Implementing the Five Rs of Nutrient Stewardship for Fertigation in Florida's Vegetable Production
The five Rs of nutrient stewardship is a mnemonic device used to emphasize accuracy and precision for nutrient management to apply the (1) right source of fertilizer at the (2) right rate at the (3) right time in the (4) right place with the (5) right irrigation method (Liu et al. 2019). Depending on state or region, the irrigation point may be omitted, leaving the four Rs of nutrient stewardship. Florida has many sandy soils that may result in mineral leaching, and this leaching may be impacted by irrigation scheduling (Waddell et al. 2000; Zhang et al. 2002). Therefore, this fifth R is imperative for sustainable nutrient management for commercial crop production. These main points of nutrient management (source, rate, time, place, irrigation) may help enhance sustainability by reducing pollution from nitrate leaching, nitrogen loss through ammonia volatilization, and climate change from soil greenhouse gas emission (Liu et al. 2015).
Fertigation is a method of nutrient application in which water-soluble fertilizer is injected into an irrigation system, thereby supplying plants with both nutrients and water. There are many benefits to implementing a fertigation system. If micro-irrigation is used for fertigation, the shoots and foliage are kept dry (Kafkafi 2005). The split application of fertigation helps to enhance efficiency because applications of water in smaller quantities help to reduce water percolation through the soil (Waddell et al. 2000). Fertilizer and water use efficiency have been shown to be enhanced by using fertigation, resulting in greater yield per unit fertilizer input (Burt 1995). Increases in yield and nutrient use efficiency have been reported in many fertigated vegetables, including cucumber (Cucumis sativus L.) (Singh et al. 2018), tomato (Solanum lycopersicum L.) (Ankush et al. 2018), potato (Solanum tuberosum L.) (Jia et al. 2018), maize (Zea mays L.) (Fanish and Muthukrishnan 2011), and broccoli (Brassica oleracea L.) (Priya et al. 2017). Fertigation also benefits the yield and nutrient use efficiency of woody fruit crops, including citrus (Citrus sinensis L.) (Alva et al. 2008) and coconut (Cocos nucifera L.) (Jayakumar et al. 2017). The precise and frequent application of nutrients to the root zone helps synchronize nutrient supply with the changing physiological demands as crops grow, reduces groundwater contamination by nitrates, and enhances nutrient recovery (Burt 1995; Kafkafi 2005; Li et al. 2019).
Right Source
Fertilizer source selection involves considerations of cost effectiveness and plant demand (Hochmuth et al. 2022b). Growers may also consider fertilizer form and whether an organic source should be used. While information for organic sources is not as widely available as inorganic fertigation sources, there are organic options. Fermented guano has been shown to effectively supply nitrogen (Hadas and Rosenberg 1992) and liquid fish waste digestion has been shown to supply nitrogen and potassium (Fernandez-Salvador et al. 2015). Fertilizers for use in fertigation should be liquid or water soluble. Fertigation source selection must consider pH, solubility, potential for precipitation, compatibility with other fertilizers, corrosion to the equipment, and salinity damage to crops. Water should be treated and application of additional ions avoided if the electrical conductivity (EC) is greater than 1.0 dS/cm (Scaife and Bar-Yosef 1995). Water should also have a near-neutral to slightly acidic pH to prevent mineral precipitation (Beck et al. 2002). The pH of the water may also be tailored to crop specifications outlined in Chapter 2 of the Vegetable Production Handbook of Florida (Liu et al. 2023).
