of 6.5 (Freeman et al. 2019). However, in water above pH 5.3, ferrous iron may oxidize to form a sparingly soluble ferric iron precipitate (Liu and McAvoy 2012). Irrigation water pH values greater than 7.5 may result in precipitation with calcium (Ca) or magnesium (Mg) and may reduce availability of phosphorus (P), iron (Fe), or zinc (Zn) (Scaife and Bar-Yosef 1995). Therefore, it is recommended to separate phosphate application spatially or temporally. Conversely, irrigation water pH values lower than 5.0 may result in aluminum (Al) or manganese (Mn) toxicity. Ammonium (NH4+) and nitrate (NO3-) may impact solution pH, with application of NH4+ resulting in pH decrease (soil acidification) (Havlin et al. 2014). Stable irrigation water pH for tomato has been shown to be maintained between a 1:4 and 1:3 NH4+-to-NO3- molar ratio (Scaife and Bar-Yosef 1995; Sureshkumar et al. 2017). Acidification of irrigation water may reduce precipitation. Hydrochloric, sulfuric, citric, and phosphoric acid may be added to the fertigation system as well as acidic fertilizers. Sulfur-based fertilizers typically lower pH values, but urea-sulfuric fertilizers are not compatible with many compounds and should therefore be avoided in fertigation systems (Liu and McAvoy 2012). Flushing fertigation systems after acid injection may prevent or minimize precipitation and corrosion (Liu and McAvoy 2012). Corrosive agents damaging to aluminum fertigation equipment include ammonium nitrate, phosphoric acid, ammonium sulfate, and copper (Beck et al. 2002). It is recommended to store phosphoric acid and other corrosives in containers made from corrosion-resistant materials, such as plastic (Papadopoulos 1996). Fertigation equipment may also be negatively impacted by corrosive halides, residual acidity, or high (bi)carbonate content from water sources (Sureshkumar et al. 2017). Therefore, testing quality of source water is an important step to determine the content of dissolved solids, sulfides, phosphates, and calcium.
Precipitate formation may clog components of a fertigation system (Havlin et al. 2014; Liu and McAvoy 2012). If the irrigation water has suspended solid content greater than 50 ppm, there is significant risk of clogging (Liu and McAvoy 2012). Preparation of a sample is recommended to examine clogging potential in which a sample of fertilizer is mixed with a sample of irrigation water at the planned dilution rate for the fertigation system. Discoloration and precipitation, if any, can then be observed (DeValerio et al. 2012). The source of water may contain nitrate, such as recycled wastewater, and microbial growth may accumulate because of continuous N application or N residue in the system after being turned off (Beck et al. 2002). Irrigation water with a high nutrient concentration may be more susceptible to bacterial growth, and water with greater than 2,600 bacterial colony-forming units (CFU) may result in clogging (Liu and McAvoy 2012). Bacterial growth may be prevented by injecting chlorine as sodium hypochlorite (NaOCl) at concentrations of 5 ppm (Liu and McAvoy 2012). Clogging can also be caused by root growth into subsurface irrigation lines or drip emitters (Liu and McAvoy 2012).
Fertigation Nutrition
The UF/IFAS fertigation recommendations for Florida field tomato production are 200 lb/acre N, 120–150 lb/A P2O5 (for low Mehlich-3 results), 100 lb/A P2O5 (for medium Mehlich-3 results), 125–150 lb/A K2O (for low Mehlich-3 results), and 100 lb/A K2O (for medium Mehlich-3 results) (Liu et al. 2019). Application of nutrients is divided over a period of many weeks (Liu et al. 2019) (Table 2).