Selecting the right source for fertilization is of particular importance when implementing a fertigation system. It is recommended to select solution-grade fertilizer or noncoated, water-soluble fertilizer (Beck et al. 2002). Common nitrogen (N) fertilizers used in fertigation systems include urea, urea ammonium nitrate, ammonium nitrate, ammonium thiosulfate, and calcium nitrate (Table 1, Table 6). Common potassium (K) fertilizers include potassium chloride and potassium nitrate, and a wide array of K fertilizers are appropriate for fertigation use because of their overall high solubility (Table 2). While many N and K fertilizers are soluble and fertigation-suitable, careful management is needed for phosphorus (P) sources; granular fertilizers such as superphosphate cannot be used due to low solubility, and many P fertilizers may cause clogging in lines or emitters because of precipitation with calcium (Ca) or magnesium (Mg). It is recommended to spatially or temporally separate application of P sources. To avoid plugging, it helps to use clean irrigation water with high purity and to flush the fertigation system after injection of Ca. If irrigation water sources have a high Ca concentration, it may help to acidify the solution by adding diluted sulfuric acid to reduce the pH (Obreza et al. 1993). Monoammonium phosphate (MAP), diammonium phosphate (DAP), and diluted phosphoric acid are commonly used P-fertilizers (Beck et al. 2002) (Table 3). Calcium should not be supplied with a sulfur (S) fertilizer because of resultant gypsum formation. Magnesium and sulfur can be added as magnesium sulfate (Table 4). It is important to use chelated forms for micronutrient fertigation sources because these are the most stable forms (Table 5).
It is important to reduce potential for corrosion of fertigation equipment because corrosion may dramatically reduce the years of operation (Rodrigues et al. 2020). Fertilizers, particularly ammonium nitrate, phosphoric acid, and ammonium sulfate, may cause corrosion of fertigation equipment. Ammonium nitrate results in severe corrosion of galvanized iron and considerable corrosion of phosphor-bronze when high concentration is used without appropriate flushes after each fertigation event. Phosphoric acid also causes severe corrosion of galvanized iron. Diammonium phosphate causes severe corrosion of phospho-bronze and yellow brass (Beck et al. 2002) if poorly managed. It is recommended to use stainless-steel grade 316, PVC pipe, or plastic tubing, if available, because each is mostly resistant to corrosion. Brass and bronze are susceptible to corrosion if supplied with phosphate, and copper is susceptible to corrosion by aluminum (Beck et al. 2002).
Right Rate
The first step to calculate the right rate of fertilization is to determine the current nutritional status of the soil. Because some nutrition comes from the soil, growers only need to apply nutrients in low soil concentration (Hochmuth et al. 2022b). A soil test may provide growers with a nutrient capacity index of the soil and is more helpful for analysis of low-mobility nutrients as opposed to high-mobility nutrients (Hochmuth et al. 2022a). Phosphorus is commonly analyzed by soil testing and has a low soil diffusion rate, with an average of 0.13 mm/day (Havlin et al. 2014). Conversely, N is more subject to changes with rain or irrigation, so soil testing does not occur as frequently for N analysis (Hochmuth 2022). There are many soil tests appropriate for Florida soils, including Mehlich-1 (double acid extraction), Mehlich-3 (buffered for wide ranging soil pH), AB-DTPA (for alkaline soil), and Olsen (for alkaline soil). UF/IFAS has provided recommendations based on Mehlich-1 soil tests before August 2013 and recommendations based on Mehlich-3 soil tests after August 2013. For soil-test-based nutrient recommendations for a variety of vegetable crops, please see EDIS publication CV296, Chapter 2: Fertilizer Management for Vegetable Production in Florida (Liu et al. 2023) (available at https://edis.ifas.ufl.edu/cv296). To fertilize at the right rate, it is also important to consider the crop species and cultivar, planting density, and stage of growth, because different crops may require tailored nutrition during different stages of their life.