Nitrogen is the most often applied nutrient in fertigation (Beck et al. 2002). Fertigation may result in reduced nitrate leaching because small amounts are added to the soil (Burt 1995). Urea (solid 46-0-0, solution 23-0-0), urea ammonium nitrate (UAN) (32-0-0), ammonium nitrate (20-0-0), potassium nitrate (13-0-44), and ammonium sulfate (21-0-0) are the most desirable forms of N fertigation sources because of low plugging risk. Calcium nitrate (15.5-0-0) and calcium ammonium nitrate (17-0-0) are also soluble, but they are less frequently used because of potential precipitation of phosphate ions with the Ca ions (Beck et al. 2002; Liu et al. 2019).
Phosphorus may interact with irrigation water, especially if Ca is present, so care is needed to ensure proper application of P sources. Carrijo and Hochmuth (2000) found that fertigation at rates of 22.3 lb/acre P (25 kg/ha) with 64.2 lb/acre (72 kg/ha) preplant improved Florida tomato marketable yield. Monoammonium phosphate (MAP) (8-24-0), diammonium phosphate (DAP) (8-46-0), phosphoric acid (0-54-0), urea phosphate (17-45-0), or liquid ammonium polyphosphate (10-34-0) may be used in fertigation systems. Phosphoric acid (green 0-52-0, white 0-54-0) may have acidification effects that may reduce precipitation potential. Because of the potential for phosphorus fertilizers to precipitate, it may be advantageous to separate application of phosphorus by using different lines or temporal breaks with flushing during the interim.
Potassium injection has been largely successful because of the high overall solubility of K fertilizers (Beck et al. 2002). Potassium chloride (0-0-62) and potassium nitrate (13-0-46) are the most used K-fertilizers. Potassium chloride is both the least expensive and most popular K source for fertigation. Potassium nitrate is an appropriate choice particularly for saline irrigation water sources (Beck et al. 2002). Potassium sulfate (0-0-50) and monopotassium phosphate (0-52-34) should be minimally used or avoided in fertigation systems because of the low solubility (potassium sulfate) and high precipitation risk (potassium phosphate). Potassium sulfate may be used in irrigation water sources with possible saline issues, but this fertilizer source is less soluble than potassium chloride (Beck et al. 2002).
Beck et al. (2002) gives the following recommendations:
- Tanks with Ca fertilizers should be flushed before injection, and irrigation lines should be flushed before injecting Ca fertilizer;
- Avoid application of Ca with sulfate fertilizer because of the resultant gypsum formation; however, gypsum can be used in fertigation if initially dissolved;
- Bicarbonate concentration should remain less than 150 ppm;
- Combined Ca and Mg concentrations should remain less than 50 ppm to avoid precipitation with phosphate; and
- Magnesium sulfate and sulfuric acid may be used for application of magnesium and sulfur.
Micronutrients are applied less frequently through fertigation methods because of the low demand by plants and possible low solubility in water. Metal carbonate-, oxide-, or hydroxide-incorporated zinc, manganese, copper, and iron are sparingly soluble (Beck et al. 2002). Micronutrients may be supplied in fertigation if they are in acidified hydrated forms or chelated forms. Chelation works as a means of increasing the use efficiency and effectiveness of micronutrients by surrounding a metal ion with organic ligands (Liu et al. 2012). Chelated micronutrients are less prone to oxidation and precipitation, making chelated micronutrient fertilizer sources valuable for fertigation (Liu et al. 2012). Tomato fertigated with the biodegradable chelating agent imidodisuccinic acid (IDHA, C8H11NO8) was able to grow with sufficient micronutrient supplies, particularly Zn (Lucena et al. 2008). Other chelating agents, such as diethylenetriamine pentaacetate (DTPA, C14H23N3O10) for pH lower than 7 and ethylenediaminetetraacetic acid (EDTA, C10H16N2O8) for pH lower than 6, have also been shown to successfully chelate micronutrients such as Zn, Mn, Fe, and Cu (Beck et al. 2002). For calcareous soils, ethylenediamine-N, N'-bis (1-hydroxyphenylacetic) acid (EDDHA, C18H20O6N2) is more commonly used because of its greater stability of the chelated micronutrients (Lucena et al. 2008). EDDHA-chelated Fe has been shown to increase crop yield relative to DTPA- or EDTA-chelated Fe, and chelated Fe is recommended for fertigation use compared to inorganic Fe (Liu et al. 2012).