Once nutrient recommendations have been established, dilution and injection rates must be determined to fertilize at the right rate. Because injections of total recommended fertilizer quantities in the irrigation lines would cause problems, dilution factors must be calculated to determine the exact rates of fertilizer and water supplementation to plants. First, determine the grade and target nutrient concentration of the fertigation event. Convert the fertilizer grade as a percentage into parts per million (ppm) by multiplying by 1,000,000 (Liu et al. 2021). Please note, however, that dilution factor calculations utilize elemental forms of nutrients, so phosphorus pentoxide (P2O5) needs to be multiplied by 0.4364 and potassium oxide (K2O) by 0.8302 to reach elemental P and K concentrations, respectively (Liu et al. 2023). The next step is to divide the fertilizer grade in ppm by the target concentration in ppm to reach the dilution factor (Liu et al. 2021). For example, a grower wants to supply 5 lb N per acre, according to soil test results, to tomato (Solanum lycopersicum L.) grown on a ten-acre field. This grower is going to use UAN-32 (32-0-0), a fertilizer with a density of 11.06 lb/gal, to reach a target concentration of 150 ppm N in the irrigation line. This grower's fertigation system has a flow rate of 500 gal/min. To calculate the injection rate, the following must be calculated:
- Total required N
- (Total N) = (pounds per acre N) × (number of acres)
- (Total N) = 5 lb/acre × 10 acres
- (Total N) = 50 lb N
2. Total required fertilizer
- (Total fertilizer) = (pounds of fertilizer) ÷ (fertilizer grade)
- (Total fertilizer) = 50 lb N ÷ 32% N
- (Total fertilizer) = 156.25 lb AN
3. Required fertilizer quantity in gallons
- (Fertilizer gallons) = (fertilizer pounds) ÷ (fertilizer density)
- (Fertilizer gallons) = 156.25 lb AN ÷ 11.06 lb/gal AN
- (Fertilizer gallons) = 14.13 gal AN
4. Dilution factor
- (Dilution factor) = (1,000,000 × (fertilizer grade)) ÷ (target ppm)
- (Dilution factor) = (1,000,000 × 32% N) ÷ 150 ppm N
- (Dilution factor) = 2,133.3
5. Injection rate
- (Injection rate) = (flow rate) ÷ dilution factor
- (Injection rate) = 500 gpm ÷ 2133.3
- (Injection rate) = 0.23 gpm
6. Injection time
- (Injection time) = (fertilizer gallons) ÷ (injection rate)
- (Injection time) = 14.13 gal ÷ 0.23 gpm
- (Injection time) = 61.4 min
Right Time
Nutrient application should be modified throughout the growing season to fit a crop's physiological demand. Most crops follow a growth pattern of initial slow growth rates, followed by a period of rapid growth rate, and then attenuation of growth rates preceding senescence (Taiz et al. 2015). Predicting a crop's nutrient needs is advantageous both economically and environmentally because it minimizes the extent of excess fertilization (Hochmuth et al. 2022b).
Fertigation provides a distinct advantage over traditional fertilization methods by allowing for precise management of nutrient application timing. Fertilization through fertigation does not typically occur through one major fertigation event, but instead through split applications over a period of weeks. Chapter 2 of the Vegetable Production Handbook (https://edis.ifas.ufl.edu/cv296) (Liu et al. 2023) provides guidelines for scheduling fertigation events for a variety of vegetable crops.
Right Place
Because nutrients are supplied throughout the growing season in small quantities through fertigation, nutrients are more confined to the root zone than traditional, dry granular fertilization methods (Figure 1). Nutrient uptake occurs in the area surrounding the root system. For fertigation, nutrients are applied directly with the water, which improves fertilizer placement (Beck et al. 2002). Placing the nutrients in the root zone can maximize the possibility of nutrient absorption by the plant.
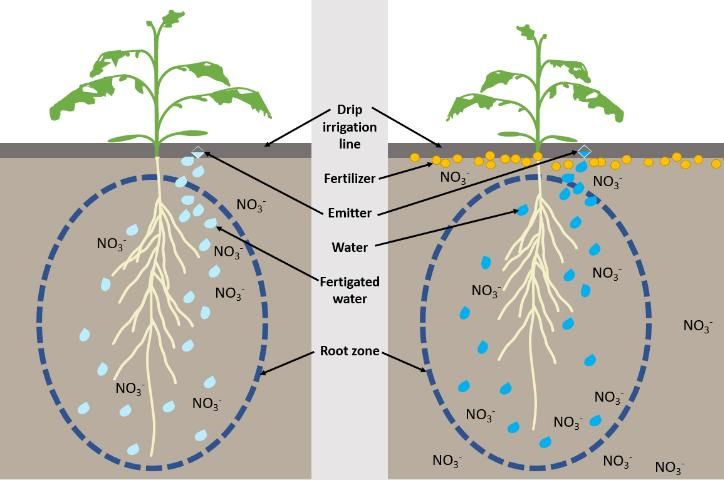
Credit: Mary Dixon, UF/IFAS
Right Irrigation