Summary
Fertigation is an efficient method to provide crops with essential nutrients through irrigation while concomitantly reducing the risk of nutrient leaching and fertilizer runoff in tomato production. Tomato cultivation may substantially benefit from fertigation. Tomato macronutrient fertigation has shown to significantly increase yield, and micronutrient fertigation has also been successful with chelated fertilizers. When setting up a fertigation system, measures must be taken to prevent backflow to the well or chemical storage tanks. It is also important to consider the fertilizer source to prevent clogging in the system from precipitation. With proper care to the equipment and fertilization sources, fertigation may help improve BMPs and increase tomato production in Florida.
Acknowledgments
Dr. Edward Hanlon, professor emeritus at the University of Florida, reviewed and improved the manuscript.
References
Beck, H., B. Boman, S. Hall, D. Haman, J. Hebb, N. Morris, T. Obreza, M. Olexa, E. Ontermaa, L. Parsons, M. Ritenour, S. Smith, E. Stover, D. Tucker, W. Tullos, V. Vandiver Jr., M. Wade, C. Wilson, and W. Wardowski. 2002. Water and Florida Citrus: Use, Regulation, Irrigation, Systems, and Management, edited by B. Bowman. Gainesville: University of Florda Institute of Food and Agricultural Sciences. 414–427.
Bhowmik, D., K. P. Sampath Kumar, S. Paswan, S. Srivastava. 2012. "Tomato—A Natural Medicine and Its Health Benefits." Journal of Pharmacognosy and Phytochemistry 1 (1): 33–43.
Burt, C. M. 1995. "Fertigation—The Next Frontier." Irrigation Business and Technology 3 (4).
Carrijo, O. A., and G. Hochmuth. 2000. "Tomato Responses to Preplant-Incorporated or Fertigated Phosphorus on Soils Varying in Mehlich-1 Extractable Phosphorus." HortScience 35 (1): 67–72.
Castner, J. L. 2004. "Solanaceae." In Photographic Atlas of Botany and Guide to Plant Identification. Gainesville: Feline Press. 214–215.
DeValerio, J., D. Nistler, R. Hochmuth, and E. Simonne. 2012. Fertigation for Vegetables: A Practical Guide for Small Fields. HS1206. Gainesville: University of Florida Institute of Food and Agricultural Sciences. https://edis.ifas.ufl.edu/hs1206. Accessed on February 6, 2024.
Du, C-W., J-M. Zhou, and A. Shaviv. 2006. "Release Characteristics of Nutrients from Polymer-Coated Compound Controlled Release Fertilizers." Journal of Polymer Environment 14:223–230.
Food and Agriculture Organization (FAO) of the United Nations. 2019. "Tomatoes." http://www.fao.org/faostat/en/?#data/QC. Accessed on February 6, 2024.
Craig Frey, Ramdas Kanissery, Hugh A. Smith, Johan Desaeger, and Gary E. Vallad. 2023. "Chapter 18. Tomato Production." CV137. In Vegetable Production Handbook of Florida, 23rd Edition, edited by P. Dittmar, S. Agehara, N.S. Dufault. 561–646. Gainesville: University of Florida Institute of Food and Agricultural Sciences. https://edis.ifas.ufl.edu/cv137. Accessed on February 6, 2024.
Havlin, J. L., S. L. Tisdale, W. L. Nelson, and J. D. Beaton. 2014. "Nutrient Management." In Soil Fertility and Fertilizers, 8th Edition, edited by W. Lawrensen and J. Gohn. 365–426. Upper Saddle River, NJ: Pearson Inc.
Hebbar, S. S., B. K. Ramachandrappa, H. V. Nanjappa, and M. Prabhakar. 2004. "Studies on NPK Drip Fertigation in Field Grown Tomato (Lycopersicum esculentum Mill.)." European J. of Agronomy 21 (1): 117–127.