Irrigation is an important consideration for fertigation establishment. Because Florida's vegetable production occurs mostly on sandy soils, the association between fertilization and irrigation is of particular importance. Therefore, there is an emphasis on the fifth R for nutrient stewardship—right irrigation. Selecting the right irrigation includes considering irrigation schedules, systems, and water sources. Irrigation scheduling outlines the time and frequency of irrigation application. In Florida, the primary irrigation system is micro-sprinkler irrigation, but this system is not typically in operation during hot, windy, and dry days because of potential evaporation loss (Dukes et al. 2010). It is not recommended to use furrow or seepage irrigation systems because of lack of nutrient uniformity, low water use efficiency, and dependency on soil characteristics. In these irrigation systems, there is potential water and nutrient loss due to lateral flow (Beck et al. 2002). Because of wind loss, it is also not recommended to use overhead sprinkler irrigation systems. The uniformity and effectiveness of water and nutrient distribution through a sprinkler system is less than with drip irrigation methods. The best irrigation system for fertigation is micro-irrigation through micro-sprinkler or drip irrigation (Beck et al. 2002). These systems distribute water directly to the root zone, thereby optimizing water and nutrient use efficiency. Drip irrigation is preferable to micro-sprinkler irrigation because wind distortion of spray patterns may occur in micro-sprinkler systems, which impacts uniformity of water and nutrient distribution (Beck et al. 2002).
Problems with micro-irrigation methods typically include clogging of emitters, so care is needed to prevent biological, chemical, and physical clogging. Commonly precipitating ions, including Ca, Mg, Mn, and Fe, may result in chemical clogging of emitters. It is highly recommended to perform a compatibility test when establishing a fertigation system to determine if the nutrients supplied will precipitate or remain in aqueous forms. Preparing a small batch of fertilizer and irrigation water at the planned dilution ratio may help to identify any discoloration or precipitation that may occur. Another method to prevent precipitation is to regularly flush the fertigation system, especially after use of any Ca fertilizers (Beck et al. 2002). Algae and bacteria may develop on the emitters, particularly if N is continuously applied. Water chlorination is an effective way to remove bacteria in the irrigation lines (Beck et al. 2002). Using 5 ppm or lower active chlorine (not chloride) can effectively stop bacteria growth in irrigation water (Liu and McAvoy 2021). Insects, typically ants and snails, may also result in biological clogging. Fertigation system shavings, mineral particulates, or debris are common sources of physical clogging of emitters. Implementation of filtration systems may help avoid clogging by preventing ingress of undesired materials (Beck et al. 2002).
Irrigation water quality can vary widely, so an important step in ensuring system integrity is to analyze a water sample. To analyze a water sample, collect a pint of water either from a well after the pump has been operating for half an hour or from the center of a surface water body. If immediately sent to a water analysis laboratory, data can be measured for electrical conductivity (EC), total dissolved solids (TDS), suspended solid size, pH, mineral content (P, Ca, Cl, Fe, Mn), alkalinity, sulfide content, and nitrate content. These items may influence the characteristics of irrigation water. Some Florida irrigation water sources may have hard water. Acidification of the water supply is a common technique used to correct hard water. Sulfuric and phosphoric acid may be used to reduce pH and clogging concerns (Imas and Cohen 2009). High sulfide content may increase bacterial populations. High phosphate and nitrate content may result in eutrophication. Water with Mn, Mg, and Fe may result in precipitation and clogging. High chloride content may damage plants. From these data, it can be determined if water treatment is necessary before use in a fertigation system (Beck et al. 2002).
Summary
Improper or excess nutrient application is economically unsustainable and may also result in environmental damage such as eutrophication, ammonia volatilization, greenhouse gas emission from soils, or contamination of water supplies. To help reduce these adverse impacts, it helps to keep in mind the five Rs of nutrient stewardship: right source, right rate, right time, right place, and right irrigation. Ensuring that nutrient application effectively fulfills these categories may help an agroecosystem operate with greater water and nutrient use efficiency.
Acknowledgments
Dr. Edward Hanlon, professor emeritus at the University of Florida, reviewed and improved the manuscript.
References
Alva, A. K., D. Mattos Jr., and J. A. Quaggio. 2008. "Advances in Nitrogen Fertigation of Citrus." Journal of Crop Improvement 22 (1): 121–146.
Ankush, A., V. Singh, V. Kumar, and D. P. Singh. 2018. "Impact of Drip Irrigation and Fertigation Scheduling on Tomato Crop—An Overview." Journal of Applied and Natural Science 10 (1): 165–170.
Beck, H., B. Boman, S. Hall, D. Haman, J. Hebb, N. Morris, T. Obreza, M. Olexa, E. Ontermaa, L. Parsons, M. Ritenour, S. Smith, E. Stover, D. Tucker, W. Tullos, V. Vandiver Jr., M. Wade, C. Wilson, and W. Wardowski. 2002. Water and Florida Citrus: Use, Regulation, Irrigation, Systems, and Management, edited by B. Boman, 414–427. Gainesville: University of Florida Institute of Food and Agricultural Sciences.
Burt, C. M. 1995. "Fertigation—The Next Frontier." Irrigation Business and Technology 3 (4): 16–19.
Dukes, M. D., L. Zotarelli, and K. T. Morgan. 2010. "Use of Irrigation Technologies for Vegetable Crops in Florida." HortTechnology 20 (1): 133–142.
Fanish, S. A., and P. Muthukrishnan. 2011. "Effect of Drip Fertigation and Intercropping on Growth, Yield and Water Use Efficiency of Maize (Zea mays L.)." Madras Agricultural Journal 98 (7-9): 238–242.
Fernandez-Salvador, J., B. C. Strik, and D. R. Bryla. 2015. "Liquid Corn and Fish Fertilizers Are Good Options for Fertigation in Blackberry Cultivars Grown in an Organic Production System." HortScience 50 (2): 225–233.
Hadas, A., and R. Rosenberg. 1992. "Guano as a Nitrogen Source for Fertigation in Organic Farming." Fertilizer Research 31 (2): 209–214.
Havlin, J. L., S. L. Tisdale, W. L. Nelson, and J. D. Beaton. 2014. "Nutrient Management." In Soil Fertility and Fertilizers, 8th Edition, edited by W. Lawrensen and J. Gohn, 365–426. Upper Saddle River, NJ: Pearson Inc.
Hochmuth, G. 2022. Plant Petiole Sap-Testing for Vegetable Crops. CIR 1144. Gainesville: University of Florida Institute of Food and Agricultural Sciences. https://edis.ifas.ufl.edu/cv004. Accessed on February 6, 2024.
Hochmuth, G., R. Mylavarapu, and E. Hanlon. 2022a. Soil Testing for Plant-Available Nutrients—What Is It and Why Do We Use It? SL408. Gainesville: University of Florida Institute of Food and Agricultural Sciences. https://edis.ifas.ufl.edu/ss621. Accessed on February 6, 2024.
Hochmuth, G., R. Mylavarapu, and E. Hanlon. 2022b. The Four Rs of Fertilizer Management. SL411. Gainesville: University of Florida Institute of Food and Agricultural Sciences. https://edis.ifas.ufl.edu/ss624. Accessed on February 6, 2024.
Imas, P., and A. Cohen. 2009. "Improving the pH of Irrigation Waters with Acidic Fertilizers." Proceedings of the International Plant Nutrition Colloquium XVI.
Jayakumar, M., S. Janapriya, and U. Surendran. 2017. "Effect of Drip Fertigation and Polythene Mulching on Growth and Productivity of Coconut (Cocos nucifera L.), Water, Nutrient Use Efficiency and Economic Benefits." Agricultural Water Management 182:87–93.
Jia, L., Y. Qin, Y. Chen, and M. Fan. 2018. "Fertigation Improves Potato Production in Inner Mongolia (China)." Journal of Crop Improvement 32 (5): 648–656.
Kafkafi, U. 2005. "September. Global Aspects of Fertigation Usage." Fertigation Proceedings, International Symposium on Fertigation, Beijing, China. 8–22.
Li, Q., C. Xu, C. Yin, L. Kong, Y. Qin, Y. Hou, H. Wang, and L. Zhao. 2019. "Evaluation of Fertigation Technique for Phosphorus Application of Maize in the Semi-arid Region of Northeast China." Plant, Soil and Environment 65 (8): 401–407.