Hochmuth, G. J. 2021. Production of Greenhouse Tomatoes—Florida Greenhouse Vegetable Production Handbook, Vol 3. HS788. Gainesville: University of Florida Institute of Food and Agricultural Sciences. https://edis.ifas.ufl.edu/cv266. Accessed on February 6, 2024.
Lamont, G. P., R. J. Worrall, and M. A. O'Connell. 1987. "The Effects of Temperature and Time on the Solubility of Resin-Coated Controlled-Release Fertilizers under Laboratory and Field Conditions." Scientia Horticulturae 32 (3–4): 265–273.
Liu, G., E. Hanlon, and Y. Li. 2022. Understanding and Applying Chelated Fertilizers Effectively Based on Soil pH. HS1208. Gainesville: University of Florida Institute of Food and Agricultural Sciences. https://edis.ifas.ufl.edu/hs1208. Accessed on February 6, 2024.
Liu, G. D., E. H. Simonne, K. T. Morgan, G. J. Hochmuth, S. Agehara, and R. Mylavarapu. 2023. "Chapter 2. Fertilizer Management for Vegetable Production in Florida." CV296. In Vegetable Production Handbook of Florida, 27th Edition, edited by P. Dittmar, S. Agehara, N.S. Dufault. 5–16. Gainesville: University of Florida Institute of Food and Agricultural Sciences. https://edis.ifas.ufl.edu/cv296. Accessed on February 6, 2024.
Lucena, J. J., J. A. Sentis, M. Villen, T. Lao, and M. Perez-Saez. 2008. "IDHA Chelates as a Micronutrient Source for Green Bean and Tomato in Fertigation and Hydroponics." Agronomy Journal 100 (3): 813–818.
Mahajan, G., and K. G. Singh. 2006. "Response of Greenhouse Tomato to Irrigation and Fertigation." Agricultural Water Management 84:202–206.
Ntinas, G. K., M. Neumair, C. D. Tsadilas, and J. Meyer. 2017. "Carbon Footprint and Cumulative Energy Demand of Greenhouse and Open-Field Tomato Cultivation Systems under Southern and Central European Climatic Conditions." Journal of Cleaner Production 142 (4): 3617–3626.
Papadopoulos, I. 1996. "Micro-irrigation Systems and Fertigation." In Sustainability of Irrigated Agriculture, edited by L. S. Pereira, R. A. Feddes, J. R. Gilley, and B. Lesaffre. NATO ASI Series 312. Dordrecht, the Netherlands: Springer.
Rindt, D. W., G. M. Blouin, and J. G. Getsinger. 1968. "Sulfur Coating on Nitrogen Fertilizer to Reduce Dissolution Rate." Journal of Agricultural and Food Chemistry 16 (5): 773–778.
Scaife, A., and B. Bar-Yosef. 1995. "Nutrient and Fertilizer Management in Field Grown Vegetables." IPI Bulletin No. 13. Basel, Switzerland: International Potash Institute.
Sureshkumar, P., P. Geetha, M. C. N. Kutty, C. N. Kutty, and T. Pradeepkumar. 2017. "Fertigation—The Key Component of Precision Farming." Journal of Tropical Agriculture 54:103–114.
USDA-NASS. 2019. "2019 State Agriculture Overview: Florida." https://www.nass.usda.gov/Quick_Stats/Ag_Overview/stateOverview.php?state=FLORIDA. Accessed on February 6, 2024.
A list of parts, their purpose, specifications, and price of common components of a typical fertigation system. These components were pulled from the industrial supplier, McMaster-Carr, in 2020 (https://www.mcmaster.com/).
UF/IFAS-recommended field fertigation for soils testing low in nitrogen and potassium from the Mehlich-3 soil extraction method. Data are from Liu et al. (2019). Visit https://edis.ifas.ufl.edu/cv296 for more information.