Liu, G., E. H. Simonne, K. T. Morgan, G. J. Hochmuth, S. Agehara, and R. Mylavarapu. 2023. Chapter 2. Fertilizer Management for Vegetable Production in Florida. CV296. Gainesville: University of Florida Institute of Food and Agricultural Sciences. https://edis.ifas.ufl.edu/cv296. Accessed on February 6, 2024.
Liu, G., J. Williamson, G. England, and A. Whidden. 2021. How to Calculate Fertigation Injection Rates for Commercial Blueberry Production. HS1197. Gainesville: University of Florida Institute of Food and Agricultural Sciences. https://edis.ifas.ufl.edu/hs1197. Accessed on February 6, 2024.
Liu, G., K. Morgan, Y. Li, L. Zotarelli, Q. Wang, and J. DeValerio. 2022. What Is 4R Nutrient Stewardship? HS1264. Gainesville: University of Florida Institute of Food and Agricultural Sciences. https://edis.ifas.ufl.edu/hs1264. Accessed on February 6, 2024.
Liu, G., Y. Li, and A. Gazula. 2023. Conversions of Parts per Million on Soil Test Reports to Pounds per Acre. HS1229. Gainesville: University of Florida Institute of Food and Agricultural Sciences. https://edis.ifas.ufl.edu/hs1229. Accessed on February 6, 2024.
Liu, G., and E. McAvoy. 2021. How to Reduce Clogging Problems in Fertigation. HS1202. Gainesville: University of Florida Institute of Food and Agricultural Sciences. https://edis.ifas.ufl.edu/hs1202. Accessed on February 6, 2024.
Obreza, T. A., M. Zekri, and D. V. Calvert. 2021. Citrus Fertilizer Management on Calcareous Soils. CIR1127. Gainesville: University of Florida Institute of Food and Agricultural Sciences. https://edis.ifas.ufl.edu/ch086. Accessed on February 6, 2024.
Priya, R. S., C. Chinnusamy, R. Karthikeyan, and R. Kuttimani. 2017. "Drip Fertigation in Vegetable Crops for Enhancing Productivity and Resource Use Efficiency: An Overview." International Journal of Current Microbiological Applied Sciences 6 (11): 3215–3230.
Rodrigues, K. V., L. A. Lima, and M. S. Thebaldi. 2020. "Effects of Fertigation on Corrosion in Galvanized Steel Used in Center Pivot Systems." Water Supply 20 (4): 1189–1194.
Scaife, A., and B. Bar-Yosef. 1995. "Nutrient and Fertilizer Management in Field Grown Vegetables." IPI Bulletin No. 13. Basel, Switzerland: International Potash Institute.
Singh, M.C., D.S. Kachwaya, and K. Kalsi. 2018. "Soilless Cucumber Cultivation under Protective Structures in Relation to Irrigation Coupled Fertigation Management, Economic Viability and Potential Benefits—A Review." International Journal of Current Microbiology and Applied Sciences 7 (3): 2451–2468.
Waddell, J. T., S. C. Gupta, J. F. Moncrief, C. J. Rosen, and D. D. Steele. 2000. "Irrigation‐ and Nitrogen‐Management Impacts on Nitrate Leaching under Potato." Journal of Environmental Quality 29 (1): 251–261.
Zhang, M. K., Z. L. He, D. V. Calvert, P. J. Stoffella, Y. C. Li, and E. M. Lamb. 2002. "Release Potential of Phosphorus in Florida Sandy Soils in Relation to Phosphorus Fractions and Adsorption Capacity." Journal of Environmental Science and Health 37 (5): 793–809.
Nitrogen fertilizer sources for fertigation. Data pulled from Beck et al. (2002) and Liu et al. (2021).
Potassium fertilizer sources for fertigation. Data pulled from Beck et al. (2002) and Liu et al. (2021).
Phosphorus fertilizer sources for fertigation. Data pulled from Beck et al. (2002) and Liu et al. (2021).
Other macronutrient fertilizer sources for fertigation. Data pulled from Beck et al. (2002), Boman and Obreza (2002), and Liu et al. (2021).